ATPS bioinks and microgel formation for bioprinting
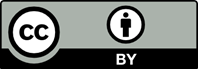
Biological tissues possess intricate hierarchical structures that enable diverse cellular functions, which are critical for maintaining physiological processes. Mimicking these properties is central to advancing tissue engineering and regenerative medicine. Aqueous two-phase systems (ATPS)-derived microgel bioinks have emerged as a versatile platform, offering biocompatibility, mechanical tunability, and multifunctionality for bioprinting applications. Recent advancements, such as oxygen-releasing constructs and modular designs, have demonstrated the potential of ATPS-derived microgel bioinks to create tailored cellular microenvironments, addressing challenges like oxygen delivery and tissue-specific integration while replicating the complexities of native tissues. This review synthesizes these advancements, critically discussing key considerations, including material selection, physicochemical properties, mechanotransduction, and stress-relaxation behavior. Future directions include advancing multi-scale fabrication techniques, refining cell–material interactions, and addressing scalability challenges to bridge the gap between research and clinical application. By providing a comprehensive perspective on the state-of-the-art in ATPS-derived microgel bioinks, this review emphasizes their potential to transform bioprinting and tissue engineering.

1. Introduction
Biological tissues feature complex, hierarchical structures with heterogeneous layers across varying length scales.1,2 This heterogeneity imparts crucial physicochemical properties, such as stiffness, elasticity, porosity, and biomolecule partitioning, that facilitate the spatial organization of diverse cell types, enabling them to carry out essential physiological functions.3 For instance, human skin consists of multiple layers, each with distinct compositions, protein networks, and mechanical properties, which together protect against external pathogens and respond to mechanical stimuli. On a cellular level, biomacromolecules are organized into membraneless condensates through phase separation, ensuring the proper regulation of intracellular functions and maintaining organelle organization.
Replicating the complex structures of biological tissues across various length scales enhances our understanding of natural systems and enables the development of multifunctional soft materials. These bioinspired materials have broad potential in applications such as studying cell dynamics, creating biocompatible interfaces for monitoring devices, and advancing tissue healing and regeneration.4 However, engineering artificial tissues that recapitulate native structural and functional properties remains a major challenge, requiring the development of advanced fabrication techniques capable of creating biomimetic, cell-laden architectures.
Bioprinting has emerged as a key technology for fabricating engineered tissues, with extrusion-based bioprinting being the most widely adopted approach due to its ability to process a broad range of bioinks with tunable mechanical properties.5,6 However, the high shear stress and pressure exerted during printing can compromise cell viability and function. These mechanical forces activate mechanotransduction pathways, through which cells convert mechanical stimuli into biochemical signals that regulate various cellular behaviors. To mitigate these challenges, extensive research has focused on developing bioinks that minimize shear-induced cell damage while maintaining printability and biological functionality.
Hydrogel-based materials, composed of crosslinked polymers with high water content, are ideal for mimicking biological tissue properties and are extensively used in biomedical fields like tissue engineering, wound care, drug delivery, and soft robotics. However, their limited ability to sustain cell-laden structures under physiologically relevant conditions remains challenging. In response to these limitations, the focus has shifted in recent years toward developing microparticulate inks, which, combined with bioprinting, serve as modular building blocks for fabricating intricate bio-constructs and personalized implants.4,7–9 These microparticles, which include bioactive inorganic particles, particulate autologous tissues, extracellular matrix (ECM)-derived microparticles, and synthetic polymeric microparticles, are incorporated into bioinks to enhance their printability, shape fidelity, and biological performance.8,10 By adjusting the rheological and mechanical properties of the inks, these microparticles can function as culture platforms for cellular expansion or as matrices for cell encapsulation. Furthermore, microparticulate inks exhibit dynamic rheological properties such as shear-thinning and self-healing, making them ideal for extrusion printing and embedded printing techniques.11 These properties open the door to numerous biological and biomedical applications, including cell and drug delivery, tissue engineering, and sensing implants.12
Nature has developed a method for building complex organisms through molecular interactions between different building blocks. Recent advances in cell biology, particularly the discovery of membraneless organelles, show that intracellular environments can be organized via biomolecular condensates formed through aqueous phase separation. This process, driven by interactions between proteins and RNA, is mimicked by aqueous two-phase systems (ATPS), which result from incompatible polymers interacting to create immiscible phases with varying properties like viscosity and surface tension.13 ATPS has been widely used in biomolecule extraction and cell organelle purification. When combined with 3D bioprinting, ATPS enables the creation of cell patterns and vascular structures, allowing selective biomolecule partitioning and compartmentalized reactions.14,15
This review investigates ATPS systems as a biocompatible platform for bioink development, highlighting their unique phase separation properties that enable controlled polymer interactions, maintain interfacial stability, and facilitate biomolecular compartmentalization—key elements in biomimetic tissue engineering. Additionally, we analyze the factors influencing material selection, including polymer composition, molecular weight, interfacial tension, and other physicochemical properties, which contribute to phase stability and tissue customization.
As an extension of ATPS bioinks, we explore the incorporation of microgels, which leverage their two aqueous phases nature to enhance structural integrity, modulate cell–matrix interactions, and improve multifunctionality, particularly in tissue regeneration applications. These microgels improve tissue survival by providing oxygen diffusion and maintaining cell functionality post-bioprinting. Next, we explore fabrication strategies for ATPS-derived microgel bioinks, detailing how polymer selection, microgel synthesis methods, and processing conditions influence microgel size, stability, and mechanical properties. By adjusting the concentration of hydrophilic and hydrophobic phases within ATPS, microgel particle size and void formation can be optimized, creating a microenvironment that supports tissue-specific cells.
We also analyze how mechano-transduction and stress-relaxation behavior impact cell behavior during and post-printing, shaping cell proliferation, migration, and integration into surrounding tissues. Stem cells, for instance, are highly sensitive to shear stress during bioprinting, and these mechanical forces can significantly influence their behavior and, ultimately, the quality of the resulting tissue.16 To address these challenges, ATPS-derived microgel bioinks provide a tunable platform to modulate mechanical properties and reduce shear-induced damage. Optimizing their viscoelasticity and stress relaxation behavior is essential for promoting cell growth, differentiation, and survival while minimizing the negative effects of shear stress. Ensuring that bioprinted constructs exhibit stress-relaxation properties similar to native tissues is also crucial for improving cell migration and tissue integration. Finally, we summarize key insights and future directions, focusing on refining ATPS formulations and advancing multi-scale fabrication strategies to replicate native tissue complexity. This review provides a structured and forward-looking perspective on developing advanced bioinks for tissue engineering.
