Cyanine-induced apoptosis for cancer therapy
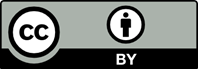
Apoptosis is a programmed cell death characterized by cell rounding, chromatin condensation and clumping, cytoplasmic collapse, and the formation of apoptotic bodies. Recently, increasing scientific research has disclosed the close relationship between apoptosis and cyanine. Cyanine, due to its excellent fluorescent characteristics, is used in constructing vital fluorescent cores for fluorescent probes. It has been extensively utilized in targeted labeling for growing cells and tumor phototherapy. Cyanine-derived compounds have provided new approaches for treating different cancers by inducing tumor cell apoptosis. This review introduces the molecular mechanism of apoptosis, essential apoptosis-related proteins, and the pathways related to tumor cell apoptosis. Furthermore, it systematically discusses the function of cyanine in cancer therapy by inducing apoptosis. A deeper understanding of cyanine-induced apoptosis may offer new options for cancer treatment and new strategies for developing and utilizing cyanine.
- Siegel RL, Miller KD, Jemal A, 2019, Cancer statistics, 2019. CA Cancer J Clin, 69(1): 7–34. https://doi.org/10.3322/caac.21551
- Sung H, Siegel RL, Torre LA, et al., 2019, Global Patterns in excess body weight and the associated cancer burden. CA Cancer J Clin, 69: 88–112. https://doi.org/10.3322/caac.21499
- Das S, Shukla N, Singh SS, et al., 2021, Mechanism of Interaction between autophagy and apoptosis in cancer. Apoptosis, 26: 512–533. https://doi.org/10.1007/s10495-021-01687-9
- Liu JJ, Lin M, Yu JY, et al., 2011, Targeting apoptotic and autophagic pathways for cancer therapeutics. Cancer Lett, 300: 105–114. https://doi.org/10.1016/j.canlet.2010.10.001
- Chaudhry GE, Md Akim A, Sung YY, et al., 2022, Cancer and apoptosis: The apoptotic activity of plant and marine natural products and their potential as targeted cancer therapeutics. Front Pharmacol, 13: 842376. https://doi.org/10.3389/fphar.2022.842376
- Yuan L, Cai Y, Zhang L, et al., 2021, Promoting apoptosis, a promising way to treat breast cancer with natural products: A comprehensive review. Front Pharmacol, 12: 801662. https://doi.org/10.3389/fphar.2021.801662
- Goldblatt ZE, Cirka HA, Billiar KL, 2021, Mechanical regulation of apoptosis in the cardiovascular system. Ann Biomed Eng, 49: 75–97. https://doi.org/10.1007/s10439-020-02659-x
- Ketelut-Carneiro N, Fitzgerald KA, 2022, Apoptosis, pyroptosis, and necroptosis-oh my! The many ways a cell can die. J Mol Biol, 434: 167378. https://doi.org/10.1016/j.jmb.2021.167378
- Pistritto G, Trisciuoglio D, Ceci C, et al., 2016, Apoptosis as anticancer mechanism: Function and dysfunction of its modulators and targeted therapeutic strategies. Aging (Albany NY), 8: 603–619. https://doi.org/10.18632/aging.100934
- D’Arcy MS, 2019, Cell death: A review of the major forms of apoptosis, necrosis and autophagy. Cell Biol Int, 43: 582–592. https://doi.org/10.1002/cbin.11137
- Tao SC, Yuan T, Rui BY, et al., 2017, Exosomes derived from human platelet-rich plasma prevent apoptosis induced by glucocorticoid-associated endoplasmic reticulum stress in rat osteonecrosis of the femoral head via the Akt/Bad/Bcl-2 signal pathway. Theranostics, 7: 733–750. https://doi.org/10.7150/thno.17450
- Sun L, Wang H, Wang Z, et al., 2012, Mixed lineage kinase domain-like protein mediates necrosis signaling downstream of Rip3 kinase. Cell, 148: 213–227. https://doi.org/10.1016/j.cell.2011.11.031
- Li X, Gong W, Wang H, et al., 2019, O-GlcNAc transferase suppresses inflammation and necroptosis by targeting receptor-interacting serine/threonine-protein kinase 3. Immunity, 50: 576–590.e6. https://doi.org/10.1016/j.immuni.2019.01.007
- Santagostino SF, Assenmacher CA, Tarrant JC, et al., 2021, Mechanisms of regulated cell death: Current perspectives. Vet Pathol, 58: 596–623. https://doi.org/10.1177/03009858211005537
- Proneth B, Conrad M, 2019, Ferroptosis and necroinflammation, a yet poorly explored link. Cell Death Differ, 26: 14–24. https://doi.org/10.1038/s41418-018-0173-9
- Conrad M, Angeli JPF, Vandenabeele P, et al., 2016, Regulated necrosis: Disease relevance and therapeutic opportunities. Nat Rev Drug Discov, 15: 348–366. https://doi.org/10.1038/nrd.2015.6
- Li J, Cao, F, Yin HL, et al., 2020, Ferroptosis: Past, present and future. Cell Death Dis, 11: 88. https://doi.org/10.1038/s41419-020-2298-2
- Yang F, He Y, Zhai Z, et al., 2019, Programmed cell death pathways in the pathogenesis of systemic lupus erythematosus. J Immunol Res, 2019: 3638562. https://doi.org/10.1155/2019/3638562
- Cai J, Yi M, Tan Y, et al., 2021, Natural product triptolide induces GSDME-mediated pyroptosis in head and neck cancer through suppressing mitochondrial hexokinase-ΙΙ. J Exp Clin Cancer Res, 40: 190. https://doi.org/10.1186/s13046-021-01995-7
- Yu J, Li S, Qi J, et al., 2019, Cleavage of GSDME by caspase-3 determines lobaplatin-induced pyroptosis in colon cancer cells. Cell Death Dis, 10: 193. https://doi.org/10.1038/s41419-019-1441-4
- Wu J, Lin S, Wan B, et al., 2019, Pyroptosis in liver disease: New insights into disease mechanisms. Aging Dis, 10: 1094–1108. https://doi.org/10.14336/AD.2019.0116
- Roth KG, Mambetsariev I, Kulkarni P, et al., 2020, The mitochondrion as an emerging therapeutic target in cancer. Trends Mol Med, 26: 119–134. https://doi.org/10.1016/j.molmed.2019.06.009
- Zaib S, Hayyat A, Ali N, et al., 2022, Role of mitochondrial membrane potential and lactate dehydrogenase a in apoptosis. Anticancer Agents Med Chem, 22: 2048–2062. https://doi.org/10.2174/1871520621666211126090906
- Malcom J, Arnold O, Howlett JG, et al., 2008, Canadian cardiovascular society consensus conference guidelines on heart failure--2008 update: Best practices for the transition of care of heart failure patients, and the recognition, investigation and treatment of cardiomyopathies. Can J Cardiol, 24: 21–40. https://doi.org/10.1016/s0828-282x(08)70545-2
- Shaddy RE, Olsen SL, Bristow MR, et al., 1995, Efficacy and safety of metoprolol in the treatment of doxorubicin-induced cardiomyopathy in pediatric patients. Am Heart J, 129: 197–199. https://doi.org/10.1016/0002-8703(95)90061-6
- Hu Y, Shao Z, Cai X, et al., 2019, Mitochondrial pathway is involved in advanced glycation end products-induced apoptosis of rabbit annulus fibrosus cells. Spine (Phila Pa 1976), 44: E585–E595. https://doi.org/10.1097/BRS.0000000000002930
- Carneiro BA, El-Deiry WS, 2020, Targeting apoptosis in cancer therapy. Nat Rev Clin Oncol, 17: 395–417. https://doi.org/10.1038/s41571-020-0341-y
- Boraldi F, Lofaro FD, Quaglino D, 2021, Apoptosis in the extraosseous calcification process. Cells. 10: 131. https://doi.org/10.3390/cells10010131
- Khodavirdipour A, Piri M, Jabbari S, et al., 2021, Apoptosis detection methods in diagnosis of cancer and their potential role in treatment: Advantages and disadvantages: A review. J gastrointest Cancer, 52: 422–430. https://doi.org/10.1007/s12029-020-00576-9
- Wlodkowic D, Telford W, Skommer J, et al., 2011, Apoptosis and beyond: Cytometry in Studies of programmed cell death. Methods Cell Biol, 103: 55–98. https://doi.org/10.1016/B978-0-12-385493-3.00004-8
- Cross CE, Halliwell B, Borish ET, et al., 1987, Oxygen radicals and human disease. Ann Intern Med, 107: 526–545. https://doi.org/10.7326/0003-4819-107-4-526
- Liou GY, Storz P, 2010, Reactive oxygen species in cancer. Free Radic Res, 44: 479–496. https://doi.org/10.3109/10715761003667554
- Wenner CE, Spirtes MA, Weinhouse S, 1952, Metabolism of neoplastic tissue. II. A survey of enzymes of the citric acid cycle in transplanted tumors. Cancer Res, 12: 44–49.
