Advancing CRISPR technologies in reproductive biology
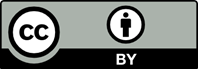
Clustered regularly interspaced short palindromic repeats (CRISPR) technology, a transformative tool for genetic modifications, gene therapy, and treating various genetic diseases, has recently garnered significant attention for its applications in reproductive biology. In this realm, researchers are focusing on addressing gynecological disorders, refining assisted reproductive methods, and conducting precise germ cell editing. The potential impact of CRISPR in these areas is monumental; however, several research gaps persist, demanding further investigation into the long-term effects, safety implications, and unintended consequences of its applications in reproductive biology. Refining the precision and specificity of CRISPR technology is a critical aspect that necessitates addressing challenges such as off-target effects and cytotoxicity. These considerations underscore the urgency for ongoing research efforts to ensure the efficacy and safety of CRISPR applications. In addition, the establishment of robust regulatory frameworks and oversight mechanisms specific to CRISPR in reproductive contexts is imperative to guarantee the responsible and ethical use of this powerful technology. This comprehensive review delves into the advantages of CRISPR over traditional gene editing methods, providing insights into its applications in embryos, pluripotent stem cells, and germline cells. It further explores the risks and limitations associated with CRISPR, including ethical concerns related to designer babies and eugenics. The article sheds light on the regulatory landscape governing new CRISPR applications in reproductive biology, emphasizing the continuous improvements in the efficiency and specificity of CRISPR technology. In contributing to the ongoing discourse, this review aims to inform and guide the responsible and effective application of CRISPR in the dynamic field of reproductive biology.
- Soh YQS, Alföldi J, Pyntikova T, et al. Sequencing the mouse Y chromosome reveals convergent gene acquisition and amplification on both sex chromosomes. Cell. 2014;159(4):800-813. doi: 10.1016/j.cell.2014.09.052
- Martin JJ, Woods DC, Tilly JL. Implications and current limitations of oogenesis from female germline or oogonial stem cells in adult mammalian ovaries. Cells. 2019;8(2):93. doi: 10.3390/cells8020093
- Bulaklak K, Gersbach CA. The once and future gene therapy. Nat Commun. 2020;11(1):5820. doi: 10.1038/s41467-020-19505-2
- Manghwar H, Lindsey K, Zhang X, Jin S. CRISPR/Cas system: Recent advances and future prospects for genome editing. Trends Plant Sci. 2019;24(12):1102-1125. doi: 10.1016/j.tplants.2019.09.006
- Gupta D, Bhattacharjee O, Mandal D, et al. CRISPR-Cas9 system: A new-fangled dawn in gene editing. Life Sci. 2019;232:116636. doi: 10.1016/j.lfs.2019.116636
- Ishino Y, Shinagawa H, Makino K, Amemura M, Nakata A. Nucleotide sequence of the iap gene, responsible for alkaline phosphatase isozyme conversion in Escherichia coli, and identification of the gene product. J Bacteriol. 1987;169(12):5429-5433. doi: 10.1128/jb.169.12.5429-5433.1987
- Ishino Y, Krupovic M, Forterre P. History of CRISPR-Cas from Encounter with a mysterious repeated sequence to genome editing technology. J Bacteriol. 2018;200(7):e00580-17. doi: 10.1128/JB.00580-17
- Frangoul H, Altshuler D, Cappellini MD, et al. CRISPR-Cas9 Gene editing for sickle cell disease and β-thalassemia. N Engl J Med. 2021;384(3):252-260. doi: 10.1056/NEJMoa2031054