2. Aqueous two-phase systems and bioprinting
Aqueous two-phase systems are composed of two immiscible aqueous phases that arise when polymers, salts, or surfactants with differing affinities for water coexist in a solution.17,18 This immiscibility is driven by phase separation due to thermodynamic incompatibility between the components, governed by molecular interactions such as steric repulsion, electrostatic forces, and hydrogen bonding, forming distinct liquid–liquid phases with stable water–water interfaces.17,19–21 The physicochemical properties of ATPS, including their low interfacial tension, tunability, and biocompatibility, make them well-suited for bioprinting applications.4,13,14
By adjusting the type and concentration of the components within ATPS, one can achieve control over phase separation behavior, viscosity, mechanical properties, and stability, which is essential for tailoring their performance in bioprinting. ATPS bioinks have been explored as an alternative to emulsion-based bioinks due to their fully aqueous composition. Unlike emulsion-based bioinks that rely on water–oil systems, ATPS bioinks maintain a fully aqueous environment. Emulsion-based bioinks often require surfactants and organic solvents, which, even in small amounts, can affect cell membrane integrity and disrupt crosslinking.13,21 ATPS bioinks eliminate this risk by maintaining a completely aqueous system, enhancing both cytocompatibility and structural uniformity.14,15 Beyond providing a fully aqueous composition, ATPS bioinks use this aqueous–aqueous phase separation as an advantage to create distinct microenvironments within the bioprinted construct, supporting cell viability during and post-extrusion. This biphasic nature enables the encapsulation of living cells, biomolecules, growth factors, and other components within distinct phases, providing enhanced control over the cellular microenvironment.18 Another significant advantage of ATPS bioinks is their tunability in terms of mechanical and porous properties. Modulating polymer concentration, molecular weight, and phase separation kinetics, combined with the low interfacial tension between the aqueous phases, minimizes mechanical stress on cells during bioprinting,15,17 and making ATPS bioinks advantageous for the production of cell–laden bioinks.
Beyond serving as biphasic bioinks, ATPS system bioinks can be selectively structured or stabilized through various mechanisms, including crosslinking, ionic interactions, phase separation-driven structuring, or physical gelation. This selective stabilization allows one phase to be retained, forming microgels, jammed gels, or colloidal gels in situ. The resulting microstructures create interconnected porous networks that improve mass transport and mechanical reinforcement while promoting cellular activity. In contrast to bulk hydrogels, which may restrict diffusion, ATPS-derived microgel bioinks create a dynamic ECM-like environment that enhances cell proliferation, differentiation, and tissue remodeling. Consequently, using the biphasic nature of ATPS system bioinks to generate microgels has emerged as a promising strategy for refining material properties in bioprinting applications.3,22
2.1. ATPS-derived microgel bioinks
ATPS-derived microgel bioinks are built upon the foundational principles of ATPS by introducing microscale structuring to enhance material properties and functionality. Compared to sacrificial templating and freeze-drying, ATPS systems bioinks provide a biphasic environment where phase separation occurs in fully aqueous media, eliminating the need for oil phases or template removal while ensuring homogeneous polymer distribution, high cell viability, and controlled structural integrity.23 This approach simplifies fabrication, enhances biocompatibility, and allows direct microstructuring within bioprinted structures.
ATPS bioinks serve as a precursor system to microgel-based bioinks, upon stabilization, where phase-separated regions can be selectively stabilized or crosslinked post-printing through various mechanisms such as ionic bonding, enzymatic reaction, physical gelation, or photopolymerization.4 Depending on the method used, the resulting ATPS-derived microgels exhibit tunable mechanical properties, viscoelasticity, and microporosity.24 By adjusting ATPS composition and phase separation parameters, it is possible to precisely control microgel size, shape, and spatial distribution, facilitating the fabrication of hierarchically structured bioinks. The interconnected microporosity of ATPS-derived microgels enhances nutrient diffusion, oxygen transport, and cellular migration, addressing key limitations found in bulk hydrogel bioinks.25 Furthermore, ATPS-derived microgel bioinks exhibit shear-thinning behavior, reducing viscosity under shear stress during extrusion but rapidly recovering structural integrity post-printing. This enhances layer-by-layer deposition and prevents collapse, ensuring high-resolution constructs with tunable mechanical properties.
The fabrication of ATPS-derived microgel bioinks depends on the careful consideration of several factors, such as the selection of the polymer component to induce phase separation and control microgel structuring. Hydrophilic polymers such as gelatin and its derivative gelatin methacryloyl (GelMA), as well as polyethylene oxide (PEO), are commonly used to achieve phase separation and form colloidal gels. Gelatin, rich in hydrophilic groups, forms strong hydrogen bonds with water, making it an excellent candidate for aqueous systems. GelMA, a gelatin derivative, introduces slight hydrophobicity due to its methacrylic groups.26 This alteration in hydrophilicity modifies its interaction with PEO, which, although inherently hydrophilic, exhibits reduced hydrophilicity as its molecular weight increases. These thermodynamic differences between polymers drive the phase separation necessary to form the microgels post-crosslinking, enabling control over their size and distribution.
The resulting microgels exhibit a granular structure that enhances mechanical stability and microporosity, making them suitable for complex constructs. These properties allow nutrients and oxygen to move easily, and their small size fits well with detailed 3D bioprinting. Additionally, their modular nature enables incorporating various cell types and bioactive molecules, broadening their applications in tissue engineering. By using the tunable interactions between hydrophilic and slightly hydrophobic polymers, ATPS-derived microgel bioinks address the limitations of bulk-phase systems, such as insufficient structural integrity and reduced resolution. These advances demonstrate the potential of ATPS-derived microgel bioinks to obtain customizable and functional materials for engineering biomimetic tissue constructs.
Figure 1 illustrates the formation and application of ATPS-based microgel bioinks, emphasizing structural and biological characteristics. ATPS formation (Figure 1, top) occurs through phase separation of aqueous polymer solutions, dictated by the binodal curve and interfacial tension properties. This biphasic system enables the generation of ATPS-derived microgel bioinks through different structuring mechanisms, including self-assembly, Pickering emulsions, colloidal stabilization, and microfluidic-assisted crosslinking (Figure 1, bottom-left). Upon extrusion, ATPS-derived microgel bioinks reorganizes into a highly interconnected microporous network (Figure 1, bottom-right). This architecture facilitates nutrient transport, cell infiltration, and dynamic remodeling while maintaining structural fidelity. By leveraging phase separation, ATPS-based bioinks overcome the limitations of traditional jammed microgel bioinks, promoting both printability and enhanced biological activity.
Figure 1. Schematic representation of aqueous two-phase system (ATPS) formation and its application in microgel bioprinting. (Top-left) Mixing two aqueous polymer solutions initiates phase separation, leading to ATPS formation. (Top-middle) The binodal curve determines the polymer concentration threshold for phase separation, distinguishing single-phase from two-phase regions. (Top-right) Interfacial tension varies with polymer concentration, influencing bioink stability and microgel uniformity. (Bottom-left) ATPS-derived microgel bioinks utilize phase separation to generate a structured, microporous network. (Bottom-right) During bioprinting, these bioinks form interconnected microporous networks, enhancing nutrient transport, cell infiltration, and biological activity while preserving structural fidelity. Insets illustrate how encapsulated cells dynamically interact with the surrounding matrix, facilitated by stress relaxation and microgel packing. Adapted under the terms of the Creative Commons CC BY license, Advanced Science, 2022.38 The bottom figures were schematized by the authors.
The structural and functional properties of ATPS-derived microgel bioinks are primarily determined by selecting polymeric components. By carefully selecting polymer pairs with suitable phase separation, the bioprinting performance can be optimized to achieve tunable porosity, mechanical strength, and print fidelity. Section 2.2 examines the role of several polymers in ATPS bioinks, highlighting their advantages, limitations, and influence on biofabrication.
2.2. Polymer selection for ATPS bioinks
In developing ATPS bioinks, the appropriate polymer selection is a fundamental step, as it directly influences phase separation, microgel formation, and overall material properties. Polymers in these systems can be categorized into natural, synthetic, and hybrid types, each offering different properties that enable tailoring bioinks for specific applications.
2.2.1. Natural polymers
Natural polymers are widely used in ATPS system bioinks due to their inherent biocompatibility, biodegradability, and ability to modulate phase separation, making them well-suited for hydrogel structuring and cellular support. Among these polymers, gelatin and alginate are usually used for their capacity to form structurally stable and biocompatible systems.