- Tsuchiya K, 2021, Switching from apoptosis to pyroptosis: Gasdermin-elicited inflammation and antitumor immunity. Int J Mol Sci, 22: 426. https://doi.org/10.3390/ijms22010426
- Hilska M, Collan YU, Laine VJ, et al., 2005, The significance of tumor markers for proliferation and apoptosis in predicting survival in colorectal cancer. Dis Colon Rectum, 48: 2197–2208. https://doi.org/10.1007/s10350-005-0202-x
- Aaltomaa S, Kärjä V, Lipponen P, et al., 2006, Expression of Ki-67, Cyclin D1 and apoptosis markers correlated with survival in prostate cancer patients treated by radical prostatectomy. Anticancer Res, 26: 4873–4878.
- Wójcik P, Žarković N, Gęgotek A, et al., 2020, Involvement of metabolic lipid mediators in the regulation of apoptosis. Biomolecules, 10: 402. https://doi.org/10.3390/biom10030402
- Dadsena S, Zollo C, García-Sáez AJ, 2021, Mechanisms of mitochondrial cell death. Biochem Soc Trans, 49: 663–674. https://doi.org/10.1042/BST20200522
- Tian C, Liu Y, Li Z, et al., 2022, Mitochondria related cell death modalities and disease. Front Cell Dev Biol, 10: 832356. https://doi.org/10.3389/fcell.2022.832356
- Frezza C, Cipolat S, Martins de Brito O, et al., 2006, OPA1 controls apoptotic cristae remodeling independently from mitochondrial fusion. Cell, 126: 177–189. https://doi.org/10.1016/j.cell.2006.06.025
- Gupta S, Kass GE, Szegezdi E, et al., 2009, The mitochondrial death pathway: A promising therapeutic target in diseases. J Cell Mol Med, 13: 1004–1033. https://doi.org/10.1111/j.1582-4934.2009.00697.x
- Calvo SE, Mootha VK, 2010, The mitochondrial proteome and human disease. Ann Rev Genom Hum Genet, 11: 25–44. https://doi.org/10.1146/annurev-genom-082509-141720
- Nunnari J, Suomalainen A, 2012, Mitochondria: In sickness and in health. Cell, 148: 1145–1159. https://doi.org/10.1016/j.cell.2012.02.035
- Gogvadze V, Orrenius S, Zhivotovsky B, 2008, Mitochondria in cancer cells: What Is so special about them? Trends Cell Biol, 18: 165–173. https://doi.org/10.1016/j.tcb.2008.01.006
- Boroughs LK, DeBerardinis RJ, 2015, Metabolic pathways promoting cancer cell survival and growth. Nat Cell Biol, 17: 351–359. https://doi.org/10.1038/ncb3124
- Patil S, Ghosh D, Radhakrishna M, et al., 2020, Mitochondrial impairment by cyanine-based small molecules induces apoptosis in cancer cells. ACS Med Chem Lett, 11: 23–28. https://doi.org/10.1021/acsmedchemlett.9b00304
- Zhang L, Jia H, Liu X, et al., 2021, Heptamethine cyanine-based application for cancer theranostics. Front Pharmacol, 12: 764654. https://doi.org/10.3389/fphar.2021.764654
- Wu XY, Xu WW, Huan XK, et al., 2023, Najafi mechanisms of cancer cell killing by metformin: A review on different cell death pathways. Mol Cell Biochem, 478: 197–214. https://doi.org/10.1007/s11010-022-04502-4
- Tait SW, Green DR, 2010, Mitochondria and cell death: Outer membrane permeabilization and beyond. Nat Rev Mol Cell Biol, 11: 621–632. https://doi.org/10.1038/nrm2952
- Zhang Q, Liu J, Zhang M, et al., 2019, Apoptosis induction of fibroblast-like synoviocytes is an important molecular-mechanism for herbal medicine along with its active components in treating rheumatoid arthritis. Biomolecules, 9: 795. https://doi.org/10.3390/biom9120795
- Gong YN, Crawford JC, Heckmann BL, et al., 2019, To the edge of cell death and back. FEBS J, 286: 430–440. https://doi.org/10.1111/febs.14714
- Cain K, Brown DG, Langlais C, et al., 1999, Caspase activation involves the formation of the aposome, a large (approximately 700 Kda) caspase-activating complex. J Biol Chem, 274: 22686–22692. https://doi.org/10.1074/jbc.274.32.22686
- Srinivasula SM, Ahmad M, Fernandes-Alnemri T, et al., 1998, Autoactivation of procaspase-9 by Apaf-1-mediated oligomerization. Mol Cell, 1: 949–957. https://doi.org/10.1016/s1097-2765(00)80095-7
- Li P, Nijhawan D, Budihardjo I, et al., 1997, Cytochrome C and Datp-dependent formation of Apaf-1/Caspase-9 complex initiates an apoptotic protease cascade. Cell, 91: 479–489. https://doi.org/10.1016/s0092-8674(00)80434-1
- Zou H, Henzel WJ, Liu X, et al., 1997, Apaf-1, a human protein homologous to C. Elegans Ced-4, participates in cytochrome c-dependent activation of caspase-3. Cell, 90: 405–413. https://doi.org/10.1016/s0092-8674(00)80501-2
- Acehan D, Jiang X, Morgan DG, et al., 2002, Three-Dimensional structure of the apoptosome: Implications for assembly, procaspase-9 binding, and activation. Mol Cell, 9: 423–432. https://doi.org/10.1016/s1097-2765(02)00442-2
- Kalkavan H, Green DR, 2018, Cell suicide as a Bcl-2 family business. Cell Death Differ, 25: 46–55. https://doi.org/10.1038/cdd.2017.179
- Ledgerwood EC, Morison IM, 2009, Targeting the apoptosome for cancer therapy. Clin Cancer Res, 15: 420–424. https://doi.org/10.1158/1078-0432.CCR-08-1172
- Liu X, Kim CN, Yang J, et al., 1996, Induction of apoptotic program in cell-free extracts: Requirement for dATP and cytochrome C. Cell, 86: 147–157. https://doi.org/10.1016/s0092-8674(00)80085-9
- Bhola PD, Letai A, 2016, Mitochondria-judges and executioners of cell death sentences. Mol Cell, 61: 695–704. https://doi.org/10.1016/j.molcel.2016.02.019
- Jiang L, Zhang X, Zheng X, et al., 2013, Apoptosis, senescence, and autophagy in rat nucleus pulposus cells: Implications for diabetic intervertebral disc degeneration. J Orthop Res, 31: 692–702. https://doi.org/10.1002/jor.22289
- Zhang F, Zhao X, Shen H, et al., 2016, Molecular Mechanisms of cell death in intervertebral disc degeneration (review). Int J Mol Med, 37: 1439–1448. https://doi.org/10.3892/ijmm.2016.2573