- Chen M, Mao A, Xu M, Weng Q, Mao J, Ji J. CRISPR-Cas9 for cancer therapy: Opportunities and challenges. Cancer Lett. 2019;447:48-55. doi: 10.1016/j.canlet.2019.01.017
- Barrera-Paez JD, Moraes CT. Mitochondrial genome engineering coming-of-age. Trends Genet. 2022;38(8):869-880. doi: 10.1016/j.tig.2022.04.011
- Liang P, Xu Y, Zhang X, et al. CRISPR/Cas9-mediated gene editing in human tripronuclear zygotes. Protein Cell. 2015;6(5):363-372. doi: 10.1007/s13238-015-0153-5
- Kingwell K. First CRISPR therapy seeks landmark approval. Nat Rev Drug Discov. 2023;22:339-341. doi: 10.1038/d41573-023-00050-8
- Wong C. UK first to approve CRISPR treatment for diseases: What you need to know. Nature. 2023;623:676-677. doi: 10.1038/d41586-023-03590-6
- Mercuri ND, Cox BJ. The need for more research into reproductive health and disease. Elife. 2022;11:e75061. doi: 10.7554/eLife.75061
- Dunleavy JEM, Dinh DT, Filby CE, et al. Reproductive biology research down under: Highlights from the Australian and New Zealand annual meeting of the society for reproductive biology, 2021. Reprod Fertil Dev. 2022;34(13):855-866. doi: 10.1071/rd22115
- Zhou Q. Progress in modern reproductive biology research in China. Biol Reprod. 2022;107(1):3-11. doi: 10.1093/biolre/ioac122
- Shivram H, Cress BF, Knott GJ, Doudna JA. Controlling and enhancing CRISPR systems. Nat Chem Biol. 2021;17(1):10-19. doi: 10.1038/s41589-020-00700-7
- Katti A, Diaz BJ, Caragine CM, Sanjana NE, Dow LE. CRISPR in cancer biology and therapy. Nat Rev Cancer. 2022;22(5):259-279. doi: 10.1038/s41568-022-00441-w
- Gostimskaya I. CRISPR-Cas9: A history of its discovery and ethical considerations of its use in genome editing. Biochemistry (Mosc). 2022;87(8):777-788. doi: 10.1134/s0006297922080090
- Jinek M, Chylinski K, Fonfara I, Hauer M, Doudna JA, Charpentier E. A programmable dual-RNA–guided DNA endonuclease in adaptive bacterial immunity. Science. 2012;337(6096):816-821. doi: 10.1126/science.1225829
- Bhushan K, Chattopadhyay A, Pratap D. The evolution of CRISPR/Cas9 and their cousins: Hope or hype? Biotechnol Lett. 2018;40(3):465-477. doi: 10.1007/s10529-018-2506-7
- Albertini DF. Reflections in reproductive medicine 2020: Windows of opportunity lost and found. J Assist Reprod Genet. 2020;37(12):2893-2895. doi: 10.1007/s10815-020-02021-z
- Albert H. The Evolution of CRISPR Technology from Editing to Diagnostics. New York: Inside Precision Medicine; 2022.
- Alok A, Sandhya D, Jogam P, et al. The rise of the CRISPR/ Cpf1 system for efficient genome editing in plants. Front Plant Sci. 2020;11:264. doi: 10.3389/fpls.2020.00264
- Nihongaki Y, Otabe T, Ueda Y, Sato M. A split CRISPR–Cpf1 platform for inducible genome editing and gene activation. Nat Chem Biol. 2019;15(9):882-888. doi: 10.1038/s41589-019-0338-y
- Pickar-Oliver A, Gersbach CA. The next generation of CRISPR–Cas technologies and applications. Nat Rev Mol Cell Biol. 2019;20(8):490-507. doi: 10.1038/s41580-019-0131-5
- Kaminski MM, Abudayyeh OO, Gootenberg JS, Zhang F, Collins JJ. CRISPR-based diagnostics. Nat Biomed Eng. 2021;5(7):643-656. doi: 10.1038/s41551-021-00760-7
- Scheufele DA, Krause NM, Freiling I, Brossard D. What we know about effective public engagement on CRISPR and beyond. Proc Natl Acad Sci. 2021;118(22):e2004835117. doi: 10.1073/pnas.2004835117
- Liu G, Lin Q, Jin S, Gao C. The CRISPR-Cas toolbox and gene editing technologies. Mol Cell. 2022;82(2):333-347. doi: 10.1016/j.molcel.2021.12.002
