Bioinformatics analysis of therapeutic targets for idiopathic pulmonary fibrosis and exploration of immune cell infiltration patterns
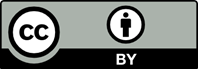
Idiopathic pulmonary fibrosis (IPF) is a severe progressive lung disease characterized by fibrotic changes in lung tissue, with limited treatment options. This study analyzed gene expression data from three gene expression omnibus datasets (GSE2052, GSE53845, and GSE110147) using R and LIMMA to identify differentially expressed genes (DEGs) in IPF samples. We identified 215 DEGs, comprising 106 upregulated and 109 downregulated genes. Weighted gene coexpression network analysis revealed five gene modules, with the module eigengene yellow showing the strongest correlation with IPF. Functional enrichment analysis of 40 consensus genes in this module indicated their significant involvement in extracellular matrix (ECM) organization. Kyoto Encyclopedia of Genes and Genomes pathway analysis revealed pathways related to protein digestion, cell adhesion molecules, and the advanced glycation end product–receptor for advanced glycation end product signaling pathway. Based on protein–protein interaction network analysis, collagen type XV alpha 1 chain (COL15A1) and collagen type VI alpha 3 chain (COL6A3) were identified as upregulated hub genes in IPF, which were regulated by microRNAs and transcription factors. Immune cell infiltration analysis showed significant changes in immune cell populations in IPF samples, with increases in memory B cells, plasma cells, and M0 macrophages and decreases in CD8 T cells, and resting natural killer cells. Potential drugs targeting COL15A1 and COL6A3 were predicted using multiple databases, revealing compounds such as (+)-JQ1, aristolochic acid I, and dexamethasone with promising binding potential. These findings suggest that COL15A1 and COL6A3 are central hub genes in IPF, are associated with ECM organization and immune response, and serve as therapeutic targets for IPF.
- Raghu G, Collard HR, Egan JJ, et al. An official ATS/ERS/ JRS/ALAT statement: Idiopathic pulmonary fibrosis: Evidence-based guidelines for diagnosis and management. Am J Respir Crit Care Med. 2011;183(6):788-824. doi: 10.1164/rccm.2009-040GL
- Raghu G, Weycker D, Edelsberg J, Bradford WZ, Oster G. Incidence and prevalence of idiopathic pulmonary fibrosis. Am J Respir Crit Care Med. 2006;174(7):810-816. doi: 10.1164/rccm.200602-163OC
- Richeldi L, Collard HR, Jones MG. Idiopathic pulmonary fibrosis. Lancet. 2017;389(10082):1941-1952. doi: 10.1016/S0140-6736(17)30866-8
- Wuyts WA, Agostini C, Antoniou KM, et al. The pathogenesis of pulmonary fibrosis: A moving target. Eur Respir J. 2013;41(5):1207-1218. doi: 10.1183/09031936.00073012
- Hwang HW, Mendell JT. MicroRNAs in cell proliferation, cell death, and tumorigenesis. Br J Cancer 2006;94(6):776-780. doi: 10.1038/sj.bjc.6603023
- O’Reilly S. MicroRNAs in fibrosis: Opportunities and challenges. Arthritis Res Ther. 2016;18:11. doi: 10.1186/s13075-016-0929-x
- Tzouvelekis A, Kaminski N. Epigenetics in idiopathic pulmonary fibrosis. Biochem Cell Biol. 2015, 93(2):159-170. doi: 10.1139/bcb-2014-0126
- Misharin AV, Morales-Nebreda L, Reyfman PA, et al. Monocyte-derived alveolar macrophages drive lung fibrosis and persist in the lung over the life span. J Exp Med. 2017;214(8):2387-2404. doi: 10.1084/jem.20162152
- Hams E, Armstrong ME, Barlow JL, et al. IL-25 and type 2 innate lymphoid cells induce pulmonary fibrosis. Proc Natl Acad Sci U S A. 2014;111(1):367-372. doi: 10.1073/pnas.1315854111
- Langfelder P, Horvath S. WGCNA: An R package for weighted correlation network analysis. BMC Bioinform. 2008;9:559. doi: 10.1186/1471-2105-9-559.