- Green DR, Reed JC, 1998, Mitochondria and apoptosis. Science (New York, NY), 281: 1309–1312. https://doi.org/10.1126/science.281.5381.1309
- Kayacan S, Sener LT, Melikoglu G, et al., 2018, Induction of apoptosis by Centaurea nerimaniae extract in HeLa and MDA-MB-231 cells by a caspase-3 pathway. Biotech Histochem, 93: 311–319. https://doi.org/10.1080/10520295.2017.1401662
- Nagata S, 2018, Apoptosis and clearance of apoptotic cells. Annu Rev Immunol, 36: 489–517. https://doi.org/10.1146/annurev-immunol-042617-053010
- Suzuki J, Denning DP, Imanishi E, et al., 2013, Xk-related protein 8 and CED-8 promote phosphatidylserine exposure in apoptotic cells. Science (New York, NY), 341: 403–406. https://doi.org/10.1126/science.1236758
- Segawa K, Kurata S, Yanagihashi Y, et al., 2014, Caspase- Mediated cleavage of phospholipid flippase for apoptotic phosphatidylserine exposure. Science (New York, NY) 344: 1164–1168. https://doi.org/10.1126/science.1252809
- Suzuki J, Umeda M, Sims PJ, et al., 2010, Calcium-dependent phospholipid scrambling by TMEM16F. Nature, 468: 834–838. https://doi.org/10.1038/nature09583
- Slee EA, Harte MT, Kluck RM, et al., 1999, Ordering the cytochrome C-initiated caspase cascade: Hierarchical activation of caspases-2, -3, -6, -7, -8, and -10 in a caspase-9- dependent manner. J Cell Biol, 144: 281–292. https://doi.org/10.1083/jcb.144.2.281
- Nicholson DW, Thornberry NA, 1997, Caspases: Killer proteases. Trends Biochem Sci, 22: 299–306. https://doi.org/10.1016/s0968-0004(97)01085-2
- Fraser A, Evan G, 1996, A license to kill. Cell, 85: 781–784. https://doi.org/10.1016/s0092-8674(00)81005-3
- Van de Craen M, Declercq W, Van den Brande I, et al., 1999, The proteolytic procaspase activation network: An in vitro analysis. Cell Death Differ, 6: 1117–1124. https://doi.org/10.1038/sj.cdd.4400589
- Chandler JM, Cohen GM, MacFarlane M, 1998, Different subcellular distribution of caspase-3 and caspase-7 following FAS-induced apoptosis in mouse liver. J Biol Chem, 273: 10815–10818. https://doi.org/10.1074/jbc.273.18.10815
- Fan TJ, Han LH, Cong RS, et al., 2005, Caspase family proteases and apoptosis. Acta Biochim Biophys Sin (Shanghai), 37: 719–727. https://doi.org/10.1111/j.1745-7270.2005.00108.x
- Wong RS, 2011, Apoptosis in cancer: From pathogenesis to treatment. J Exp Clin Cancer Res, 30: 87. https://doi.org/10.1186/1756-9966-30-87
- Kale J, Osterlund EJ, Andrews DW, 2018, Bcl-2 family proteins: Changing partners in the dance towards death. Cell Death Differ,25: 65–80. https://doi.org/10.1038/cdd.2017.186
- Yang R, Dong H, Jia S, 2022, Resveratrol as a modulatory of apoptosis and autophagy in cancer therapy. Clin Transl Oncol, 24: 1219–1230. https://doi.org/10.1007/s12094-021-02770-y
- Siddiqui WA, Ahad A, Ahsan H, 2015, The mystery of Bcl2 family: Bcl-2 proteins and apoptosis: An update. Arch Toxicol, 89: 289–317. https://doi.org/10.1007/s00204-014-1448-7
- Harada H, Grant S, 2003, Apoptosis regulators. Rev Clin Exp Hematol, 7: 117–138.
- Wang K, Yin XM, Chao DT, et al., 1996, Bid: A novel Bh3 domain-only death agonist. Genes Dev, 10: 2859–2869. https://doi.org/10.1101/gad.10.22.2859
- O’Connor L, Strasser A, O’Reilly LA, et al., 1998, Bim: A novel member of the Bcl-2 family that promotes apoptosis. EMBO J, 17: 384–395. https://doi.org/10.1093/emboj/17.2.384
- Nakano K, Vousden KH, 2001, PUMA, a novel proapoptotic gene, is induced by P53. Mol Cell, 7: 683–694. https://doi.org/10.1016/s1097-2765(01)00214-3
- Cory S, Huang DC, Adams JM, 2003, The Bcl-2 family: Roles in cell survival and oncogenesis. Oncogene, 22: 8590–8607. https://doi.org/10.1038/sj.onc.1207102
- Gross A, McDonnell JM, Korsmeyer SJ, 1999, Bcl-2 family members and the mitochondria in apoptosis. Genes Dev, 13: 1899–1911. https://doi.org/10.1101/gad.13.15.1899
- Shakeri R, Kheirollahi A, Davoodi J, 2017, Apaf-1: Regulation and function in cell death. Biochimie, 135: 111–125. https://doi.org/10.1016/j.biochi.2017.02.001
- Bruckheimer EM, Cho SH, Sarkiss M, et al., 1998, The Bcl-2 gene family and apoptosis. Adv Biochem Eng Biotechnol, 62: 75–105. https://doi.org/10.1007/BFb0102306
- Gloire G, Charlier E, Piette J, 2008, Regulation of Cd95/ Apo-1/Fas-induced apoptosis by protein phosphatases. Biochem Pharmacol, 76: 1451–1458. https://doi.org/10.1016/j.bcp.2008.06.023
- Gottlieb TM, Oren M, 1998, P53 and apoptosis. Semin Cancer Biol, 8: 359–368. https://doi.org/10.1006/scbi.1998.0098
- McMahon SB, 2014, Myc and the control of apoptosis. Cold Spring Harb Perspect Med, 4: a014407. https://doi.org/10.1101/cshperspect.a014407
- Smith GC, d’Adda di Fagagna F, Lakin ND, 1999, Cleavage and inactivation of Atm during apoptosis. Mol Cell Biol, 19: 6076–6084. https://doi.org/10.1128/mcb.19.9.6076
- Bleicken S, Jeschke G, Stegmueller C, et al., 2014, Structural model of active bax at the membrane. Mol Cell, 56: 496–505. https://doi.org/10.1016/j.molcel.2014.09.022
- Borner C, Andrews DW, 2014, The apoptotic pore on mitochondria: Are we breaking through or still stuck? Cell Death Differ, 21: 187–191. https://doi.org/10.1038/cdd.2013.169
- Jeong SH, Lee HW, Han JH, et al., 2008, Low expression of bax predicts poor prognosis in resected non-small cell lung cancer patients with non-squamous histology. Jpn J Clin Oncol, 38: 661–669. https://doi.org/10.1093/jjco/hyn089
- Schuyer M, van der Burg ME, Henzen-Logmans SC, et al., 2001, Reduced expression of bax is associated with poor prognosis in patients with epithelial ovarian cancer: A multifactorial analysis of Tp53, P21, Bax and Bcl-2. Br J Cancer, 85: 1359–1367. https://doi.org/10.1054/bjoc.2001.2101