Gelatin, a collagen derivative, is commonly used in ATPS bioinks due to its thermosensitive gelation properties and ability to form microporous scaffolds that provide structural support for embedded cells, contributing to tissue regeneration and drug delivery.27 Similarly, alginate, an anionic polysaccharide derived from brown algae, exhibits strong gelation characteristics through ionic crosslinking, creating mechanically stable and highly porous hydrogel networks when exposed to divalent cations such as Ca2+.28
In ATPS bioinks, natural polymers enhance biocompatibility and influence phase separation, forming interconnected microporous structures that improve cellular interactions and nutrient diffusion. However, while natural polymers provide structural support and tunable physicochemical properties, they often exhibit weak mechanical properties and rapid degradation under physiological conditions requiring chemical modifications or reinforcement with synthetic materials to improve mechanical integrity.29,30
2.2.2. Synthetic polymers
Synthetic polymers, such as PEO and polyethylene glycol (PEG), provide tunable mechanical and rheological properties, enabling control over molecular weight, hydrophilicity, and phase behavior. Their role in creating stable ATPS systems has been well-documented in several studies where they modulate phase separation and the physical and mechanical properties of bioinks.31,32 These polymers allow researchers to tailor crosslinking density, degradation kinetics, stiffness, and stability for diverse bioprinting applications. However, despite their mechanical advantages, synthetic polymers often lack biological activity, meaning they do not naturally support cell adhesion or tissue integration, requiring a combination with natural polymers to promote cellular interaction and tissue incorporation.
2.2.3. Hybrid polymers
Hybrid polymer systems, such as GelMA/dextran32 and GelMA/PEO,14 combine the biocompatibility of natural polymers with the mechanical properties of synthetic materials. These systems have been well-used to obtain hydrogels with enhanced functionality, characterized by tailored porosity, optimized mechanical properties, and controlled degradation kinetics, which make them suitable for applications requiring dynamic cell–material interactions. Despite their advantages, hybrid polymers present formulation challenges. First, the ratio of natural to synthetic components must be carefully optimized to balance phase separation, modulate gelation kinetics, and preserve cell viability. Second, crosslinking parameters, such as reaction time, photoinitiator concentration, and light wavelength, must be tuned to ensure mechanical properties without compromising biocompatibility.
Figure 2 illustrates an overview of ATPS bioinks applications, highlighting their role in tissue engineering and the biomedical field.
Figure 2. An overview of the representative applications of aqueous two-phase systems bioinks.
Building on the categorization shown in Figure 2, Table 1 summarizes the diverse range of polymers utilized in ATPS bioinks, detailing their selection based on application, cell type, and required mechanical properties. Additionally, it provides an overview of the advantages and limitations of each polymer system, offering insights into their suitability for specific biomedical applications. This comprehensive summary highlights the versatility of ATPS bioinks in addressing a wide range of research and clinical applications.
Table 1. Summary of polymers used in ATPS bioinks, detailing their associated cell types, biomedical applications, advantages, and limitations
Polymer | Cell type | Applications | Advantages | Disadvantages | Ref. |
---|---|---|---|---|---|
ACMMA, GelMA, PEO, and PCL | Auricular chondrocytes | Auricular cartilage regeneration | Promotes cartilage-specific ECM deposition | Hard to achieve mechanical stability | 29 |
Type I collagen, PEG, dextran | HEK 293, NIH 3T3, MC 3T3, MCF10A | ECM remodeling, wound healing, drug testing | Supports high cell viability | Phase instability over long durations | 31 |
Alginate, PEG, and dextran | HeLa and HepG2 | Tissue engineering and drug delivery vehicles | Promotes high cell proliferation and metabolic activity | Phase separation parameters need optimization | 28 |
GelMA and gelatin norbornene | Bone marrow-derived MSCs | Tissue engineering | Supports long-term cell viability | Complex photopolymerization control | 33 |
GelMA and PEO | hMSCs | Wound and tissue repair | Enhances macroporosity for cell growth proliferation | Injection stress requires optimization | 27 |
GelMA, dextran, and pCNF | MC3T3-E1 | Bone tissue regeneration | Stabilizes emulsion and supports biomineralization | Flocculation of pCNF in physiological conditions | 32 |
GelMA and dextran, stabilized by β-lactoglobulin nanoparticles | NIH/3T3, C2C12, SK-Hep-1 | Cartilage tissue | Enhances macroporosity for cell growth and mechanical properties | Emulsification stability requires adjustment | 34 |
GelMA, dextran, and GGMMA | NHDFs, MC3T3-E1 | Tissue engineering | Improves emulsion stability and supports cell viability | Crosslinking conditions require adjustment | 3 |
GelMA and PEO | NIH/3T3, HUVECs, HepG2 cells | Tissue engineering and drug screening | Improves cell spreading and proliferation | Requires optimized crosslinking kinetics | 14 |
PEO and dextran | NIH/3T3 | Tissue engineering | Supports freeform printing and multi-compartment tissue models | Colloidal stability | 35 |
PNIPAM and dextran | Cell-free | Tissue engineering | Thermoresponsive properties allow controlled phase separation | Careful temperature control | 36 |
PLL, oxBC | ECs | Vascularized tissue constructs and drug delivery | Supports long-term cell viability | Polymer ratio and concentration require optimization | 37 |
PEG, dextran, and alginate | NIH/3T3 | Tissue engineering | Allows printing at high extrusion speeds while maintaining cell viability | Polymer ratio and concentration require optimization | 38 |
Poly(acrylic acid) bottlebrush polymers and linear poly(allylamine hydrochloride) | Cell-free | Tubular structure | High stability under varying salt/pH conditions | Weak polyelectrolyte interactions | 39 |
Gelatin and maltodextrin | Cell-free | Microgel carriers for enzyme delivery in food industry | Biocompatible, enzyme protection and stability | Phase separation parameters need optimization | 40 |
Dextran and PEO | HepG2, HUVECs | Vascularized tissue engineering, drug testing, and tissue engineering | Enhances angiogenesis without external VEGF | Control of cell ratio for optimal vascularization | 41 |
PEG and dextran | Cell-free | Drug delivery and encapsulation of biomolecules with controlled release properties | Controlled permeability | Requires optimized crosslinking kinetics | 42 |
Alginate, dextran, and PEG | Caco-2, HepG2 | 3D cell coculture systems for studying inter-tissue interactions and developing disease models | Supports high cell viability | Requires optimized crosslinking kinetics | 43 |
GelMA and PEG | HepG2 | Tissue engineering for regenerative therapy and drug development | Encapsulates cells without damage | Polymer ratio and concentration require optimization | 44 |
PEG and dextran | C2C12 | Engineering stem cell niches for neuronal differentiation and creating heterocellular constructs | Supports high cell viability | Phase instability over time | 45 |
