Hydrogen sulfide ameliorates non-alcoholic fatty liver disease in mice
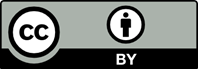
Non-alcoholic fatty liver disease (NAFLD) is a clinicopathological syndrome characterized by excessive accumulation of intrahepatic fat and elevated hepatic metabolic enzyme levels, leading to hepatocellular vesicular steatosis. The global prevalence of this liver disease has been steadily increasing, closely associated with lifestyle factors such as obesity, hypertension, and hyperlipidemia. Notably, there is currently no approved pharmaceutical treatment for NAFLD. Hydrogen sulfide (H2S) is considered a vital gaseous signaling molecule in mammalian cells, playing a crucial role in various physiological and pathological processes. Numerous recent studies have demonstrated that H2S is involved in modulating a diverse array of biological functions. In vitro and in vivo research often utilizes various readily soluble organic or inorganic compounds H2S donors, such as sodium hydrosulfide, sodium sulfide, and GYY4137, which allow for controlled release and concentration adjustment of H2S, thereby facilitating the exploration of its biological effects and underlying mechanisms. Our findings indicate that H2S holds significant potential in reducing the accumulation of cellular lipid droplets and multiple lipid deposits, as evidenced by cellular and tissue staining, as well as triglyceride and total cholesterol assays. Subsequent transcriptomic analysis revealed a potential association between the degradation of lipid droplets by H2S and the activation of cellular autophagy. Validation through Western blotting in both cellular and animal models demonstrated that H2S effectively triggers autophagy through the AMP-activated protein kinase and mechanistic target of rapamycin pathway. This activation results in enhanced degradation of intracellular lipid content and decreased lipid accumulation in hepatocytes, ultimately ameliorating NAFLD in mice.
- Chen Y, Gao WK, Shu YY, Ye J. Mechanisms of ductular reaction in non-alcoholic steatohepatitis. World J Gastroenterol. 2022;28(19):2088. doi: 10.3748/wjg.v28.i19.2088
- Guo L, Guo YY, Lib Y, et al. Enhanced acetylation of ATP-citrate lyase promotes the progression of nonalcoholic fatty liver disease. J Biol Chem. 2019;294:11805-11816. doi: 10.1074/jbc.ra119.008708
- Kashyap ML, Ganji S, Nakra NK, Kamanna VS. Niacin for treatment of nonalcoholic fatty liver disease (NAFLD): Novel use for an old drug? J Clin Lipidol. 2019;13:873-879. doi: 10.1016/j.jacl.2019.10.006
- Sheka AC, Adeyi O, Thompson J, et al. Nonalcoholic steatohepatitis: A review. JAMA. 2020;323(12):1175-1183. doi: 10.3390/ijms241914482
- Shirazi F, Wang J, Wong RJ. Nonalcoholic steatohepatitis becomes the leading indication for liver transplant registrants among US adults born between 1945 and 1965. J Clin Exp Hepatol. 2020;10:30-36. doi: 10.1016/j.jceh.2019.06.007
- Younossi ZM. Non-alcoholic fatty liver disease-a global public health perspective. J Hepatol. 2019;70:531-544. doi: 10.1016/j.jhep.2018.10.033
- Papatheodoridi AM, Chrysavgis L, Koutsilieris M, et al. The role of senescence in the development of nonalcoholic fatty liver disease and progression to nonalcoholic steatohepatitis. Hepatology. 2020;71(1):363-374. doi: 10.1002/hep.30834
- Wu WK, Zhang L, Chan MT. Autophagy, NAFLD and NAFLD-related HCC. Adv Exp Med Biol. 2018,1061:127-138. doi: 10.1007/978-981-10-8684-7_10
- Roeb E. Diagnostic and therapy of nonalcoholic fatty liver disease: A narrative review. Visc Med. 2022;38(2):126-132. doi: 10.1159/000519611
- Han CY, Rho HS, Kim A, et al. FXR inhibits endoplasmic reticulum stress-induced NLRP3 inflammasome in hepatocytes and ameliorates liver injury. Cell Rep. 2018;24(11):2985. doi: 10.1016/j.celrep.2018.07.068
- Loomba R, Friedman SL, Shulman GI. Mechanisms and disease consequences of nonalcoholic fatty liver disease. Cell. 2021;184(10):2537-2564. doi: 10.1016/j.cell.2021.04.015