- Indran IR, Tufo G, Pervaiz S, et al., 2011, Recent advances in apoptosis, mitochondria and drug resistance in cancer cells. Biochim Biophys Acta, 1807: 735–745. https://doi.org/10.1054/bjoc.2001.2101
- Miquel C, Borrini F, Grandjouan S, et al., 2005, Role of bax mutations in apoptosis in colorectal cancers with microsatellite instability. Am J Clin Pathol, 123: 562–570. https://doi.org/10.1309/JQ2X-3RV3-L8F9-TGYW
- Pepper C, Hoy T, Bentley DP, 1997, Bcl-2/Bax ratios in chronic lymphocytic leukaemia and their correlation with in vitro apoptosis and clinical resistance. Br J Cancer, 76: 935–938. https://doi.org/10.1038/bjc.1997.487
- Soengas MS, Capodieci P, Polsky D, et al., 2001, Lowe inactivation of the apoptosis effector Apaf-1 in malignant melanoma. Nature, 409: 207–211. https://doi.org/10.1038/35051606
- Baldi A, Santini D, Russo P, et al., 2004, Analysis of Apaf-1 expression in human cutaneous melanoma progression. Exp Dermatol, 13: 93–97. https://doi.org/10.1111/j.0906-6705.2004.00136.x
- Wan J, Kalpage HA, Vaishnav A, et al., 2019, Regulation of respiration and apoptosis by cytochrome C threonine 58 phosphorylation. Sci Rep, 9: 15815. https://doi.org/10.1038/s41598-019-52101-z
- Kagan VE, Bayir HA, Belikova NA, et al., 2009, Cytochrome C/cardiolipin relations in mitochondria: A kiss of death. Free Radic Biol Med, 46: 1439–1453. https://doi.org/10.1016/j.freeradbiomed.2009.03.004
- Kagan VE, Tyurin VA, Jiang J, et al., 2005, Cytochrome C acts as a cardiolipin oxygenase required for release of proapoptotic factors. Nat Chem Biol, 1: 223–232. https://doi.org/10.1038/nchembio727
- Vladimirov YA, Proskurnina EV, Izmailov DY, et al., 2006, Cardiolipin activates cytochrome C peroxidase activity since it facilitates H(2)O(2) access to heme. Biochemistry (Mosc), 71: 998–1005. https://doi.org/10.1134/s0006297906090082
- Thornberry NA, Lazebnik Y, 1998, Caspases: Enemies within. Science (New York, NY), 281: 1312–1316. https://doi.org/10.1126/science.281.5381.1312
- Nicholson DW, Ali A, Thornberry NA, et al., 1995, Identification and inhibition of the Ice/Ced-3 protease necessary for mammalian apoptosis. Nature, 376: 37–43. https://doi.org/10.1038/376037a0
- Fernandes-Alnemri T, Litwack G, Alnemri ES, 1994, Cpp32, a novel human apoptotic protein with homology to caenorhabditis elegans cell death protein Ced-3 and mammalian interleukin-1 beta-converting enzyme. J Biol Chem, 269: 30761–30764. https://doi.org/10.1016/S0021-9258(18)47344-9
- Cohen GM, 1997, Caspases: The executioners of apoptosis. Biochem J, 326 (Pt 1): 1–16. https://doi.org/10.1042/bj3260001
- Medina V, Edmonds B, Young GP, et al., 1997, Induction of caspase-3 protease activity and apoptosis by butyrate and trichostatin a (inhibitors of histone deacetylase): Dependence on protein synthesis and synergy with a mitochondrial/cytochrome C-dependent pathway. Cancer Res, 57: 3697–3707.
- Asselin E, Mills GB, Tsang BK, 2001, XIAP regulates Akt activity and caspase-3-dependent cleavage during cisplatin-induced apoptosis in human ovarian epithelial cancer cells. Cancer Res, 61: 1862–1868.
- Törmänen-Näpänkangas U, Soini Y, Kahlos K, et al., 2001, Expression of Caspases-3, -6 and -8 and their relation to apoptosis in non-small cell lung carcinoma. Int J Cancer 93: 192–198. https://doi.org/10.1002/ijc.1315
- Hsia JY, Chen CY, Chen JT, et al., 2003, Prognostic significance of caspase-3 expression in primary resected esophageal squamous cell carcinoma. Eur J Surg Oncol, 29: 44–48. https://doi.org/10.1053/ejso.2002.1338
- Kania J, Konturek SJ, Marlicz K, et al., 2003, Expression of Survivin and caspase-3 in gastric cancer. Digest Dis Sci, 48: 266–271. https://doi.org/10.1023/a:1021915124064
- Li Y, Yu H, Zhao L, et al., 2020, Effects of carbon nanotube-mediated caspase3 gene silencing on cardiomyocyte apoptosis and cardiac function during early acute myocardial infarction. Nanoscale, 12: 21599–21604. https://doi.org/10.1039/D0NR05032F
- Vaux DL, Cory S, Adams JM, 1988, Bcl-2 gene promotes haemopoietic cell survival and cooperates with C-Myc to immortalize pre-B cells. Nature, 335: 440–442. https://doi.org/10.1038/335440a0
- Šošić D, Richardson JA, Yu K, et al., 2003, Twist regulates cytokine gene expression through a negative feedback loop that represses Nf-Kappab activity. Cell, 112: 169–180. https://doi.org/10.1016/s0092-8674(03)00002-3
- Liu JR, Chen BQ, Yang YM, et al., 2002, Effect of apoptosis on gastric adenocarcinoma cell line Sgc-7901 induced by Cis-9, Trans-11-conjugated linoleic acid. World J Gastroenterol, 8: 999–1004. https://doi.org/10.3748/wjg.v8.i6.999
- Zhao AG, Zhao HL, Jin XJ, et al., 2002, Effects of Chinese Jianpi herbs on cell apoptosis and related gene expression in human gastric cancer grafted onto nude mice. World J Gastroenterol, 8: 792–796. https://doi.org/10.3748/wjg.v8.i5.792
- Wu YL, Sun B, Zhang XJ, et al., 2001, Growth inhibition and apoptosis induction of sulindac on human gastric cancer cells. World J Gastroenterol, 7: 796–800. https://doi.org/10.3748/wjg.v7.i6.796
- Siervo-Sassi RR, Marrangoni AM, Feng X, et al., 2003, Physiological and molecular effects of Apo2l/trail and cisplatin in ovarian carcinoma cell lines. Cancer Lett, 190: 61–72. https://doi.org/10.1016/s0304-3835(02)00579-7
- Rudin CM, Yang Z, Schumaker LM, et al., 2003, Cullen inhibition of glutathione synthesis reverses Bcl-2- mediated cisplatin resistance. Cancer Res, 63: 312–318.