Fibrinogen, PEG, and dextran | NHLF, IFP | Tissue engineering | Controlled fibrin polymerization | Optimization of polymer ratio | 46 |
PEG and dextran | hFB | Drug release and tissue engineering | Tunable release kinetics and high biocompatibility | Photopolymerization control | 47 |
Alginate, collagen, and Matrigel | HA, HBVP, HBMEC | Development of 3D micro-tissues | Supports blood-brain barrier modeling in vitro | Careful temperature and viscosity control | 1 |
PEG, dextran, polyacrylamide | HUVECs | Wound dressing, healing, and tissue engineering applications | Supports biomimetic tissue construction | Phase separation parameters need optimization | 48 |
PAM and alginate with functionalized chemical groups | β-TC-6 | Tissue engineering and responsive drug delivery scaffolds | Tailored mechanical properties via double-network hydrogel | Crosslinking uniformity needs improvement | 49 |
GelMA and PEO | NIH/3T3, HUVECs | Cannular tissue models | Maintains high cell viability | Crosslinking conditions require adjustment | 50 |
GelMA and dextran loaded with AgGNRs | MSCs | Tissue engineering | Enhances antibacterial and immunomodulatory properties | Risks of nanoparticle cytotoxicity at high concentrations | 51 |
GelMA and dextran | ADSCs | Drug screening, tissue engineering, and disease modeling | Allows microporous structures for enhanced cell migration | Polymer ratio requires optimization for pore size regulation | 52 |
GelMA and GelTA | hMSCs | Vessel regeneration, ischemic tissue repair, and muscle regeneration | Oxygenating and cell-tethering hydrogels | Complex fabrication and material synthesis | 53 |
Gelatin, GelMA, and PEO | hMSCs | Engineering of biomimetic tissues with enhanced oxygenation for regeneration of complex tissue constructs | Supports cell differentiation through targeted oxygen delivery | Careful control of oxygen release | 26 |
GelMA and PEO | NIH/3T3, HepG2 | Tissue engineering, regenerative medicine, and pharmaceutical screening | Highly tunable microporosity and improved emulsion stability | Emulsion phase separation can affect structure integrity | 22 |
Abbreviations: ACMMA, methacrylated acellular cartilage matrix; ADSCs: Adipose-derived stem cells, adipose derived-stem cells; AgGNRs, silver-coated gold-nanorods; ECM, extracellular matrix; ECs, endothelial cells; GelMA, gelatin methacryloyl; GelTA, tyramine-conjugated gelatin; GGMMA, methacrylated galactoglucomannan; HA, hyaluronic acid; HBMEC, human brain microvascular endothelial cells; HBVP, human brain vascular pericytes; hFB, human fibroblasts; HUVECs, human umbilical vein endothelial cells; IFP, idiopathic pulmonary fibrosis fibroblasts; MSCs, mesenchymal stem cells; NHDFs, normal human dermal fibroblasts; NHLF, normal human lung fibroblast; oxBC, oxidized bacterial cellulose; PAM, polyacrylamide; PCL, polycaprolactone; pCNF, phosphorylated cellulose nanofibrils; PEO, polyethylene oxide; PLL, polylysine; PNIPAM, poly(N-isopropylacrylamide); VEGF, vascular endothelial growth factor.
As shown in Table 1, ATPS bioinks present several advantages, such as biocompatibility, tunable porosity, and improved mechanical properties, making them highly versatile for tissue engineering. However, challenges such as phase separation control, polymer crosslinking, and emulsion stability must be addressed to optimize their potential. Several studies have explored strategies to refine these properties by tailoring polymer ratios, compositions, and concentrations, as well by adjusting crosslinking conditions and emulsification techniques.
Ying et al.14 explain that in ATPS bioinks, one aqueous phase (such as GelMA) forms a polymer network, whereas the other phase (like PEO) can be removed after crosslinking to form pores within the hydrogel. This method facilitates the development of highly porous structures that enhance cell proliferation, oxygen diffusion, and nutrient transport, being suitable for tissue engineering. Building upon this phase-separation technique, Wang et al.32 used a similar ATPS-based emulsion using GelMA and dextran. In this system, GelMA serves as the continuous phase and dextran forms droplets. After crosslinking, dextran phase leaches out, producing a network of interconnected pores.
Predominantly, researchers have developed macroporous hydrogels using GelMA and PEO in ATPS.22,54 This system enables the fabrication of interconnected porous structures that promote cell proliferation and differentiation. Adding biosurfactants, such as rhamnolipids, improves the emulsion stability, allowing the bioink to maintain performance over prolonged periods during 3D bioprinting.55 Another notable example of ATPS is a biphasic hydrogel bioink developed using PEG microparticles embedded in a continuous GelMA-nanosilicate colloidal network.56 This biphasic structure allows for the precise patterning of cells and enhances tissue engineering capabilities. The ATPS system in this bioink contributes to its mechanical stability and improves cell viability, enabling its use in complex 3D tissue bioprinting.
In summary, polymer selection in ATPS bioinks is essential for shaping the mechanical, biological, and functional properties of the resulting structures. The combination of natural, synthetic, and hybrid polymers presents a variety of options for developing tailored systems that support advanced tissue engineering and bioprinting applications. By combining polymers with high biocompatibility, controlled phase separation, and improved mechanical stability, ATPS bioinks continue to expand the possibilities for biofabrication.
2.3. Fabrication methods for ATPS-derived microgel bioinks
Depending on the intended application, ATPS bioinks can be used directly as biphasic bioinks or further structured into ATPS-derived microgels. After selecting polymer components, the fabrication method also significantly influences the microstructure, porosity, and stability of ATPS-derived microgel bioinks. Different fabrication techniques modulate size distribution, reproducibility, and phase separation behavior. The most used methods include batch emulsion processing, microfluidics, phase-separation-driven techniques, and Pickering emulsions, each one offering distinct advantages and challenges depending on polymer properties, emulsification parameters, and crosslinking mechanisms.
Among the available fabrication methods, the batch emulsion process is widely used due to its simplicity, scalability, and compatibility with diverse polymer systems.3,22 This method involves the mechanical mixing of two immiscible aqueous phases to generate droplet-stabilized emulsions, which act as templates for microporous hydrogel formation. During the batch emulsion process, polymers such as GelMA, PEO, or dextran are phase-separated into droplets within a continuous aqueous phase.14,26,38 Stabilizers, such as biosurfactants, are often added to improve emulsion stability and minimize droplet coalescence. Once stabilized, the droplets are crosslinked to fix their structure, and the dispersed phase is selectively removed, resulting in interconnected porous networks with tunable pore size and mechanical properties.34 While this method allows rapid fabrication and adaptability, it also produces polydisperse microgels, leading to inconsistent porosity and variable mechanical properties.2,57 Optimizing shear stress, surfactant concentration, and polymer phase ratios to address these limitations can mitigate polydispersity, improving uniformity and printability.