- Shannon P, Markiel A, Ozier O, et al. Cytoscape: A software environment for integrated models of biomolecular interaction networks. Genome Res. 2003;13(11):2498-2504. doi: 10.1101/gr.1239303
- Huang HY, Lin YC, Li J, et al. miRTarBase 2020: Updates to the experimentally validated microRNA-target interaction database. Nucleic Acids Res. 2020;48(D1):D148-D154. doi: 10.1093/nar/gkz896
- Yang JH, Li JH, Shao P, Zhou H, Chen YQ, Qu LH. starBase: A database for exploring microRNA-mRNA interaction maps from Argonaute CLIP-Seq and Degradome-Seq data. Nucleic Acids Res. 2011;39(Database issue):D202-D209. doi: 10.1093/nar/gkq1056
- Agarwal V, Bell GW, Nam JW, Bartel DP. Predicting effective microRNA target sites in mammalian mRNAs. Elife. 2015;4:e05005. doi: 10.7554/eLife.05005
- Chen B, Khodadoust MS, Liu CL, Newman AM, Alizadeh AA. Profiling tumor infiltrating immune cells with CIBERSORT. Methods Mol Biol. 2018;1711:243-259. doi: 10.1007/978-1-4939-7493-1_12
- Liu Y, Yang X, Gan J, Chen S, Xiao ZX, Cao Y. CB-Dock2: Improved protein-ligand blind docking by integrating cavity detection, docking and homologous template fitting. Nucleic Acids Res. 2022;50(W1):W159-W164. doi: 10.1093/nar/gkac394
- Morse C, Tabib T, Sembrat J, et al. Proliferating SPP1/ MERTK-expressing macrophages in idiopathic pulmonary fibrosis. Eur Respir J. 2019;54(2):1802441. doi: 10.1183/13993003.02441-2018
- Kumar A, Elko E, Bruno SR, et al. Inhibition of PDIA3 in club cells attenuates osteopontin production and lung fibrosis. Thorax. 2022;77(7):669-678. doi: 10.1136/thoraxjnl-2021-216882
- Rosas IO, Richards TJ, Konishi K, et al. MMP1 and MMP7 as potential peripheral blood biomarkers in idiopathic pulmonary fibrosis. PLoS Med. 2008;5(4):e93. doi: 10.1371/journal.pmed.0050093
- Zuo F, Kaminski N, Eugui E, et al. Gene expression analysis reveals matrilysin as a key regulator of pulmonary fibrosis in mice and humans. Proc Natl Acad Sci U S A. 2002;99(9):6292-6297. doi: 10.1073/pnas.092134099
- Tartaglione A, Oneto A, Bandini F, Favale E. Visual evoked potentials and pattern electroretinograms in Parkinson’s disease and control subjects. J Neurol Neurosurg Psychiatry. 1987;50(9):1243-1244. doi: 10.1136/jnnp.50.9.1243
- Cui H, Guo Z, Guo Z, et al. TMEM100 regulates neuropathic pain by reducing the expression of inflammatory factors. Mediators Inflamm. 2023;2023:9151967. doi: 10.1155/2023/9151967
- Song JJ, Hwang I, Cho KH, et al. Plasma carboxypeptidase B downregulates inflammatory responses in autoimmune arthritis. J Clin Investig. 2011;121(9):3517-3527. doi: 10.1172/JCI46387
- Ray K. CPB2 dampens inflammation in autoimmune arthritis. Nat Rev Rheumatol. 2011;7(10):558. doi: 10.1038/nrrheum.2011.135
- Lu S, Lu H, Jin R, Mo Z. Promoter methylation and H3K27 deacetylation regulate the transcription of VIPR1 in hepatocellular carcinoma. Biochem Biophys Res Commun. 2019;509(1):301-305. doi: 10.1016/j.bbrc.2018.12.129