- Fang YL, Chen H, Wang CL, Liang L. Pathogenesis of non-alcoholic fatty liver disease in children and adolescence: From “two hit theory” to “multiple hit model”. World J Gastroenterol. 2018;24(27):2974-2983. doi: 10.3748/wjg.v24.i27.2974
- Polyzos SA, Kountouras J, Mantzoros CS. Obesity and nonalcoholic fatty liver disease: From pathophysiology to therapeutics. Metabolism. 2019;92:82-97. doi: 10.1016/j.metabol.2018.11.014
- Zhou L, Li Q, Chen A, et al. KLF15‐activating Twist2 ameliorated hepatic steatosis by inhibiting inflammation and improving mitochondrial dysfunction via NF‐κB‐FGF21 or SREBP1c‐FGF21 pathway. FASEB J. 2019;33(12): 14254-14269. doi: 10.1096/fj.201901347rr
- Nozaki Y, Kobayashi M, Wakasawa H, et al. Systemic depletion of WWP1 improves insulin sensitivity and lowers triglyceride content in the liver of obese mice. FEBS Open Bio. 2023;13(6):1086-1094. doi: 10.1002/2211-5463.13610
- Cui X, Yao M, Feng Y, et al. Exogenous hydrogen sulfide alleviates hepatic endoplasmic reticulum stress via SIRT1/FoxO1/PCSK9 pathway in NAFLD. FASEB J. 2023;37(8):e23027. doi: 10.1096/fj.202201705rr
- Zou J, Yuan Z, Chen X, et al. Hydrogen sulfide responsive nanoplatforms: Novel gas responsive drug delivery carriers for biomedical applications. Asian J Pharm Sci. 2023;19:100858. doi: 10.1016/j.ajps.2023.100858
- Sun HJ, Wu ZY, Nie XW, Wang XY, Bian JS. Implications of hydrogen sulfide in liver pathophysiology: Mechanistic insights and therapeutic potential. J Adv Res. 2021;27:127-135. doi: 10.1016/j.jare.2020.05.010
- Wang Y, Yu R, Wu L, Yang GD. Hydrogen sulfide signaling in regulation of cell behaviors. Nitric Oxide. 2020;103:9-19. doi: 10.1016/j.niox.2020.07.002
- Ge Y, Rong F, Li W, Wang Y. On-demand therapeutic delivery of hydrogen sulfide aided by biomolecules. J Control Release. 2022;352:586-599. doi: 10.1016/j.jconrel.2022.10.055
- Abdollahi Govar A, Törő G, Szaniszlo P, et al. 3-Mercaptopyruvate sulfurtransferase supports endothelial cell angiogenesis and bioenergetics. Br J Pharmacol. 2020;177(4):866-883. doi: 10.1111/bph.14574
- Ahmad A, Druzhyna N, Szabo C. Cystathionine-gamma-lyase deficient mice are protected against the development of multiorgan failure and exhibit reduced inflammatory response during burn. Burns. 2017;43(5):1021-1033. doi: 10.1016/j.burns.2017.02.011
- Ali A, Zhang Y, Fu M, et al. Cystathionine gamma-lyase/ H2S system suppresses hepatic acetyl-CoA accumulation and nonalcoholic fatty liver disease in mice. Life Sci. 2020;252:117661. doi: 10.1016/j.lfs.2020.117661
- Bełtowski J. Synthesis, metabolism, and signaling mechanisms of hydrogen sulfide: An overview. Methods Mol Biol. 2019;2007:1-8. doi: 10.1007/978-1-4939-9528-8_1
- Liu Y, Li J, Chen H, et al. Magnet-activatable nanoliposomes as intracellular bubble microreactors to enhance drug delivery efficacy and burst cancer cells. Nanoscale. 2019;11(40):18854-18865. doi: 10.1039/c9nr07021d
- Larraufie P, Haroun K, Fleury C, Andriamihaja M, Blachier F. Regulation of enteroendocrine cell respiration by the microbial metabolite hydrogen sulfide. Front Endocrinol. 2023;14:1123364. doi: 10.3389/fendo.2023.1123364
- Brown EM, Bowden NB. Stabilities of three key biological trisulfides with implications for their roles in the release of hydrogen sulfide and bioaccumulation of sulfane sulfur. ACS Omega. 2022;7(13):11440-11451. doi: 10.1021/acsomega.2c00736
- Ubuka T. Assay methods and biological roles of labile sulfur in animal tissues. J Chromatogr B Analyt Technol Biomed Life Sci. 2002;781(1-2):227-249. doi: 10.1016/s1570-0232(02)00623-2
- Wilkie SE, Borland G, Carter RN, Morton NM, Selman C. Hydrogen sulfide in ageing, longevity and disease. Biochem J. 2021;478(19):3485-3504. doi: 10.1042/bcj20210517
- Meng GL, Wang J, Xiao YJ, et al. GYY4137 protects against myocardial ischemia and reperfusion injury by attenuating oxidative stress and apoptosis in rats. J Biomed Res. 2015;29(3):203-213. doi: 10.7555/jbr.28.20140037
- Chen JJ, Van Der Vlies AJ, Hasegawa U. Hydrogen sulfide-releasing micelles for promoting angiogenesis. Polym Chem. 2020;11(27):4454-4463. doi: 10.1039/d0py00495b