Although batch emulsification is scalable, achieving uniform microgel distribution remains a challenge. In contrast, microfluidic techniques control droplet formation, ensuring monodisperse microgel production and reproducibility. This technique uses microchannel networks to manipulate two immiscible aqueous phases, where shear forces govern droplet formation and phase separation. Common microfluidic configurations include T-junctions, co-flow, and flow-focusing devices, which facilitate the creation of monodisperse droplets with adjustable sizes and phase compositions. Depending on flow rate ratios, polymer concentration, and microchannel geometry, these systems can produce microgels ranging from submicrons to several hundred microns. Microfluidic-generated microgels show high packing density, optimizing shear-thinning behavior and mechanical properties. This technique also allows obtaining hybrid bioinks by compartmentalizing different polymeric phases, enabling control over phase separation for encapsulating single cells or cell clusters in tunable aqueous compartments.58,59
Despite their advantages, microfluidic fabrication faces scalability challenges, as most systems function at a lower throughput than batch emulsion, limiting the volume of bioinks that can be produced. To address this, parallelized microfluidic arrays and continuous-flow systems have been explored to increase output. However, device fabrication and operational tuning require precise control over the flow rates and interfacial forces, adding complexity to the method.60
In Pickering emulsions, solid particles stabilize ATPS droplets by forming a protective layer at the interface that prevents coalescence and enhances stability.3 Unlike conventional surfactant-stabilized emulsions, this method relies on colloidal stabilizers, such as silica nanoparticles or protein-based stabilizers, which absorb at the droplet interface to create a physical barrier. This method enables the formation of highly stable microgels with controlled size and extended stability, making it particularly effective for low interfacial tension systems like PEG-dextran ATPS.61 By adjusting particle size, concentration, and surface properties, microgel characteristics can be tuned for bioprinting applications.3 However, careful optimization is needed to balance mechanical stability and flow properties, ensuring consistent extrusion performance
ATPS-derived microgels can also be formed by self-assembly mechanisms driven through phase separation. In this approach, thermodynamic incompatibility between two aqueous polymer phases leads to spontaneous droplet formation. The polymer ratio and their molecular weights determine droplet size. Higher molecular weight polymers generally form smaller, more stable microgels, while lower molecular weight polymers producelarger, more deformable droplets.1,36,62 This method allows for tunable microgel structures, where adjusting polymer concentration, molecular weight, temperature, or pH enables the fabrication of bioinks with customizable porosity and mechanical properties suited for diverse tissue engineering applications.1 Encapsulation of bioactive agents and cells within these microgels enhances bioprinting precision and functionality. However, reproducibility and size control remain challenges, as phase separation dynamics depend on polymer interactions. Without careful optimization, undesired coarsening or aggregation may occur. Moreover, the stability of phase-separated microgels in bioprinting environments requires attention, as they may gradually coalesce or fuse over time.
2.3.1. Size distribution and polydispersity: Factors affecting the size of ATPS microgels
As mentioned in Section 2.3, the choice of fabrication method plays a crucial role in determining the final properties of ATPS-derived microgel bioinks. While each technique offers distinct advantages and limitations, microgel size distribution and polydispersity are among the most critical aspects influenced by fabrication parameters. The uniformity of microgel particle size influences porosity, mechanical stability,22,63 and biocompatibility,64 while polydispersity impacts the uniformity and reproducibility22,62,65 which can introduce inconsistences that impact on extrusion-based bioprinting.
Batch emulsion produces polydisperse microgels ranging from ~1 to 1000 μm due to the random nature of droplet formation. This method tends to produce more polydisperse microgels, but their limitation can be mitigated by optimizing emulsification parameters such as shear stress, surfactant concentration, and polymer phase ratios. For example, Widener et al.57 demonstrated that increasing shear stress during emulsification reduces microgel size, generating microgels as small as 5–30 µm, while lowering shear stress leads to larger, more polydisperse particles (~50–200 µm). Similarly, Qin et al.55 showed that using biosurfactants like rhamnolipids improves emulsion stability, minimizing droplet coalescence, and promoting uniformity in droplet size. Optimizing these key factors enables monodisperse droplets, enhancing scaffold porosity, cell spreading, and overall functionality for tissue engineering and bioprinting applications.4,8
Microfluidics generates highly monodisperse microgels, typically ranging from ~10 to 500 μm, with precise size control achieved through flow rate adjustments, interfacial tension modulation, and microchannel geometry design.19 This method ensures low polydispersity, with some systems achieving a coefficient of variation (CV) of 1–2%, enabling highly uniform microgel production. Keller et al.66 reached microgels in the 10–100 μm range through droplet microfluidics, achieving tight size distributions that enhance scaffold uniformity and packing density. Similarly, He et al.43 fabricated multicompartmental microcarriers ranging from 150 to 300 μm using a co-flow microfluidic approach, allowing for precise control over microgel structure and internal compartmentalization. These microfluidic systems provide a uniform particle size distribution, enabling tighter packing and homogeneous structures in granular bioinks.3,13 Phase separation-based ATPS microgels exhibit a broad size range from ~10 to 200 μm, depending on polymer concentration, molecular weight, and phase incompatibility.1,62 Beldengrün et al.40 reported that gelatin-maltodextrin emulsions stabilized via phase separation yielded microgels ranging from 5 to 25 μm, with size distribution controlled by stirring speed and emulsification temperature. By adjusting polymer concentration, molecular weight, and stirring conditions, phase separation-based ATPS microgels can be tailored for precise size control, optimizing their use in biomaterial formulations and tissue engineering.
Pickering emulsion ATPS microgels range from ~20 to 300 μm, with droplet size controlled by nanoparticle concentration, interfacial adsorption, and agitation speed.67,68 Qian et al.69 showed that dextran/PEG ATPS emulsions stabilized by starch cellulose nanocrystals (sCNC) produced microgels ranging from 20 to 200 μm, with higher sCNC concentrations yielding smaller (~20–50 μm) and more stable droplets, whereas lower concentrations resulted in larger (~100–200 μm) and less stable microgels. Similarly, Peddireddy et al.70 demonstrated that CNCs stabilize ATPS emulsions, forming dextran/PEO microgels with sizes ranging from 10 to 100 μm, where higher CNC concentrations reduced droplet size and improved emulsion stability. The choice of stabilizing particles, their surface properties, and their interaction with polymer phases directly influence microgel size, providing tunability for biomaterial applications requiring precise droplet control.
As discussed, different fabrication methods generate microgels with distinct size distributions, influenced not only by the chosen technique but also by multiple factors such as polymer composition and concentration, viscosity, emulsification parameters, shear stress, and mixing speed. These key variables should be carefully considered as they significantly affect droplet stability, size, and distribution.17,19,64
Polymer concentration and molecular weight directly impact droplet formation, stability, and size uniformity. Higher polymer concentration and molecular weights increase viscosity, which improves interfacial stability. This results in smaller, uniform microgels due to less droplet deformation under shear forces. In contrast, low-viscosity systems facilitate droplet deformation, producing larger microgels and a broader size distribution.64
Beyond influencing microgel size uniformity, the balance between hydrophilic and hydrophobic polymer concentrations further determines morphology and void formations.17,71 Hydrophilic polymers, such as PEG and dextran, stabilize smaller droplets by increasing viscosity and reducing coalescence.44,60 The ultralow interfacial tension combined with the biocompatibility of PEG-dextran systems makes them suitable candidates in bioprinting applications requiring high fidelity, such as neural or vascularized tissues.17 Higher concentrations of hydrophilic polymers reduce interfacial tension, resulting in smaller, more uniform microgel particles.17,62 In contrast, hydrophobic polymers like GelMA promote larger droplets by altering interfacial tension, which governs droplet morphology.63,72 Increasing concentrations of hydrophobic polymers increase interfacial tension, producing larger microgel particles with more polydispersity and, consequently, larger void spaces, which are ideal for cartilage or adipose tissue engineering.73
Adjusting polymer concentrations, phase ratios, and processing conditions, ATPS systems offer control over microgel size and produce monodisperse particles suitable for bioprinting applications, where uniform particle size enhances extrusion performance and scaffold reproducibility.