- Chen J, 2016, The cell-cycle arrest and apoptotic functions of P53 in tumor initiation and progression. Cold Spring Harb Perspect Med, 6: a026104. https://doi.org/10.1101/cshperspect.a026104
- Edison N, Zuri D, Maniv I, et al., 2012, The Iap-antagonist arts initiates caspase activation upstream of cytochrome C and Smac/Diablo. Cell Death Differ, 19: 356–368. https://doi.org/10.1038/cdd.2011.112
- Raffo AJ, Perlman H, Chen MW, et al.,1995, Overexpression of Bcl-2 protects prostate cancer cells from apoptosis in vitro and confers resistance to androgen depletion in vivo. Cancer Res, 55: 4438–4445.
- Fulda S, Meyer E, Debatin KM, 2002, Inhibition of trail-induced apoptosis by Bcl-2 overexpression. Oncogene, 21: 2283–2294. https://doi.org/10.1038/sj.onc.1205258
- Foreman KE, Wrone-Smith T, Boise LH, et al., 1996, Kaposi’s sarcoma tumor cells preferentially express Bcl-Xl. Am J Pathol, 149: 795–803.
- Krajewska M, Moss SF, Krajewski S, et al., 1996, Elevated expression of Bcl-X and reduced bak in primary colorectal adenocarcinomas. Cancer Res, 56: 2422–2427.
- Han JY, Hong EK, Choi BG, et al., 2003, Death receptor 5 and Bcl-2 protein expression as predictors of tumor response to gemcitabine and cisplatin in patients with advanced non-small-cell lung cancer. Med Oncol (Northwood, London, England), 20: 355–362. https://doi.org/10.1385/MO:20:4:355
- Erovic BM, Pelzmann M, Grasl M, et al., 2005, Mcl-1, vascular endothelial growth factor-R2, and 14-3-3sigma expression might predict primary response against radiotherapy and chemotherapy in patients with locally advanced squamous cell carcinomas of the head and neck. Clin Cancer Res, 11: 8632–8636. https://doi.org/10.1158/1078-0432.CCR-05-1170
- Williams J, Lucas PC, Griffith KA, et al., 2005, Expression of Bcl-Xl in ovarian carcinoma is associated with chemoresistance and recurrent disease. Gynecol Oncol, 96: 287–295. https://doi.org/10.1016/j.ygyno.2004.10.026
- Michaud WA, Nichols AC, Mroz EA, et al., 2009, Bcl-2 blocks cisplatin-induced apoptosis and predicts poor outcome following chemoradiation treatment in advanced oropharyngeal squamous cell carcinoma. Clin Cancer Res, 15: 1645–1654. https://doi.org/10.1158/1078-0432.CCR-08-2581
- Yang D, Chen MB, Wang LQ, et al., 2013, Bcl-2 expression predicts sensitivity to chemotherapy in breast cancer: A systematic review and meta-analysis. J Exp Clin Cancer Res, 32: 105. https://doi.org/10.1186/1756-9966-32-105
- Edison N, Curtz Y, Paland N, et al., 2017, Degradation of Bcl-2 by Xiap and Arts promotes apoptosis. Cell Rep, 21: 442–454. https://doi.org/10.1016/j.celrep.2017.09.052
- Wang X, Simpson ER, Brown KA, 2015, P53: Protection against tumor growth beyond effects on cell cycle and apoptosis. Cancer Res, 75: 5001–5007. https://doi.org/10.1158/0008-5472.CAN-15-0563
- Yu G, Luo H, Zhang N, et al., 2019, Loss of P53 sensitizes cells to palmitic acid-induced apoptosis by reactive oxygen species accumulation. Int J Mol Sci, 20: 6268. https://doi.org/10.3390/ijms20246268
- Vousden KH, 2000, P53: Death star. Cell, 103: 691–694. https://doi.org/10.1016/s0092-8674(00)00171-9
- Chi SW, 2014, Structural insights into the transcription-independent apoptotic pathway of P53. BMB Rep, 47: 167–172. https://doi.org/10.5483/BMBRep.2014.47.3.261
- Tomita Y, Marchenko N, Erster S, et al., 2006, WT P53, but not tumor-derived mutants, bind to Bcl2 via the DNA binding domain and induce mitochondrial permeabilization. J Biol Chem, 281: 8600–8606. https://doi.org/10.1074/jbc.M507611200
- Marchenko ND, Moll UM, 2014, Mitochondrial death functions of P53. Mol Cell Oncol, 1: e955995. https://doi.org/10.1080/23723548.2014.955995
- Moll UM, Zaika A, 2001, Nuclear and mitochondrial apoptotic pathways of P53. FEBS Lett, 493: 65–69. https://doi.org/10.1016/s0014-5793(01)02284-0
- Mihara M, Erster S, Zaika A, et al., 2003, P53 Has a direct apoptogenic role at the mitochondria. Mol Cell, 11: 577– 590. https://doi.org/10.1016/s1097-2765(03)00050-9
- Aubrey BJ, Kelly GL, Janic A, et al., 2018, How does P53 induce apoptosis and how does this relate to P53-mediated tumour suppression? Cell Death Differ, 25: 104–113. https://doi.org/10.1038/cdd.2017.169
- Vogelstein B, Lane D, Levine AJ, 2000, Surfing the P53 network. Nature, 408: 307–310. https://doi.org/10.1038/35042675
- Donepudi M, Grütter MG, 2002, Structure and zymogen activation of caspases. Biophys Chem, 101–102: 145–153. https://doi.org/10.1016/s0301-4622(02)00151-5
- O’Connor L, Harris AW, Strasser A, 2000, Cd95 (Fas/Apo- 1) and P53 signal apoptosis independently in diverse cell types. Cancer Res, 60: 1217–1220.