- Tang B, Wu J, Zhu MX, et al. VPAC1 couples with TRPV4 channel to promote calcium-dependent gastric cancer progression via a novel autocrine mechanism. Oncogene. 2019;38(20):3946-3961. doi: 10.1038/s41388-019-0709-6
- Zhao L, Yu Z, Zhao B. Mechanism of VIPR1 gene regulating human lung adenocarcinoma H1299 cells. Med Oncol. 2019;36(11):91. doi: 10.1007/s12032-019-1312-y
- Heljasvaara R, Aikio M, Ruotsalainen H, Pihlajaniemi T. Collagen XVIII in tissue homeostasis and dysregulation - Lessons learned from model organisms and human patients. Matrix Biol. 2017;57-58:55-75. doi: 10.1016/j.matbio.2016.10.002
- Wang S, Zhou M, Xia Y. COL15A1 interacts with P4HB to regulate the growth and malignancy of HepG2.2.15 cells. Biochem Biophys Res Commun. 2023;681:20-28. doi: 10.1016/j.bbrc.2023.09.031
- Yu DH, Ruan XL, Huang JY, et al. Analysis of the interaction network of hub miRNAs-Hub genes, being involved in idiopathic pulmonary fibers and its emerging role in non-small cell lung cancer. Front Genet. 2020;11:302. doi: 10.3389/fgene.2020.00302
- Jin CY, Zheng R, Lin ZH, et al. Study of the collagen type VI alpha 3 (COL6A3) gene in Parkinson’s disease. BMC Neurol. 2021;21(1):187. doi: 10.1186/s12883-021-02215-7
- Chen J, Luo SF, Yuan X, et al. Diabetic kidney disease-predisposing proinflammatory and profibrotic genes identified by weighted gene co-expression network analysis (WGCNA). J Cell Biochem. 2022;123(2):481-492. doi: 10.1002/jcb.30195
- Saradna A, Do DC, Kumar S, Fu QL, Gao P. Macrophage polarization and allergic asthma. Transl Res. 2018;191:1-14. doi: 10.1016/j.trsl.2017.09.002
- Huen SC, Moeckel GW, Cantley LG. Macrophage-specific deletion of transforming growth factor-β1 does not prevent renal fibrosis after severe ischemia-reperfusion or obstructive injury. Am J Physiol Renal Physiol. 2013;305(4):F477-F484. doi: 10.1152/ajprenal.00624.2012
- Craig VJ, Zhang L, Hagood JS, Owen CA. Matrix metalloproteinases as therapeutic targets for idiopathic pulmonary fibrosis. Am J Respir Cell Mol Biol. 2015;53(5):585-600. doi: 10.1165/rcmb.2015-0020TR
- Xu Y, Lan P, Wang T. The role of immune cells in the pathogenesis of idiopathic pulmonary fibrosis. Medicina (Kaunas). 2023;59(11):1984. doi: 10.3390/medicina59111984
- Papiris SA, Kollintza A, Karatza M, et al. CD8+ T lymphocytes in bronchoalveolar lavage in idiopathic pulmonary fibrosis. J Inflamm. 2007;4:14. doi: 10.1186/1476-9255-4-14
- Brodeur TY, Robidoux TE, Weinstein JS, Craft J, Swain SL, Marshak-Rothstein A. IL-21 promotes pulmonary fibrosis through the induction of profibrotic CD8+ T cells. J Immunol. 2015;195(11):5251-5260. doi: 10.4049/jimmunol.1500777
- Xue J, Kass DJ, Bon J, et al. Plasma B lymphocyte stimulator and B cell differentiation in idiopathic pulmonary fibrosis patients. J Immunol. 2013;191(5):2089-2095. doi: 10.4049/jimmunol.1203476
- Prêle CM, Miles T, Pearce DR, et al. Plasma cell but not CD20-mediated B-cell depletion protects from bleomycin-induced lung fibrosis. Eur Respir J. 2022;60(5):2101469. doi: 10.1183/13993003.01469-2021