Further illustrating the role of polymer interactions in microgel formations, Yi et al.22 demonstrated that GelMA when combined with different porogens, such as PEO, polyvinyl alcohol (PVA), and dextran, produces distinct size distributions influenced by molecular interactions with GelMA, underscoring how formulation choices affect final properties. GelMA/PEO systems typically produce microgels with sizes between 7 and 60 µm, influenced by PEO molecular weight, concentration, and applied shear forces during mixing.22 GelMA/PVA systems yield pores between 10 and 50 µm, while GelMA/dextran combinations generate smaller micropores, ranging from 3 to 20 µm, depending on porogen properties and polymer ratios. Smaller, more uniform microgels benefit applications requiring dense cell packing, such as vascularized tissues,62,72 while larger particles create void spaces suitable for cartilage engineering.63
External parameters such as temperature and ionic strength also modulate phase separation behavior. Ahmed et al.71 analyzed the effect of temperature and ionic strength on phase separation, showing that droplet morphology and size distribution can be tuned by adjusting these parameters. Similarly, Keller et al.66 emphasized the role of interfacial tension in governing droplet morphology, reinforcing the need for balanced polymer ratios to achieve stable and monodisperse microgels. Ying et al.14 demonstrated that reducing interfacial tension between polymer-rich and polymer-poor phases results in smaller, more stable droplets, improving bioprinting fidelity. They found that GelMA/PEO systems enable the formation of uniform microgels without surfactants due to their ultralow interfacial tension and thermodynamic incompatibility. The stability and size of droplets can be controlled by tuning the volume ratio of GelMA to PEO, which directly affects phase separation. A higher GelMA-to-PEO ratio results in smaller, more uniform droplets (~22.7 ± 5.5 µm), whereas a lower ratio leads to larger, less stable droplets (~52.7 ± 17.7 µm). Furthermore, at low concentrations of PEO, phase separation is weak, resulting in irregular droplet formation, while at higher concentrations, well-defined and stable droplet form, highlighting the role of PEO concentration in droplet stabilization. By adjusting polymer ratio and phase affinity, the final microgel morphology can be tuned, impacting mechanical integrity and print resolution while also influencing microporosity, nutrient diffusion, and cell viability in 3D-bioprinted constructs.
While interfacial tension, polymer concentration, and phase affinity influence microgel formation, mixing parameters are also crucial in controlling droplet size and stability. The impact of shear forces on emulsification is particularly evident in batch emulsion methods, where optimizing mixing speed can significantly improve size uniformity. Muir et al.59 reported that optimizing these parameters is essential for achieving uniform droplet size and ensuring comparability among microgels prepared by different techniques. Microgels produced via the bath emulsion process at 350 rpm showed a size distribution similar to those obtained by the microfluidic method, while microgels fabricated at 250 rpm maintained an average diameter of ~100 μm but with a broader size distribution ranging from ~20 to 200 μm. Mixing speed affects shear forces and droplet breakup efficiency. At lower agitation rates, larger and more polydisperse droplets form due to decreased shear stress, while higher mixing speeds promote droplet fragmentation, leading to smaller microgels but with increased size variability. This relationship between mixing speed and droplet size distribution is illustrated in Figure 3, which demonstrates how increased mixing speed promotes uniform microgel formation by enhancing droplet fragmentation and reducing polydispersity.
Figure 3. Influence of mixing speed on microgel uniformity. The schematic shows how higher mixing speed during microgel fabrication reduces polydispersity, facilitating the transition of polydisperse to monodisperse droplets.
Achieving uniform microgel sizes offers significant advantages for bioprinting applications, as it reduces nozzle clogging and ensures consistent extrusion during bioprinting. Granular hydrogels with tightly packed, uniform microgels exhibit superior shear-thinning and self-healing properties, facilitating smoother extrusion and precise deposition of bioink layers.74 Moreover, the intrinsic porosity of these granular bioinks supports cell infiltration and growth post-printing, bridging the gap between structural stability and biological functionality.4
2.3.2. Particle-to-volume fraction: Effect on space availability for cells and tissue formation
Beyond size distribution and uniformity, particle-to-volume fraction (PVF) is another variable influencing the functionality of ATPS bioink. PVF defines the proportion of microgel particles within the total bioink volume, which governs the void space available for cellular activities such as migration, proliferation, and tissue formation.3,27,34,37 Higher PVF values increase scaffold density, improving mechanical stability and supporting extrusion processes. However, excessive PVF can reduce void spaces, limiting nutrient diffusion and cell infiltration. Conversely, lower PVF values enhance porosity, promoting cell migration and interconnectivity but potentially compromising the mechanical integrity of the bioink scaffold.27 Pereira and Coutinho75 demonstrated that low PVF increases permeability in ATPS systems and enhances cellular functionality by increasing void space, which improves nutrient and oxygen diffusion required for cell survival and migration. Figure 4 shows how PVF directly affects the spatial arrangement of microgels and the availability of space for cells in bioprinted structures. Lower PVF results in irregular microgel packing, creating excess voids that restrict direct cell-cell interactions. Conversely, higher PVF results in more uniform packing, promoting stable cell adhesion and supporting functional tissue formation.
Figure 4. Influence of varying PVF on microgel packing and space availability for cells. Lower PVF results in irregular packing, increased void spaces, and limited cell spreading, while higher PVF promotes uniform microgel arrangement, enhancing structural integrity and facilitating improved cell distribution. Abbreviation: PVF, particle-to-volume fraction.
Optimizing PVF is key to tailoring bioinks for specific applications, as it influences both structural and biological outcomes.19,36 For instance, Keller et al.66 showed that adjusting phase ratios during emulsification can tune PVF to balance porosity and mechanical properties, enhancing cell viability and scaffold functionality. Similarly, Qazi et al.76 reported that modulation of PVF affects cell infiltration and the spatial arrangement within hydrogel constructs.
In vascularized tissue engineering, lower PVF supports the integration of endothelial cells and promotes capillary network formation by increasing porosity and facilitating cell migration.8,71 Conversely, in soft tissue engineering applications,38,46 higher PVF values contribute to scaffold integrity by providing a dense microgel matrix that supports cellular adhesion and structural stability.77 Achieving the desired PVF requires careful control of microgel size, packing efficiency, and polymer concentration. Sacrificial phases like PEO or dextran can be incorporated to create controlled void spaces, further enhancing cellular interactions.36,47,70 By leveraging phase separation dynamics and interfacial tension, PVF can be tuned to balance porosity, scaffold stability, and cellular functionality, addressing diverse requirements in tissue engineering.75,78
In extrusion-based bioprinting, PVF influences cellladen scaffold formation and directly affects print fidelity and rheological behavior. Granular bioinks with tunable microgel compositions exhibit shear-thinning behavior and self-healing properties, which are essential for maintaining structural integrity, as noted by Riley et al.8 emphasizing their potential in creating tissue-engineered constructs with complex geometries.
2.4. Extrusion-based bioprinting of ATPS microgels: Physical and rheological requirements
As discussed in previous sections, diverse parameters define the fundamental characteristics of ATPS microgels, influencing their structural and functional properties. Moreover, microgels must also meet several physical and biological requirements to ensure accurate printing and cell viability throughout and after the bioprinting process.62,72 Key parameters, including temperature, viscosity, shear-thinning, viscoelastic behavior, and microgel size, directly influence the stability, mechanical properties, and biological functionality of bioprinted construct.17,63,65 Together, these parameters determine both immediate print fidelity and long-term stability. To systematically address these requirements, ATPS-microgel bioinks can be categorized into three stages: pre-printing, printing, and post-printing. Each stage presents distinct challenges that must be optimized to balance printability, cell viability, and structural integrity.