- Liu X, Yue P, Khuri FR, et al., 2004, P53 upregulates death receptor 4 expression through an intronic P53 binding site. Cancer Res, 64: 5078–5083. https://doi.org/10.1158/0008-5472.CAN-04-1195
- Oda E, Ohki R, Murasawa H, et al., 2000, Noxa a Bh3- only member of the Bcl-2 family and candidate mediator of P53-induced apoptosis. Science (New York, NY), 288: 1053–1058. https://doi.org/10.1126/science.288.5468.1053
- Fridman JS, Lowe SW, 2003, Control of apoptosis by P53. Oncogene, 22: 9030–9040. https://doi.org/10.1038/sj.onc.1207116
- Hao Q, Chen J, Liao J, et al., 2021, P53 induces arts to promote mitochondrial apoptosis. Cell Death Dis, 12: 204. https://doi.org/10.1038/s41419-021-03463-8
- Liu T, Wang L, Chen H, et al., 2017, Molecular and cellular mechanisms of apoptosis during dissociated spermatogenesis. Front Physiol, 8: 188. https://doi.org/10.3389/fphys.2017.00188
- Hancock JF, 2003, Ras proteins: Different signals from different locations. Nat Rev Mol Cell Biol, 4: 373–384. https://doi.org/10.1038/nrm1105
- Alzahrani AS, 2019, Pi3k/Akt/Mtor inhibitors in cancer: At the bench and bedside. Semin Cancer Biol, 59: 125–132. https://doi.org/10.1016/j.semcancer.2019.07.009
- Carnero A, Blanco-Aparicio C, Renner O, et al., 2008, Leal The Pten/Pi3k/Akt signalling pathway in cancer, therapeutic implications. Curr Cancer Drug Targets, 8: 187–198. https://doi.org/10.2174/156800908784293659
- Manning BD, Toker A, 2017, Akt/Pkb signaling: Navigating the network. Cell, 169: 381–405. https://doi.org/10.1016/j.cell.2017.04.001
- Dummler B, Hemmings BA, 2007, Physiological roles of Pkb/Akt isoforms in development and disease. Biochem Soc Trans, 35: 231–235. https://doi.org/10.1042/BST0350231
- Szymonowicz K, Oeck S, Malewicz NM, et al., 2018, New Insights into protein kinase B/Akt signaling: Role of localized Akt Activation and compartment-specific target proteins for the cellular radiation response. Cancers, 10: 78. https://doi.org/10.3390/cancers10030078
- Revathidevi S, Munirajan AK, 2019, Akt in Cancer: Mediator and more. Semin Cancer Biol, 59: 80–91. https://doi.org/10.1016/j.semcancer.2019.06.002
- Risso G, Blaustein M, Pozzi B, et al., 2015, Akt/Pkb: One kinase, many modifications. Biochem J, 468: 203–214. https://doi.org/10.1042/BJ20150041
- Reyes-Farias M, Carrasco-Pozo C, 2019, The anti-cancer effect of quercetin: Molecular implications in cancer metabolism. Int J Mol Sci, 20: 3177. https://doi.org/10.3390/ijms20133177
- Liu R, Chen Y, Liu G, et al., 2020, Pi3k/Akt pathway as a key link modulates the multidrug resistance of cancers. Cell Death Dis, 11: 797. https://doi.org/10.1038/s41419-020-02998-6
- Sochalska M, Tuzlak S, Egle A, et al., 2015, Lessons from gain- and loss-of-function models of pro-survival Bcl2 family proteins: Implications for targeted therapy. FEBS J, 282: 834–849. https://doi.org/10.1111/febs.13188
- Choi E, Kim E, Kim JH, et al., 2019, Akt1-targeted proapoptotic activity of compound K in human breast cancer cells. J Ginseng Res, 43: 692–698. https:/doi.org/10.1016/j.jgr.2019.07.001
- Adams JM, Cory S, 2018, The Bcl-2 arbiters of apoptosis and their growing role as cancer targets. Cell Death Differ, 25: 27–36. https://doi.org/10.1038/cdd.2017.161
- Chen YH, Yang SF, Yang CK, et al., 2021, Metformin induces apoptosis and inhibits migration by activating the Ampk/P53 axis and suppressing Pi3k/Akt signaling in human cervical cancer cells. Mol Med Rep, 23: 88. https://doi.org/10.3892/mmr.2020.11725
- Viollet B, Horman S, Leclerc J, et al., 2010, AMPK inhibition in health and disease. Crit Rev Biochem Mol Biol, 45: 276–295. https://doi.org/10.3109/10409238.2010.488215
- Gui D, Cui Z, Zhang L, et al., 2017, Salidroside Attenuates hypoxia-induced pulmonary arterial smooth muscle cell proliferation and apoptosis resistance by upregulating autophagy through the Ampk-Mtor-Ulk1 pathway. BMC Pulm Med, 17: 191. https://doi.org/10.1186/s12890-017-0477-4
- White JP, Billin AN, Campbell ME, et al., 2018, The Ampk/P27(Kip1) axis regulates autophagy/apoptosis decisions in aged skeletal muscle stem cells. Stem Cell Rep, 11: 425–439. https://doi.org/10.1016/j.stemcr.2018.06.014
- Nitulescu GM, Van De Venter M, Nitulescu G, et al., 2018, The Akt pathway in oncology therapy and beyond (review). Int J Oncol, 53: 2319–2331. https://doi.org/10.3892/ijo.2018.4597
- Cha JH, Yang WH, Xia W, et al., 2018, Metformin promotes antitumor immunity via endoplasmic-reticulum-associated degradation of Pd-L1. Mol Cell, 71: 606–620.e607. https://doi.org/10.3892/ijo.2018.4597
- Deng M, Yang X, Qin B, et al., 2016, Deubiquitination and activation of Ampk by Usp10. Mol Cell, 61: 614–624. https://doi.org/10.1016/j.molcel.2016.01.010
- Faubert B, Vincent EE, Poffenberger MC, 2015, The Amp-activated protein kinase (Ampk) and cancer: Many faces of a metabolic regulator. Cancer Lett, 356: 165–170. https://doi.org/10.1074/jbc.RA119.010243
- Roberts DJ, Miyamoto S, 2015, Hexokinase Ii integrates energy metabolism and cellular protection: Akting on mitochondria and torcing to autophagy. Cell Death Differ, 22: 248–257. https://doi.org/10.1038/cdd.2014.173
- Laplante M, Sabatini DM, 2012, Mtor signaling in growth control and disease. Cell, 149: 274–293. https://doi.org/10.1016/j.cell.2012.03.017
- Lien EC, Dibble CC, Toker A, 2017, Pi3k signaling in cancer: Beyond Akt. Curr Opin Cell Biol, 45: 62–71. https://doi.org/10.1016/j.ceb.2017.02.007
- Lien EC, Lyssiotis CA, Cantley LC, 2016, Metabolic reprogramming by the Pi3k-Akt-Mtor pathway in cancer. Recent Results Cancer Res, 207: 39–72. https://doi.org/10.1007/978-3-319-42118-6_3
- Sui X, Kong N, Ye L, et al., 2014, P38 and Jnk Mapk pathways control the balance of apoptosis and autophagy in response to chemotherapeutic agents. Cancer Lett, 344: 174–179. https://doi.org/10.1016/j.canlet.2013.11.019
- Chen M, Sun J, Lu C, et al., 2016, The impact of neuronal Notch-1/Jnk pathway on intracerebral hemorrhage-induced neuronal injury of rat model. Oncotarget, 7: 73903–73911. https://doi.org/10.18632/oncotarget.12094
