Identification of hotspots in synthetic peptide inhibitors of the FOXO4:p53 interaction
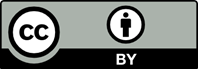
Forkhead box protein O4 (FOXO4) plays a pivotal role in cellular senescence by binding to and inactivating p53. Consequently, misregulation of the FOXO4:p53 complex is associated with numerous diseases. Targeting the FOXO4-p53 interface has been achieved using a synthetic D-retro-inverso (DRI) peptide derived from the forkhead-homology domain of FOXO4 (FOXO4-FDH), also known as DRI (FOXO4-FHD residues 91–124). However, a comprehensive understanding of the key amino acids driving the interaction between DRI and p53 remains incomplete. While previous publications have demonstrated a robust interaction between the forkhead homology domain of FOXO4 (FOXO4-FHD) and the transactivation domain of p53 (p53-TAD), emerging evidence suggests that the interaction within the binary complex forms a highly interconnected network, including a predicted interaction between FOXO4-FHD and the DNA-binding domain of p53 (p53-DBD). In this study, we investigated the DRI: p53-DBD interaction by measuring the binding affinities of DRI and the native peptide of FOXO4, from which it is derived, to p53-DBD using microscale thermophoresis and computational modeling. Our in vitro measurements reveal that DRI binds to p53-DBD with high affinity (Kd ~50 nM), while the native peptide exhibits significantly weaker binding affinity (Kd ~2.5 mM), implying distinct modes of interaction. Subsequently, we created an in silico model of the DRI: p53-DBD interaction, which we analyzed to identify potential interaction hotspots. The analysis of this model suggests that a truncated DRI peptide (FOXO4-FHD amino acids 101–109) retains the majority of the binding affinity, as subsequently demonstrated in vitro (Kd ~40 nM). Collectively, this data furnishes molecular-level insights that contribute to the understanding of the interplay of the amino acids between DRI and p53, further supporting the notion of domain rearrangement or refolding during the formation of the FOXO4:p53 complex. In addition, this data provides an additional basis for the design of small molecules aimed at inhibiting this interaction.
- Muñoz-Espín D, Cañamero M, Maraver A, et al., 2013, Programmed cell senescence during mammalian embryonic development. Cell, 155(5): 1104–1118. https://doi.org/10.1016/j.cell.2013.10.019
- Coppé JP, Desprez PY, Krtolica A, et al., 2010, The senescence-associated secretory phenotype: The dark side of tumor suppression. Annu Rev Pathol, 5: 99–118. https://doi.org/10.1146/annurev-pathol-121808-102144
- Young AR, Narita M, 2009, SASP reflects senescence. EMBO Rep, 10(3): 228–230. https://doi.org/10.1038/embor.2009.22
- Birch J, Gil J, 2020, Senescence and the SASP: Many therapeutic avenues. Genes Dev, 34(23–24): 1565–1576. https://doi.org/10.1101/gad.343129.120
- Campisi J, 2000, Cancer, aging and cellular senescence. In Vivo, 14(1): 183–188.