2.4.1. Pre-printing conditions
The pre-printing stage focuses on preparing the bioink under suitable physical and environmental conditions to ensure phase separation and cell viability. Rogers et al.79 discussed how the phase separation in the ATPS is highly temperature-dependent. Phase separation occurs within a narrow temperature range (18–26 °C), where molecular mobility supports the formation of distinct aqueous phases. At higher temperatures, molecular mobility facilitates smooth phase separation, ensuring the bioink remains fluid and printable, while lower temperatures (~18 °C) induce gelation, stabilizing the bioink structure but limiting fluidity. This gel-like network is advantageous for stabilizing microgels before printing and is beneficial for maintaining the structure of printed constructs. However, maintaining phase separation within a narrow temperature range introduces challenges for reproducibility and scalability as small fluctuations in temperature can alter polymer interactions, affecting phase separation kinetics and microgel uniformity. In gelatin-based ATPS bioinks, temperature control is even more critical to maintaining stability, as GelMA exhibits thermoresponsive sol–gel behavior, transitioning from a gel state at lower temperatures to a sol state as temperature increases. At 4°C, GelMA bioinks adopt a solid-like gel behavior due to the formation of triple-helix structures.14,80 This state provides suitable viscosity for droplet formation, ensuring stability of the bioink and supporting consistent phase separation in ATPS. Moreover, this solid-like state minimizes premature flow and ensures structural reproducibility during bioink preparation.32
As the temperature rises to 25–35 °C, GelMA undergoes a sol–gel transition, where the triple-helix structures disassemble, and the bioink transitions into a liquid-like sol state.14,81 This transition increases molecular mobility, improving fluidity and enabling smooth extrusion during printing. The controlled transition between gel and sol states directly impacts the phase separation process, determining the viscoelastic properties before extrusion.34
Optimizing phase separation during bioink preparation is essential for achieving the desired viscoelastic properties before printing. The stability of ATPS directly affects microgel packing, polymer interactions, and mechanical integrity upon extrusion. Proper polymer ratios regulate phase separation, influencing the storage modulus (G′), which dictates initial microgel stability before extrusion, preventing premature phase dissolution or microgel aggregation.
2.4.2. During printing
To ensure successful printing, bioinks must exhibit dynamic viscosity changes to accommodate extrusion and structural integrity. In contrast to Newtonian fluids, which maintain viscosity regardless of the shear rate, bioinks need to exhibit shear-thinning behavior to enhance flow dynamics during the printing process.23 At lower shear rates (0.1–1 s−1), high viscosity (>100 Pa·s) is needed to maintain stability in the printed layers, preventing deformation and collapse. Conversely, viscosity must decrease as the shear rate increases (10–1000 s−1) to allow smooth bioink flow through the nozzle while preventing excessive extrusion pressure.
Shear-thinning behavior is fundamental to the printing process, preventing needle clogging and reducing extrusion pressures during printing. However, depending on the printing parameters and extrusion conditions, excessive shear stress can still occur, negatively impacting cell viability. Zhang et al.82 noted that using narrow nozzles enhances print resolution, but they can also lead to higher shear stress, which can negatively impact cell viability by damaging cell membranes, decreasing viability, and altering cell behavior. Shear stresses exceeding 487.5 Pa during extrusion result in mechanical damage to cells, mainly in primary mammalian cells, leading to reduced viability and altering their morphology. As illustrated in Figure 5A, extrusion pressure drives bioink flow through the nozzle, leading to microgels rearrangement due to shear-thinning. This dynamic behavior ensures controlled flow and facilitates filament formation and structural fidelity.
Figure 5. ATPS bioink network during bioprinting and its effects on shear stress and cell viability. (A) Schematic representation of the ATPS bioink under extrusion process, showing how shear-thinning behavior facilitates smooth filament formation and prevents nozzle clogging by rearrangement of microgel network structure under applied pressure. (B) Influence of low-viscosity bioinks on shear stress distribution and cell viability. Low-viscosity bioinks generate minimal shear stress, reducing mechanical damage to cells, even at higher flow rates (1000 μL/min) where green-stained live cells dominate, indicating enhanced viability. Scale bar: 250 µm. (C) Impact of high-viscosity bioinks on shear stress and cellular response. High viscosity increases shear forces near the nozzle wall, leading to high cell stress and lower viability where red-stained dead cells and stressed cells are prevalent, demonstrating the adverse effects of excessive shear stress on cell function. These results highlight the importance of tailoring bioink rheology to optimize printability while preserving cell viability. Scale bar: 250 µm. Reproduced under the terms of the Creative Commons CC BY license, Advanced Science, 2022.38
Viscosity also influences resolution and printing fidelity. Bioinks with low viscosity extrude easily but often result in poorly defined droplets or filaments, leading to low-resolution printed structures. Low-viscosity bioinks (<30 mPa s) lack the necessary stability to retain their shape after extrusion, resulting in collapse under their weight.83 In contrast, overly viscous bioinks (>1000 Pa·s)24,84 can clog the nozzle, hindering the smooth extrusion and requiring higher extrusion pressures. The impact of viscosity on shear stress and cell survival is shown in Figure 5B and C, where low-viscosity bioinks promote higher cell survival, even at increased flow rates (1000 μL/min), as lower shear stress minimizes mechanical damage. Conversely, high-viscosity bioinks introduce higher shear forces, since slower flow speeds (50 μL/min) lead to increased cell stress and decreased viability.
To minimize the adverse effects of shear stress on cells, tailoring the rheological properties of microgels can mitigate excessive shear stress and enhance cell viability during printing. Different cell types exhibit varying tolerance levels to shear stress. Stem cells are more sensitive to mechanical damage than cancer cells, lacking robust adaptive mechanisms to tolerate such forces. When exposed to excessive shear stress, stem cells experience membrane rupture, apoptosis, or necrosis.82,85 Optimizing nozzle size and extrusion pressures minimizes these stresses, protecting sensitive cells and ensuring functional viability post-printing.
Besides viscosity and shear-thinning behavior, viscoelastic properties are also fundamental to obtaining continuous filaments, adequate resolution, and printing fidelity after extrusion. The viscoelastic properties of ATPS microgels, defined by the storage modulus (G′) and loss modulus (G″), govern the transition between fluid-like and solid-like behavior, which enables the bioink to recover and maintain structural integrity after printing. Moon et al.86 demonstrated that jammed microgel-based bioinks exhibit balanced viscoelastic behavior, ensuring structural integrity after printing. These inks quickly stabilized after deposition, showing their suitability for bioprinting processes. Similarly, Kessler et al.87 showed that reducing microgel size can significantly increase the storage modulus, enhancing stiffness and ensuring structural fidelity during tissue maturation.
2.4.3. Post-printing
Beyond structural stability, the viscoelastic properties of the bioink impact cellular sensing and adaptation to their microenvironment. The dynamic nature of viscoelasticity integrates multiple factors, including stress relaxation, which complements structural stability by enabling the bioink to adjust to cellular activity. According to Ma et al.88 the dynamic viscoelasticity of the bioink mimics the behavior of the ECM, supporting cell spreading, migration, proliferation, and differentiation, contributing to enhanced tissue development. The ability to tailor G′ and G″ through polymer concentration, molecular weight, and phase separation provides opportunities to engineer bioinks that mimic the dynamic properties of the ECM, promoting natural cellular functions.
Stress relaxation is a crucial post-printing factor that influences cellular adaptation and structural stability. It refers to the gradual reduction of internal stress in bioinks under constant strain that impacts cell–matrix interactions and the overall functionality of bioprinted constructs. After printing, the cellular microenvironment is subjected to mechanical stresses as cells adhere, migrate, and remodel the surrounding matrix. Stress relaxation allows the construct to accommodate these forces while maintaining its intended architecture, as illustrated in Figure 6. Faster stress relaxation rates facilitate these processes by reducing resistance to deformation, allowing cells to spread, elongate, and proliferate effectively. For example, Chaudhuri et al.89 showed that stress relaxation directly impacts stem cell differentiation, with faster relaxation rates promoting osteogenic or adipogenic lineage specification depending on the application.