- Musi CA, Agrò G, Santarella F, et al., 2020, Jnk3 as Therapeutic target and biomarker in neurodegenerative and neurodevelopmental brain diseases. Cells, 9: 2190. https://doi.org/10.3390/cells9102190
- Tan J, Gao W, Yang W, et al., 2021, Isoform-specific functions of C-Jun N-terminal kinase 1 and 2 in lung ischemia-reperfusion injury through the C-Jun/activator protein-1 pathway. J Thorac Cardiovasc Surg, 162: e143– e156. https://doi.org/10.1016/j.jtcvs.2020.03.083
- Wang H, Zhong L, Mi S, et al., 2020, Tanshinone Iia prevents platelet activation and down-regulates Cd36 and Mkk4/Jnk2 signaling pathway. BMC Cardiovasc Disord, 20: 81. https://doi.org/10.1186/s12872-019-01289-z
- Desai S, Laskar S, Pandey BN, 2013, Autocrine Il-8 and Vegf mediate epithelial-mesenchymal transition and invasiveness via P38/Jnk-Atf-2 signalling in A549 lung cancer cells. Cell Signal, 25: 1780–1791. https://doi.org/10.1016/j.cellsig.2013.05.025
- Ngoei KR, Catimel B, Church N, et al., 2011, Characterization of a Novel Jnk (C-Jun N-terminal kinase) inhibitory peptide. Biochem J, 434: 399–413. https://doi.org/10.1042/BJ20101244
- Matsui Y, Kuwabara T, Eguchi T, et al., 2018, Acetylation regulates the Mkk4-Jnk pathway in T cell receptor signaling. Immunol Lett, 194: 21–28. https://doi.org/10.1016/j.imlet.2017.12.00
- Paul M, Hemshekhar M, Thushara RM, et al., 2015, Methotrexate promotes platelet apoptosis via Jnk-mediated mitochondrial damage: Alleviation by N-acetylcysteine and N-acetylcysteine amide. PLoS One, 10: e0127558. https://doi.org/10.1038/sj.onc.1205258
- Li Y, Zhou Y, Yue X, et al., 2021, Cyanine conjugates in cancer theranostics. Bioact Mater, 6: 794–809. https://doi.org/10.1016/j.bioactmat.2020.09.009
- Fang M, Xia S, Bi J, et al., 2018, A Cyanine-based fluorescent cassette with aggregation-induced emission for sensitive detection of Ph changes in live cells. Chem Commun (Cambridge, England), 54: 1133–1136. https://doi.org/10.1039/C7CC08986D
- Gui L, Yuan Z, Kassaye H, et al., 2018, A Tumor-targeting probe based on a mitophagy process for live imaging. Chem Commun (Cambridge, England), 54: 9675–9678. https://doi.org/10.1039/C8CC04246B
- Liu R, Tang J, Xu Y, et al., 2019, Bioluminescence imaging of inflammation in vivo based on bioluminescence and fluorescence resonance energy transfer using nanobubble ultrasound contrast agent. ACS Nano, 13: 5124–5132. https://doi.org/10.1021/acsnano.8b08359
- Cao J, Chi J, Xia J, et al., 2019, Iodinated cyanine dyes for fast near-infrared-guided deep tissue synergistic phototherapy. ACS Appl Mater Interfaces, 11: 25720– 25729. https://doi.org/10.1021/acsami.9b07694
- Waszkiewicz M, Choromanska A, Kulbacka J, et al., 2022, The photodynamic reaction with Ir-775 cyanine combined with 2-methoxyestradiol in ovarian (Skov-3) and human breast adenocarcinoma (Mda Mb-231) cell lines. Photodiagn Photodyn Ther, 38: 102766. https://doi.org/10.1016/j.pdpdt.2022.102766
- Choi SY, Baek SH, Chang SJ, et al., 2017, Synthesis of upconversion nanoparticles conjugated with graphene oxide quantum dots and their use against cancer cell imaging and photodynamic therapy. Biosens Bioelectron, 93: 267–273. https://doi.org/10.1016/j.bios.2016.08.094
- Chudy M, Tokarska K, Jastrzębska E, et al., 2018, Lab-on-a-chip systems for photodynamic therapy investigations. Biosens Bioelectron, 101: 37–51. https://doi.org/10.1016/j.bios.2017.10.013
- Shamjith S, Joseph MM, Murali VP, et al., 2022, NADH-depletion triggered energy shutting with cyclometalated iridium (Iii) complex enabled bimodal luminescence-sers sensing and photodynamic therapy. Biosens Bioelectron, 204: 114087. https://doi.org/10.1016/j.bios.2022.114087
- Bilici K, Cetin S, Celikbas E, et al., 2021, Recent advances in cyanine-based phototherapy agents. Front Chem, 9: 707876. https://doi.org/10.3389/fchem.2021.707876
- Kadigamuwa CC, Le VQ, Wimalasena K, 2015, 2, 2’- and 4, 4’-Cyanines are transporter-independent in vitro dopaminergic toxins with the specificity and mechanism of toxicity similar to Mpp+. J Neurochem, 135: 755–767. https://doi.org/10.1111/jnc.13201
- Yang N, Gilman P, Mirzayans R, et al., 2015, Characterization of the apoptotic response induced by the cyanine dye D112: A potentially selective anti-cancer compound. PLoS One, 10: e0125381. https://doi.org/10.1371/journal.pone.0125381
- Zhai X, Yuan S, Yang X, et al., 2018, Growth-inhibition of S180 residual-tumor by combination of cyclophosphamide and chitosan oligosaccharides in vivo. Life Sci, 202: 21–27. https://doi.org/10.1016/j.lfs.2018.04.004
- Zhai X, Yuan S, Yang X, et al., 2019, Chitosan Oligosaccharides induce apoptosis in human renal carcinoma via reactive-oxygen-species-dependent endoplasmic reticulum stress. J Agric Food Chem, 67: 1691–1701. https://doi.org/10.1021/acs.jafc.8b06941
- Li X, Lovell JF, Yoon J, et al., 2020, Clinical development and potential of photothermal and photodynamic therapies for cancer. Nat Rev Clin Oncol, 17: 657–674.
- Dolmans DE, Fukumura D, Jain RK, 2003, Photodynamic therapy for cancer. Nat Rev Cancer, 3: 380–387. https://doi.org/10.1038/nrc1071
- Hou X, Tao Y, Pang Y, et al., 2018, Nanoparticle-based photothermal and photodynamic immunotherapy for tumor treatment. Int J Cancer, 143: 3050–3060. https://doi.org/10.1002/ijc.31717
- Plenagl N, Duse L, Seitz BS, et al., 2019, Photodynamic therapy - hypericin tetraether liposome conjugates and their antitumor and antiangiogenic activity. Drug Deliv, 26: 23–33. https://doi.org/10.1080/10717544.2018.1531954
- Liu J, Wang L, Shen R, et al., 2022, Novel heptamethine cyanine photosensitizer for fret-amplified photodynamic therapy and two-photon imaging in a-549 cells. Spectrochim Acta Part A Mol Biomol Spectrosc, 274: 121083. https://doi.org/10.1016/j.saa.2022.121083
- Zhao X, Yang Y, Yu Y, et al., 2019, A cyanine-derivative photosensitizer with enhanced photostability for mitochondria-targeted photodynamic therapy. Chem Commun (Cambridge, England), 55: 13542–13545. https://doi.org/10.1039/C9CC06157F
- Murren JR, 2002, Modulating multidrug resistance: Can we target this therapy? Clin Cancer Res, 8: 633–635.