- Xie J, Ge W, Li N, et al. Efficient base editing for multiple genes and loci in pigs using base editors. Nat Commun. 2019;10(1):2852. doi: 10.1038/s41467-019-10421-8
- Wang Y, Cheng H, Liu Y, et al. In-situ generation of large numbers of genetic combinations for metabolic reprogramming via CRISPR-guided base editing. Nat Commun. 2021;12(1):678. doi: 10.1038/s41467-021-21003-y
- Li Q, Li Y, Yang S, et al. CRISPR–Cas9-mediated base-editing screening in mice identifies DND1 amino acids that are critical for primordial germ cell development. Nat Cell Biol. 2018;20(11):1315-1325. doi: 10.1038/s41556-018-0202-4
- Rosello M, Serafini M, Concordet JP, Del Bene F. Precise mutagenesis in zebrafish using cytosine base editors. Nat Protoc. 2023;18(9):2794-2813. doi: 10.1038/s41596-023-00854-3
- Koonin EV, Makarova KS, Zhang F. Diversity, classification and evolution of CRISPR-Cas systems. Curr Opin Microbiol. 2017;37:67-78. doi: 10.1016/j.mib.2017.05.008
- Makarova KS, Wolf YI, Iranzo J, et al. Evolutionary classification of CRISPR–Cas systems: A burst of class 2 and derived variants. Nat Rev Microbiol. 2020;18(2):67-83. doi: 10.1038/s41579-019-0299-x
- Abbasi F, Miyata H, Ikawa M. Revolutionizing male fertility factor research in mice by using the genome editing tool CRISPR/Cas9. Reprod Med Biol. 2018;17(1):3-10. doi: 10.1002/rmb2.12067
- Hidalgo-Cantabrana C, Goh YJ, Barrangou R. Characterization and repurposing of type I and type II CRISPR–cas systems in bacteria. J Mol Biol. 2019;431(1):21-33. doi: 10.1016/j.jmb.2018.09.013
- Wang G, Li J. Review, analysis, and optimization of the CRISPR Streptococcus pyogenes Cas9 system. Med Drug Discov. 2021;9:100080. doi: 10.1016/j.medidd.2021.100080
- Khanzadi MN, Khan AA. CRISPR/Cas9: Nature’s gift to prokaryotes and an auspicious tool in genome editing. J Basic Microbiol. 2020;60(2):91-102. doi: 10.1002/jobm.201900420
- Hillary VE, Ceasar SA. A review on the mechanism and applications of CRISPR/Cas9/Cas12/Cas13/Cas14 proteins utilized for genome engineering. Mol Biotechnol. 2023;65(3):311-325. doi: 10.1007/s12033-022-00567-0
- Salanga CM, Salanga MC. Genotype to phenotype: CRISPR gene editing reveals genetic compensation as a mechanism for phenotypic disjunction of morphants and mutants. Int J Mol Sci. 2021;22(7):3472. doi: 10.3390/ijms22073472
- Tahir T, Ali Q, Rashid MH, Malik A. The journey of CRISPR-CAS9 from bacterial defense mechanism to a gene editing tool in both animals and plants. Biol Clin Sci Res J. 2020;2020:e017.
- Xue C, Greene EC. DNA repair pathway choices in CRISPR-Cas9-mediated genome editing. Trends Genet. 2021;37(7):639-656. doi: 10.1016/j.tig.2021.02.008
- Zhang H, Qin C, An C, et al. Application of the CRISPR/ Cas9-based gene editing technique in basic research, diagnosis, and therapy of cancer. Mol Cancer. 2021;20(1):126. doi: 10.1186/s12943-021-01431-6
- Ding X, Yu L, Chen L, et al. Recent progress and future prospect of CRISPR/Cas-derived transcription activation (CRISPRa) system in plants. Cells. 2022;11(19):3045. doi: 10.3390/cells11193045
- Rezanejadbardeji H, Asl BB, Amirkhah R. CRISPR as a versatile technology for gene activation and genome editing. J Genes Cells. 2018;4(1):5-9.
- Uddin F, Rudin CM, Sen T. CRISPR gene therapy: Applications, limitations, and implications for the future. Front Oncol. 2020;10:1387. doi: 10.3389/fonc.2020.01387
- Harper JC, Schatten G. Are we ready for genome editing in human embryos for clinical purposes? Eur J Med Genet. 2019;62(8):103682.