- Kumar M, Sandhir R. Hydrogen sulfide in physiological and pathological mechanisms in brain. CNS Neurol Disord Drug Targets. 2018;17(9):654-670. doi: 10.2174/1871527317666180605072018
- Gao W, Liu YF, Zhang YX, et al. The potential role of hydrogen sulfide in cancer cell apoptosis. Cell Death Discov. 2024;10(1):114. doi: 10.1038/s41420-024-01868-w
- Huang D, Jing G, Zhu S. Regulation of mitochondrial respiration by hydrogen sulfide. Antioxidants (Basel). 2023;12(8):1644. doi: 10.3390/antiox12081644
- Ngowi EE, Sarfraz M, Afzal A, et al. Roles of hydrogen sulfide donors in common kidney diseases. Front Pharmacol. 2020;11:564281. doi: 10.3389/fphar.2020.564281
- Lian CY, Zhai ZZ, Li ZF, Wang L. High fat diet-triggered non-alcoholic fatty liver disease: A review of proposed mechanisms. Chem Biol Interact. 2020;330:109199. doi: 10.1016/j.cbi.2020.109199
- Wang H, Zhu YY, Wang L, et al. Mangiferin ameliorates fatty liver via modulation of autophagy and inflammation in high-fat-diet induced mice. Biomed Pharmacother. 2017;96:328-335. doi: 10.1016/j.biopha.2017.10.022
- Van Rooyen DM, Larter CZ, Haigh WG, et al. Hepatic free cholesterol accumulates in obese, diabetic mice and causes nonalcoholic steatohepatitis. Gastroenterology. 2011;141:1393-1403, 1403.e1-5 doi: 10.1053/j.gastro.2011.06.040
- Kannt A, Madsen AN, Kammermeier C, et al. Incretin combination therapy for the treatment of non‐alcoholic steatohepatitis. Diabetes Obes Metab. 2020;22(8):1328-1338. doi: 10.1111/dom.14035
- Wu D, Zheng N, Qi K, et al. Exogenous hydrogen sulfide mitigates the fatty liver in obese mice through improving lipid metabolism and antioxidant potential. Med Gas Res. 2015;5:1. doi: 10.1186/s13618-014-0022-y
- Larter CZ, Yeh MM, Van Rooyen DM, et al. Roles of adipose restriction and metabolic factors in progression of steatosis to steatohepatitis in obese, diabetic mice. Gastroenterol Hepatol. 2009;24:1658-1668. doi: 10.1111/j.1440-1746.2009.05996.x
- Heeren J, Scheja L. Metabolic-associated fatty liver disease and lipoprotein metabolism. Mol Metab. 2021;50:101238. doi: 10.1016/j.molmet.2021.101238
- Elnegaard JJ, Iena FM, Herold J, Lebeck J. Sex‐specific effect of AQP9 deficiency on hepatic triglyceride metabolism in mice with diet‐induced obesity. J Physiol. 2023;602:3131-3149. doi: 10.1113/jp284188
- Zhang L, Zhang Z, Li C, et al. S100A11 promotes liver steatosis via FOXO1-mediated autophagy and lipogenesis. Cell Mol Gastroenterol Hepatol. 2021;11(3):697-724. doi: 10.1016/j.jcmgh.2020.10.006
- Dugbartey GJ, Alornyo KK, Adams I, et al. Targeting hepatic sulfane sulfur/hydrogen sulfide signaling pathway with α-lipoic acid to prevent diabetes-induced liver injury via upregulating hepatic CSE/3-MST expression. Diabetol Metab Syndr. 2022;14(1):148. doi: 10.1186/s13098-022-00921-x
- Matsumoto M, Hada N, Sakamaki Y, et al. An improved mouse model that rapidly develops fibrosis in non-alcoholic steatohepatitis. Int J Exp Pathol. 2013;94:93-103. doi: 10.1111/iep.12008
- Shen WJ, Azhar S, Kraemer FB. Lipid droplets and steroidogenic cells. Exp Cell Res. 2016;340(2):209-214. doi: 10.1016/j.yexcr.2015.11.024
- Byrne CD, Targher G. NAFLD: A multisystem disease. J Hepatol. 2015;62:S47-S64. doi: 10.1177/20420188221145549
- Suppli MP, Rigbolt KT, Veidal SS, et al. Hepatic transcriptome signatures in patients with varying degrees of nonalcoholic fatty liver disease compared with healthy normal-weight individuals. Am J Physiol Gastrointest Liver Physiol. 2019;316(4):G462-G472. doi: 10.1152/ajpgi.00358.2018
- Zeng J, Zhu B, Su M. Autophagy is involved in acetylshikonin ameliorating non-alcoholic steatohepatitis through AMPK/mTOR pathway. Biochem Biophys Res Commun. 2018;503(3):1645-1650. doi: 10.1016/j.bbrc.2018.07.094
- Lai SL, Mustafa MR, Wong PF. Panduratin A induces protective autophagy in melanoma via the AMPK and mTOR pathway. Phytomedicine. 2018;42:144-151. doi: 10.1016/j.phymed.2018.03.027
- Tanida I, Minematsu-Ikeguchi N, Ueno T, Kominami E. Lysosomal turnover, but not a cellular level, of endogenous LC3 is a marker for autophagy. Autophagy. 2005;1:84-91. doi: 10.4161/auto.1.2.1697