- Marei HE, Althani A, Afifi N, et al., 2021, p53 signaling in cancer progression and therapy. Cancer Cell Int, 21(1): 703. https://doi.org/10.1186/s12935-021-02396-8
- Joerger AC, Fersht AR, 2010, The tumor suppressor p53: From structures to drug discovery. Cold Spring Harb Perspect Biol, 2(6): a000919. https://doi.org/10.1101/cshperspect.a000919
- Aubrey BJ, Kelly GL, Janic A, et al., 2018, How does p53 induce apoptosis and how does this relate to p53-mediated tumour suppression? Cell Death Differ, 25: 104–113. https://doi.org/10.1038/cdd.2017.169
- Fischer M, 2017, Census and evaluation of p53 target genes. Oncogene, 36(28): 3943–3956. https://doi.org/10.1038/onc.2016.502
- Harris CC, 1996, p53 tumor suppressor gene: At thecrossroads of molecular carcinogenesis, molecular epidemiology, and cancer risk assessment. Environ Health Perspect, 104 Suppl 3(Suppl 3): 435–439. https://doi.org/10.1289/ehp.96104s3435
- Baar MP, Brandt RM, Putavet DA, et al., 2017, Targeted apoptosis of senescent cells restores tissue homeostasis in response to chemotoxicity and aging. Cell, 169(1): 132–147.e16. https://doi.org/10.1016/j.cell.2017.02.031
- Bourgeois B, Madl T, 2018, Regulation of cellular senescence via the FOXO4-p53 axis. FEBS Lett, 592(12): 2083–2097. https://doi.org/10.1002/1873-3468.13057
- Rodier F, Muñoz DP, Teachenor R, et al., 2011, DNA-SCARS: Distinct nuclear structures that sustain damage-induced senescence growth arrest and inflammatory cytokine secretion. J Cell Sci, 124(Pt 1): 68–81. https://doi.org/10.1242/jcs.071340
- Wang L, Wang N, Zhang W, et al., 2022, Therapeutic peptides: Current applications and future directions. Signal Transduct Target Ther, 7(1): 48. https://doi.org/10.1038/s41392-022-00904-4
- Mandal R, Kohoutova K, Petrvalska O, et al., 2022, FOXO4 interacts with p53 TAD and CRD and inhibits its binding to DNA. Protein Sci, 31(5): e4287. https://doi.org/10.1002/pro.4287
- Le HH, Cinaroglu SS, Manalo EC, et al., 2021, Molecular modelling of the FOXO4-TP53 interaction to design senolytic peptides for the elimination of senescent cancer cells. EBioMedicine, 73: 103646. https://doi.org/10.1016/j.ebiom.2021.103646
- Kim J, Ahn D, Park CJ, 2022, Biophysical investigation of the dual binding surfaces of human transcription factors FOXO4 and p53. FEBS J, 289(11): 3163–3182. https://doi.org/10.1111/febs.16333
- Dümmler A, Lawrence AM, de Marco A, 2005, Simplified screening for the detection of soluble fusion constructs expressed in E. coli using a modular set of vectors. Microb Cell Fact, 4: 34. https://doi.org/10.1186/1475-2859-4-34
- Caporale A, Doti N, Sandomenico A, et al., 2017, Evaluation of combined use of Oxyma and HATU in aggregating peptide sequences. J Pept Sci, 23(4): 272–281. https://doi.org/10.1002/psc.2977
- Caporale A, Doti N, Monti A, et al., 2018, Automatic procedures for the synthesis of difficult peptides using oxyma as activating reagent: A comparative study on the use of bases and on different deprotection and agitation conditions. Peptides, 102: 38–46. https://doi.org/10.1016/j.peptides.2018.02.006
- Nanotempertech, 2021, Protein Labeling-improved Quantitation of Biomolecular Interactions by MST Using the His-tag Labeling Kit RED-tris-NTA 2nd Generation. Available from: https://resources.nanotempertech.com/technical-notes [Last accessed on 2023 Jun 20].
- Van Zundert GC, Rodrigues JP, Trellet M, et al., 2016, The HADDOCK2.2 web server: User-friendly integrative modeling of biomolecular complexes. J Mol Biol, 428: 720–725. https://doi.org/10.1016/j.jmb.2015.09.014
- Petty TJ, Emamzadah S, Costantino L, et al., 2011, An induced fit mechanism regulates p53 DNA binding kinetics to confer sequence specificity. EMBO J, 30(11): 2167–2176. https://doi.org/10.1038/emboj.2011.127
- Boura E, Rezabkova L, Brynda J, et al., 2010, Structure of the human FOXO4-DBD-DNA complex at 1.9 Å resolution reveals new details of FOXO binding to the DNA. Acta Crystallogr D Biol Crystallogr, 66(Pt 12): 1351–1357. https://doi.org/10.1107/S0907444910042228
- Kuriata A, Gierut AM, Oleniecki T, et al., 2018, CABS-flex 2.0: A web server for fast simulations of flexibility of protein structures. Nucleic Acids Res, 46(W1): W338–W343. https://doi.org/10.1093/nar/gky356
- Pettersen EF, Goddard TD, Huang CC, et al., 2021, UCSF ChimeraX: Structure visualization for researchers, educators, and developers. Protein Sci, 30(1): 70–82. https://doi.org/10.1002/pro.3943
- Sela M, Zisman E, 1997, Different roles of D-amino acids in immune phenomena. FASEB J, 11(6): 449–456. https://doi.org/10.1096/fasebj.11.6.9194525