- Khan FA, Pandupuspitasari NS, ChunJie H, et al. Applications of CRISPR/Cas9 in reproductive biology. Curr Issues Mol Biol. 2018;26(1):93-102. doi: 10.21775/cimb.026.093
- Ahmed HMM, Hildebrand L, Wimmer EA. Improvement and use of CRISPR/Cas9 to engineer a sperm-marking strain for the invasive fruit pest Drosophila suzukii. BMC Biotechnol. 2019;19(1):85. doi: 10.1186/s12896-019-0588-5
- Bodke VV, Burdette JE. Advancements in microfluidic systems for the study of female reproductive biology. Endocrinology. 2021;162(10):bqab078 doi: 10.1210/endocr/bqab078
- Wu SS, Li QC, Yin CQ, Xue W, Song CQ. Advances in CRISPR/Cas-based gene therapy in human genetic diseases. Theranostics. 2020;10(10):4374. doi: 10.7150/thno.43360
- Zarei A, Razban V, Hosseini SE, Tabei SMB. Creating cell and animal models of human disease by genome editing using CRISPR/Cas9. J Gene Med. 2019;21(4):e3082. doi: 10.1002/jgm.3082
- Cinalli RM, Rangan P, Lehmann R. Germ cells are forever. Cell. 2008;132(4):559-562. doi: 10.1016/j.cell.2008.02.003
- Almeida M, Diogo R. Human enhancement: Genetic engineering and evolution. Evol Med Public Health. 2019;2019(1):183-189. doi: 10.1093/emph/eoz026
- Liang Z, Dai C, He F, et al. AKAP3 mediated type I PKA Signaling is required for mouse sperm hyperactivation and fertility. Biol Reprod. 2023:ioad180.
- Viotti M, Victor AR, Griffin DK, et al. Estimating demand for germline genome editing: An in vitro fertilization clinic perspective. CRISPR J. 2019;2(5):304-315. doi: 10.1089/crispr.2019.0044
- Sahin GN, Soyler G, Kayabolen A, Kocabay A, Taskin AC, Karahuseyinoglu S. P-567 Re-establishment of fertilization competency of the oocyte via CRISPR/dCas9 epigenome edition technology. Hum Reprod. 2021;36:deab130.566.
- Li JR, Walker S, Nie JB, Zhang XQ. Experiments that led to the first gene-edited babies: The ethical failings and the urgent need for better governance. J Zhejiang Univ Sci B. 2019;20(1):32-38. doi: 10.1631/jzus.B1800624
- Tomlinson T. A crispr future for gene-editing regulation: A proposal for an updated biotechnology regulatory system in an era of human genomic editing. Fordham Law Rev. 2018;87(1):437-483.
- Sefid F, Khadempar S, Shamriz R, Amjadi N. CRISPR gene editing on human embryos. World J Peri Neonatol. 2018;1:48-55.
- Ishii T. Germ line genome editing in clinics: The approaches, objectives and global society. Brief Funct Genomics. 2017;16(1):46-56. doi: 10.1093/bfgp/elv053
- Kumita W, Sato K, Suzuki Y, et al. Efficient generation of Knock-in/Knock-out marmoset embryo via CRISPR/Cas9 gene editing. Sci Rep. 2019;9(1):1-13. doi: 10.1038/s41598-019-49110-3
- Yoon HH, Ye S, Lim S, et al. CRISPR-Cas9 gene editing protects from the A53T-SNCA overexpression-induced pathology of Parkinson’s disease in vivo. CRISPR J. 2022;5(1):95-108. doi: 10.1089/crispr.2021.0025
- Rath A, Mishra A, Ferreira VD, et al. Functional interrogation of Lynch syndrome-associated MSH2 missense variants via CRISPR-Cas9 gene editing in human embryonic stem cells. Hum Mutat. 2019;40(11):2044-2056. doi: 10.1002/humu.23848
- Pandey VK, Tripathi A, Bhushan R, Ali A, Dubey PK. Application of CRISPR/Cas9 genome editing in genetic disorders: A systematic review up to date. J Genet Syndr Gene Ther. 2017;8(2):1-10.