- Kessel D, Reiners JJ Jr., 2007, Apoptosis and autophagy after mitochondrial or endoplasmic reticulum photodamage. Photochem Photobiol, 83: 1024–1028. https://doi.org/10.1111/j.1751-1097.2007.00088.x
- Buytaert E, Dewaele M, Agostinis P, 2007, Molecular effectors of multiple cell death pathways initiated by photodynamic therapy. Biochim Biophys Acta, 1776: 86–107. https://doi.org/10.1016/j.bbcan.2007.07.001
- Kulbacka J, Choromańska A, Drąg-Zalesińska M, et al., 2020, Proapoptotic activity induced by photodynamic reaction with novel cyanine dyes in caspase-3-deficient human breast adenocarcinoma cell lines (Mcf/Wt and Mcf/Dx). Photodiagnosis Photodyn Ther, 30: 101775. https://doi.org/10.1016/j.pdpdt.2020.101775
- Krejcir R, Briza T, Sterba M, et al., 2020, Anticancer pentamethinium salt is a potent photosensitizer inducing mitochondrial disintegration and apoptosis upon red light illumination. J Photochem Photobiol B, 209: 111939. https://doi.org/10.1016/j.jphotobiol.2020.111939
- Krejcir R, Krcova L, Zatloukalova P, et al., 2019, A cyclic pentamethinium salt induces cancer cell cytotoxicity through mitochondrial disintegration and metabolic collapse. Int J Mol Sci, 20: 4208. https://doi.org/10.3390/ijms20174208
- Wezgowiec J, Kotulska M, Saczko J, et al., 2013, Cyanines in photodynamic reaction assisted by reversible electroporation--in vitro study on human breast carcinoma cells. Photodiagnosis Photodyn Ther, 10: 490–502. https://doi.org/10.1016/j.pdpdt.2013.04.004.
- Flaumenhaft R, Tanaka E, Graham GJ, et al., 2007, Localization and quantification of platelet-rich thrombi in large blood vessels with near-infrared fluorescence imaging. Circulation, 115: 84–93. https://doi.org/10.1161/CIRCULATIONAHA.106.643908
- Cai Z, Yu J, Hu J, et al., 2023, Three near-Infrared and lysosome-targeting probes for photodynamic therapy (Pdt). Spectrochim Acta Part A Mol Biomol Spectrosc, 286: 122027. https://doi.org/10.1016/j.saa.2022.122027
- Kong C, Chen X, 2022, Combined photodynamic and photothermal therapy and immunotherapy for cancer treatment: A review. Int J Nanomed, 17: 6427–6446. https://doi.org/10.2147/IJN.S388996
- Li P, Liu Y, Liu W, et al., 2019, Ir-783 inhibits breast cancer cell proliferation and migration by inducing mitochondrial fission. Int J Oncol, 55: 415–424. https://doi.org/10.3892/ijo.2019.4821
- Tang Q, Liu W, Zhang Q, et al., 2018, Dynamin-related protein 1-mediated mitochondrial fission contributes to Ir-783-induced apoptosis in human breast cancer cells. J Cell Mol Med, 22: 4474–4485. https://doi.org/10.1111/jcmm.13749
- Hou L, Yang X, Ren J, et al., 2016, A novel redox-sensitive system based on single-walled carbon nanotubes for chemo-photothermal therapy and magnetic resonance imaging. Int J Nanomed, 11: 607–624. https://doi.org/10.2147/IJN.S98476
- Wu JB, Shi C, Chu GC, et al., 2015, Near-infrared fluorescence heptamethine carbocyanine dyes mediate imaging and targeted drug delivery for human brain tumor. Biomaterials, 67: 1–10. https://doi.org/10.1016/j.biomaterials.2015.07.028
- Shao C, Liao CP, Hu P, et al., 2014, Detection of live circulating tumor cells by a class of near-infrared heptamethine carbocyanine dyes in patients with localized and metastatic prostate cancer. PLoS One, 9: e88967. https://doi.org/10.1371/journal.pone.0088967
- Cohen S, Margel S, 2012, Engineering of near Ir fluorescent albumin nanoparticles for in vivo detection of colon cancer. J Nanobiotechnol, 10: 36. https://doi.org/10.1186/1477-3155-10-36
- Zhang J, Liu Z, Lian P, et al., 2016, Selective imaging and cancer cell death via Ph switchable near-infrared fluorescence and photothermal effects. Chem Sci, 7: 5995–6005. https://doi.org/10.1039/C6SC00221H
- Thomas RG, Jeong YY, 2017, NIRF heptamethine cyanine dye nanocomplexes for multi modal theranosis of tumors. Chonnam Med J, 53: 83–94. https://doi.org/10.4068/cmj.2017.53.2.83
- Yang X, Shi C, Tong R, et al., 2010, Near Ir Heptamethine cyanine dye-mediated cancer imaging. Clin Cancer Res, 16: 2833–2844. https://doi.org/10.1158/1078-0432.CCR-10-0059
- Yuan J, Yi X, Yan F, et al., 2015, Near-infrared fluorescence imaging of prostate cancer using heptamethine carbocyanine dyes. Mol Med Rep, 11: 821–828. https://doi.org/10.3892/mmr.2014.2815
- Sun C, Wang J, Xia T, et al., 2022, Mitochondrion-targeted NIR therapeutic agent suppresses melanoma by inducing apoptosis and cell cycle arrest via E2f/Cyclin/Cdk pathway. Pharmaceuticals (Basel, Switzerland), 15: 1589. https://doi.org/10.3390/ph15121589
- Cai H, Wang R, Guo X, et al., 2021, Combining gemcitabine-loaded macrophage-like nanoparticles and erlotinib for pancreatic cancer therapy. Mol Pharm, 18: 2495–2506. https://doi.org/10.1021/acs.molpharmaceut.0c01225
- Zhan Y, Ma W, Zhang Y, et al., 2019, DNA-based nanomedicine with targeting and enhancement of therapeutic efficacy of breast cancer cells. ACS Appl Mater Interfaces, 11: 15354–15365. https://doi.org/10.1021/acsami.9b03449
- Hou YJ, Yang XX, Liu RQ, et al., 2020, Pathological mechanism of photodynamic therapy and photothermal therapy based on nanoparticles. Int J Nanomed, 15: 6827–6838. https://doi.org/10.2147/IJN.S269321
- Zhang Y, Lv T, Zhang H, et al., 2017, Folate and Heptamethine cyanine modified chitosan-based nanotheranostics for tumor targeted near-infrared fluorescence imaging and photodynamic therapy. Biomacromolecules, 18: 2146–2160. https://doi.org/10.1021/acs.biomac.7b00466
- Rizvi SF, Mu S, Zhao C, et al., 2022, Fabrication of self-assembled peptide nanoparticles for in vitro assessment of cell apoptosis pathway and in vivo therapeutic efficacy. Mikrochim Acta, 189: 53. https://doi.org/10.1007/s00604-021-05148-7
- Han X, Wang R, Song X, et al., 2018, A mitochondrial-targeting near-infrared fluorescent probe for bioimaging and evaluating endogenous superoxide anion changes during ischemia/reperfusion injury. Biomaterials, 156: 134–146. https://doi.org/10.1016/j.biomaterials.2017.11.039
- Cooper E, Choi PJ, Hwang K, et al., 2023, Elucidating the cellular uptake mechanisms of heptamethine cyanine dye analogues for their use as an anticancer drug-carrier molecule for the treatment of glioblastoma. Chem Biol Drug Des, 101: 696–716. https://doi.org/10.1111/cbdd.14171
- Chi J, Ma Q, Shen Z, et al., 2020, Targeted nanocarriers based on iodinated-cyanine dyes as immunomodulators for synergistic phototherapy. Nanoscale, 12: 11008–11025. https://doi.org/10.1111/cbdd.14171
- Yu Z, Meng X, Zhang S, et al., 2021, Ir-808 loaded nanoethosomes for aggregation-enhanced synergistic transdermal photodynamic/photothermal treatment of hypertrophic scars. Biomater Sci, 10: 158–166. https://doi.org/10.1039/D1BM01555A