- Ledford H. CRISPR gene editing in human embryos wreaks chromosomal mayhem. Nature. 2020;583(7814):17-18. doi: 10.1038/d41586-020-01906-4
- Namula Z, Wittayarat M, Do LTK, et al. Effects of the timing of electroporation during in vitro maturation on triple gene editing in porcine embryos using CRISPR/Cas9 system. Vet Anim Sci. 2022;16:100241. doi: 10.1016/j.vas.2022.100241
- Ilioi EC, Golombok S. Psychological adjustment in adolescents conceived by assisted reproduction techniques: A systematic review. Hum Reprod Update. 2015;21(1):84-96. doi: 10.1093/humupd/dmu051
- Mani S, Ghosh J, Coutifaris C, Sapienza C, Mainigi M. Epigenetic changes and assisted reproductive technologies. Epigenetics. 2020;15(1-2):12-25. doi: 10.1080/15592294.2019.1646572
- Park JE, Sasaki E. Assisted reproductive techniques and genetic manipulation in the common marmoset. ILAR J. 2020;61(2-3):286-303. doi: 10.1093/ilar/ilab002
- Novakovic B, Lewis S, Halliday J, et al. Assisted reproductive technologies are associated with limited epigenetic variation at birth that largely resolves by adulthood. Nature Communications. 2019;10(1):3922. doi: 10.1038/s41467-019-11929-9
- Ramos‐Ibeas P, Heras S, Gómez‐Redondo I, et al. Embryo responses to stress induced by assisted reproductive technologies. Mol Reprod Dev. 2019;86(10):1292-1306. doi: 10.1002/mrd.23119
- Gutási A, Hammer SE, El-Matbouli M, Saleh M. Review: Recent applications of gene editing in fish species and aquatic medicine. Animals (Basel). 2023;13(7):1250. doi: 10.3390/ani13071250
- Farquhar CM, Bhattcharya S, Repping S, et al. Female subfertility. Nat Rev Dis Primers. 2019;5:7. doi: 10.1038/s41572-018-0058-8
- Uludağ H, Aliabadi HM, Gasiunas G. Editorial: Current approaches to CRISPR/Cas9 delivery. Front Bioeng Biotechnol. 2022;10:1103007. doi: 10.3389/fbioe.2022.1103007
- Onuma A, Fujii W, Sugiura K, Naito K. Efficient mutagenesis by CRISPR/Cas system during meiotic maturation of porcine oocytes. J Reprod Dev. 2017;63(1):45-50. doi: 10.1262/jrd.2016-094
- Chen Y, Chen X, Zhang H, et al. TBC1D21 is an essential factor for sperm mitochondrial sheath assembly and male fertility. Biol Reprod. 2022;107(2):619-634. doi: 10.1093/biolre/ioac069
- Dai J, Li Q, Zhou Q, et al. IQCN disruption causes fertilization failure and male infertility due to manchette assembly defect. EMBO Mol Med. 2022;14(12):e16501. doi: 10.15252/emmm.202216501
- Wang HQ, Wang T, Gao F, Ren WZ. Application of CRISPR/ Cas technology in spermatogenesis research and male infertility treatment. Genes (Basel). 2022;13(6):1000. doi: 10.3390/genes13061000
- Klymiuk N, Seeliger F, Bohlooly YM, Blutke A, Rudmann DG, Wolf E. Tailored pig models for preclinical efficacy and safety testing of targeted therapies. Toxicol Pathol. 2016;44(3):346-357. doi: 10.1177/0192623315609688
- Kang Y, Chu C, Wang F, Niu Y. CRISPR/Cas9-mediated genome editing in nonhuman primates. Dis Models Mech. 2019;12(10):dmm039982. doi: 10.1242/dmm.039982
- Madeja Z, Pawlak P, Piliszek A. Beyond the mouse: Non-rodent animal models for study of early mammalian development and biomedical research. Int J Dev Biol. 2019;63(3-4-5):187-201. doi: 10.1387/ijdb.180414ap
- Choi CQ. CRISPR meets its match. ACS Cent Sci. 2021;7(5):699-701. doi: 10.1021/acscentsci.1c00427
- Mukherjee P, Roy S, Ghosh D, Nandi SK. Role of animal models in biomedical research: A review. Lab Anim Res. 2022;38(1):18. doi: 10.1186/s42826-022-00128-1
- Zhong L, Huang Y, He J, et al. Generation of in situ CRISPR-mediated primary and metastatic cancer from monkey liver. Signal Transduct Target Ther. 2021;6(1):411. doi: 10.1038/s41392-021-00799-7
- Wan H, Feng C, Teng F, et al. One-step generation of p53 gene biallelic mutant Cynomolgus monkey via the CRISPR/ Cas system. Cell Res. 2015;25(2):258-261. doi: 10.1038/cr.2014.158
- Bai S, Hu M, Yu L, et al. DNAJB7 is dispensable for male fertility in mice. Reprod Biol Endocrinol. 2023;21(1):32. doi: 10.1186/s12958-023-01086-6
- Wolthuis RMF, van de Vrugt HJ, Cornel MC. CRISPR gene therapy enters the clinic: the future starts now. Ned Tijdschr Geneeskd. 2021;165:D5955.
- Drakopoulou E, Anagnou NP, Pappa KI. Gene therapy for malignant and benign gynaecological disorders: A systematic review of an emerging success story. Cancers (Basel). 2022;14(13):3238. doi: 10.3390/cancers14133238
- Atabiekov I, Hobeika E, Sheikh U, El Andaloussi A, Al-Hendy A. The role of gene therapy in premature ovarian insufficiency management. Biomedicines. 2018;6(4):102. doi: 10.3390/biomedicines6040102
- Kretzmann JA, Evans CW, Moses C, et al. Tumour suppression by targeted intravenous non-viral CRISPRa using dendritic polymers. Chem Sci. 2019;10(33):7718-7727. doi: 10.1039/c9sc01432b
- Zhang W, Liu Y, Zhou X, Zhao R, Wang H. Applications of CRISPR-Cas9 in gynecological cancer research. Clin Genet. 2020;97(6):827-834. doi: 10.1111/cge.13717
- Cai J, Wu D, Jin Y, Bao S. Effect of CMB carrying PTX and CRISPR/Cas9 on endometrial cancer naked mouse model. J Healthc Eng. 2022;2022:7119195. doi: 10.1155/2022/7119195
- Wu R, Stolfi C, Zhai Y, Fearon ER, Cho KR. Abstract AP16: MODELING endometrioid and high grade serous carcinomas in the mouse using crispr/cas9-mediated somatic gene editing in fallopian tube epithelium. Clin Cancer Res. 2019;25:AP16. doi: 10.1158/1557-3265.OVCASYMP18-AP16
- Chen XZ, Guo R, Zhao C, et al. A novel anti-cancer therapy: CRISPR/Cas9 gene editing. Front Pharmacol. 2022;13:939090. doi: 10.3389/fphar.2022.939090
- Kang XJ, Caparas CIN, Soh BS, Fan Y. Addressing challenges in the clinical applications associated with CRISPR/Cas9 technology and ethical questions to prevent its misuse. Protein Cell. 2017;8(11):791-795. doi: 10.1007/s13238-017-0477-4
- Liu W, Li L, Jiang J, Wu M, Lin P. Applications and challenges of CRISPR-Cas gene-editing to disease treatment in clinics. Precis Clin Med. 2021;4(3):179-191. doi: 10.1093/pcmedi/pbab014
- Rasul MF, Hussen BM, Salihi A, et al. Strategies to overcome the main challenges of the use of CRISPR/Cas9 as a replacement for cancer therapy. Mol Cancer. 2022;21(1):64. doi: 10.1186/s12943-021-01487-4
- Li W, Huang C, Chen J. The application of CRISPR/Cas mediated gene editing in synthetic biology: Challenges and optimizations. Front Bioeng Biotechnol. 2022;10:890155. doi: 10.3389/fbioe.2022.890155
- Yang Y, Xu J, Ge S, Lai L. CRISPR/Cas: Advances, limitations, and applications for precision cancer research. Front Med. 2021;8:649896. doi: 10.3389/fmed.2021.649896
- Dohn MN. Preventing an Era of ” New Eugenics”: An argument for federal funding and regulation of gene editing research in human embryos. Richmond J Law Technol. 2018;25:1.
- Hashmi F. Necessity or vanity: Designer babies, CRISPR, and the future of genetic modifications. Int J Sci Res Manag. 2019;7(11);B-2018-35-41.
- Ranisch R. When CRISPR Meets Fantasy: Transhumanism and the Military in the Age of Gene Editing. In: Transhumanism: The Proper Guide to a Posthuman Condition or a Dangerous Idea? ; 2021. p. 111-120.
- Ayanoğlu FB, Elçin AE, Elçin YM. Bioethical issues in genome editing by CRISPR-Cas9 technology. Turk J Biol. 2020;44(2):110-120. doi: 10.3906/biy-1912-52
- Garland-Thomson R. How we got to CRISPR: The dilemma of being human. Perspect Biol Med. 2020;63(1):28-43. doi: 10.1353/pbm.2020.0002
- Actis AM. Cuestiones éticas de la edición genética mediante la tecnología CRISPR-Cas9. Revista de Bioética y Derecho. 2021;53:203-214.
- Doxzen K, Halpern J. Focusing on human rights: A framework for CRISPR germline genome editing ethics and regulation. Perspect Biol Med. 2020;63(1):44-53. doi: 10.1353/pbm.2020.0003
- DiEuliis D, Giordano J. Gene editing using CRISPR/Cas9: Implications for dual-use and biosecurity. Protein Cell. 2018;9(3):239-240. doi: 10.1007/s13238-017-0493-4
- Barrangou R, Doudna JA. Applications of CRISPR technologies in research and beyond. Nat Biotechnol. 2016;34(9):933-941. doi: 10.1038/nbt.3659
- Hammond AM, Kyrou K, Gribble M, et al. Gene-drive suppression of mosquito populations in large cages as a bridge between lab and field. Nat Commun. 2021;12: 4589. doi: 10.1038/s41467-021-24790-6.
- Godwin J, Serr M, Barnhill-Dilling SK, et al. Rodent gene drives for conservation: Opportunities and data needs. Proc R Soc B. 2019;286(1914):20191606. doi: 10.1098/rspb.2019.1606
- Brown EA, Eikenbary SR, Landis WG. Bayesian network-based risk assessment of synthetic biology: Simulating CRISPR-Cas9 gene drive dynamics in invasive rodent management. Risk Anal. 2022;42(12):2835-2846. doi: 10.1111/risa.13948
- Orr TJ, Hayssen V. The female snark is still a boojum: Looking toward the future of studying female reproductive biology. Integr Comp Biol. 2020;60(3):782-795. doi: 10.1093/icb/icaa091
- Otabe T, Nihongaki Y, Sato M. Optical control of genome editing by photoactivatable Cas9. In: Mammalian Cell Engineering: Methods and Protocols. Berlin: Springer; 2021. p. 225-233. doi: 10.1007/978-1-0716-1441-9_13
- Farris MH, Texter PA, Mora AA, et al. Detection of CRISPR-mediated genome modifications through altered methylation patterns of CpG islands. BMC Genomics. 2020;21(1):856. doi: 10.1186/s12864-020-07233-2
- Menchaca A, Dos Santos-Neto PC, Cuadro F, Souza-Neves M, Crispo M. From reproductive technologies to genome editing in small ruminants: An embryo’s journey. Anim Reprod. 2018;15(Suppl 1):984-995. doi: 10.21451/1984-3143-ar2018-0022
- Jacobi AM, Rettig GR, Turk R, et al. Simplified CRISPR tools for efficient genome editing and streamlined protocols for their delivery into mammalian cells and mouse zygotes. Methods. 2017;121-122:16-28. doi: 10.1016/j.ymeth.2017.03.021
- Yunaini L, Ari Pujianto D. Various gene modification techniques to discover molecular targets for nonhormonal male contraceptives: A review. Int J Reprod Biomed. 2023;21(1):17-32. doi: 10.18502/ijrm.v21i1.12662
- Nayyab S, Gervasi MG, Tourzani DA, et al. TSSK3, a novel target for male contraception, is required for spermiogenesis. Mol Reprod Dev. 2021;88(11):718-730. doi: 10.1002/mrd.23539
- Safari F, Farajnia S, Ghasemi Y, Zarghami N. New developments in CRISPR technology: Improvements in specificity and efficiency. Curr Pharm Biotechnol. 2017;18(13):1038-1054. doi: 10.2174/1389201019666180209120533
- Matson AW, Hosny N, Swanson ZA, Hering BJ, Burlak C. Optimizing sgRNA length to improve target specificity and efficiency for the GGTA1 gene using the CRISPR/Cas9 gene editing system. PLoS One. 2019;14(12):e0226107. doi: 10.1371/journal.pone.0226107
- Schmidt MJ, Gupta A, Bednarski C, et al. Improved CRISPR genome editing using small highly active and specific engineered RNA-guided nucleases. Nat Commun. 2021;12(1):4219. doi: 10.1038/s41467-021-24454-5
- Wei T, Cheng Q, Min YL, Olson EN, Siegwart DJ. Systemic nanoparticle delivery of CRISPR-Cas9 ribonucleoproteins for effective tissue specific genome editing. Nat Commun. 2020;11(1):3232. doi: 10.1038/s41467-020-17029-3
- Chen S, Yao Y, Zhang Y, Fan G. CRISPR system: Discovery, development and off-target detection. Cell Signal. 2020;70:109577. doi: 10.1016/j.cellsig.2020.109577
- Dong L, Guan X, Li N, et al. An anti-CRISPR protein disables type V Cas12a by acetylation. Nat Struct Mol Biol. 2019;26(4):308-314. doi: 10.1038/s41594-019-0206-1
- Marino ND, Pinilla-Redondo R, Csörgő B, Bondy-Denomy J. Anti-CRISPR protein applications: Natural brakes for CRISPR-Cas technologies. Nat Methods. 2020;17(5):471-479. doi: 10.1038/s41592-020-0771-6
- Shinmyo Y, Kawasaki H. CRISPR/Cas9-mediated gene knockout in the mouse brain using in utero electroporation. Curr Protoc Neurosci. 2017;79(1):3.32.1-3.32.11. doi: 10.1002/cpns.26
- Abbasi S, Uchida S, Toh K, et al. Co-encapsulation of Cas9 mRNA and guide RNA in polyplex micelles enables genome editing in mouse brain. J Controll Release. 2021;332:260-268. doi: 10.1016/j.jconrel.2021.02.026
- Chen K, Han H, Zhao S, et al. Lung and liver editing by lipid nanoparticle delivery of a stable CRISPR-Cas9 RNP. bioRxiv. 2023. doi: 10.1101/2023.11.15.566339
- Shen J, Lu Z, Wang J, et al. Traceable nano-biohybrid complexes by one-step synthesis as CRISPR-chem vectors for neurodegenerative diseases synergistic treatment. Adv Mater. 2021;33(27):2101993. doi: 10.1002/adma.202101993
- Lee K, Conboy M, Park HM, et al. Nanoparticle delivery of Cas9 ribonucleoprotein and donor DNA in vivo induces homology-directed DNA repair. Nat Biomed Eng. 2017;1(11):889-901. doi: 10.1038/s41551-017-0137-2
- Lee B, Lee K, Panda S, et al. Nanoparticle delivery of CRISPR into the brain rescues a mouse model of fragile X syndrome from exaggerated repetitive behaviours. Nat Biomed Eng. 2018;2(7):497-507.
- Comizzoli P, Holt WV. Breakthroughs and new horizons in reproductive biology of rare and endangered animal species. Biol Reprod. 2019;101(3):514-525. doi: 10.1093/biolre/ioz031