Figure 6. Post-printing structural evolution and the role of stress relaxation in ATPS bioinks. After deposition, the printed construct undergoes crosslinking to stabilize the microgel network while maintaining structural integrity. During early stress relaxation, cells within the microgel matrix experience a gradual reduction in internal stress, facilitating their spreading, elongation, and adaptation to the surrounding microenvironment. Over time, stress relaxation supports extensive cell–matrix remodeling, promoting cell proliferation, extracellular matrix deposition, and integration into a stabilized tissuelike structure.
In addition to its effects on cell behavior, stress relaxation is conducive to void formation and network remodeling.90 As cells proliferate and secrete matrix proteins, the ability of the bioink in stress relaxation enables the construct to accommodate cellular growth without compromising structural properties. Excessive tension within the printed structure could lead to mechanical failure or inhibit proper tissue development without sufficient stress relaxation. This dynamic adjustment supports cellular functionality and tissue development over time.91
Tailoring the stress relaxation properties of ATPS bioinks enables the engineering of constructs that mimic the adaptive mechanics of native tissues. This tunability ensures that the mechanical environment evolves alongside cellular activity, supporting sustained tissue development while maintaining the stability of the printed structure.
3. Mechanotransduction in ATPS-based bioprinting
Cellular performance in response to the parameters and characteristics of ATPS bioinks is made possible by the ability of cells to perceive physical and mechanical stimuli from their environment and convert them into biological responses, a process known as mechanotransduction. By definition, mechanotransduction is a critical process by which cells sense and respond to biophysical and biomechanical cues in their surrounding environment. Guided by changes in the mechanical properties of their microenvironment, cells navigate pathways that determine their fate, transitioning through quiescence, proliferation, maturation, activation, and even apoptosis.91 Key factors that act as biophysical and biomechanical signal includes matrix architecture and stiffness, surface topography and morphology, electromagnetic forces, negative pressure, electrical stimulation, and mechanical forces.91
Cells detect these environmental cues through specialized macromolecular complexes known as mechanosensors and, in response to unfavorable conditions, activate adaptive mechanisms.92 Among the cellular mechanosensory proteins, cytoskeletal components play a crucial role in transmitting mechanical stimuli from the extracellular environment to the cell nucleus. This process is mediated by transmembrane proteins located at focal adhesions, which bind not only to ECM ligands but also to intracellular proteins. Similarly, cadherins connect the cytoskeletons of adjacent cells, enabling the transmission of mechanical signals between them. Primary cilia sense external fluid flow, pressure, and tension, triggering ion flux through channels within the ciliary axoneme, which regulates intracellular signaling. Additionally, other membrane proteins can be modulated by shear stress and mechanical tension.93 Extracellular matrix components play an active role in mechanosensation and mechanotransduction, as their organization varies across tissues, creating either softer or stiffer environments. These components directly interact with the cytoskeleton, transmitting physical and mechanical signals to cells. In response, cells may adjust their behavior by, for example, upregulating the expression of ECM remodeling enzymes.91
Mechanotransduction begins with the activation of signaling pathways triggered by mechanosensors after detection of biophysical or biomechanical cues. Besides essential proteins, such as calcium channels, MAPK, and G-protein-coupled receptors (GPCRs) which are readily activated by mechanical and physical forces, one of the main pathways involved in mechanotransduction is the YAP/TAZ signaling pathway, which is canonically regulated by the Hippo pathway. In this pathway, YAP/TAZ are phosphorylated, keeping them sequestered in the cytoplasm, where they are eventually degraded. Upon inactivation of the Hippo pathway, dephosphorylated YAP/TAZ translocate to the nucleus, where they complex with TEAD transcription factors to activate genes that regulate proliferation, differentiation, and cell survival.94 In mechanotransduction, ECM stiffness and cytoskeletal tension can promote the nuclear translocation of YAP/TAZ independently of Hippo signaling, adjusting cellular responses according to environmental changes. For instance, in stem cells, a stiff substrate promotes YAP/TAZ nuclear signaling, favoring osteogenic differentiation, whereas on soft substrates, the signaling remains restricted to the cytoplasm, favoring adipogenic differentiation.95 The Rho/ROCK pathway also plays a crucial role in mechanotransduction, exhibiting hyperactivation in response to increased matrix stiffness, which modulates the assembly of cytoplasmic stress fibers and can trigger apoptosis. Due to its significance in stem cell mechanotransduction, ROCK inhibitors are frequently used to enhance the survival and clonogenic capacity of stem cells.96 Focal adhesion kinase (FAK) is another key protein involved in mechanotransduction. Increased cellular mechanical tension enhances the forces detected by focal adhesions, leading to FAK activation. FAK plays a critical role in regulating several pathways that modulate cell differentiation and migration.97,98
Figure 7 presents a simplified schematic illustrating how mechanical forces such as ECM stiffness, fluid shear stress, and matrix tension are detected by membrane proteins and transmitted to the nucleus through intracellular signaling pathways. These mechanical cues are then converted into biochemical signals that regulate gene expression, ultimately influencing cellular behavior, including proliferation, differentiation, and ECM remodeling.
Figure 7. Overview of mechanotransduction at the subcellular level and its effects on cell behavior. Mechanical cues such as extracellular matrix (ECM) stiffness, fluid shear stress, and tensile forces are detected by mechanosensors, particularly integrins and mechanosensitive ion channels (e.g., calcium channels). Integrin activation recruits focal adhesion kinase (FAK), which phosphorylates downstream effectors, leading to the activation of the RhoA/ROCK pathway, modulating cytoskeletal tension and cellular contractility. Simultaneously, calcium influx via mechanosensitive channels influences downstream effectors, including YAP/TAZ, which translocate to the nucleus to regulate gene transcription. These biochemical signals drive cellular responses such as proliferation, differentiation, apoptosis, and ECM remodeling. Adapted under the terms of the Creative Commons CC BY license, Advanced Science, 2023.99
In bioprinting, recreating these complex mechanical microenvironments is critical for mimicking physiological conditions and allowing cells to exhibit their native behaviors.100 The tunability of ATPS-derived microgel bioinks addresses this challenge by tailoring their physical properties to modulate mechanotransduction pathways during and after bioprinting.101–103 ATPS phase-separated structure provides localized control over stiffness gradients, mimicking ECM organization and facilitating spatially specific mechanotransduction. Additionally, their key biophysical properties discussed in Section 2.3, such as viscosity, viscoelasticity, and stress relaxation, directly impact cellular behavior. ATPS-derived microgel bioinks with tunable shear-thinning properties ensure reduced shear stress during printing, which can preserve mechanosensitive signaling pathways like YAP/TAZ and integrin-mediated responses.
While ATPS bioinks mitigate many mechanotransduction challenges, the bioprinting process itself introduces additional mechanical stresses.104 Nozzle-based techniques, such as inkjet and extrusion-based methods, expose cells to significant shear stress, which can exceed their tolerance thresholds. Excessive shear stress can lead to irreversible cell damage, such as membrane rupture or cytoskeletal disruption, ultimately leading to apoptosis or necrosis.105 The severity of this damage depends on extrusion parameters, particularly pressure and nozzle diameter. For example, Nair et al.106 showed that higher extrusion pressures and smaller nozzle diameters reduced endothelial cell viability, while Zhang et al.82 noted similar effects, reporting decreased cell viability and morphology changes with smaller nozzles.
Although most studies do not investigate in depth the molecular mechanisms triggered by shear stress induced during bioprinting, variations in the magnitude and duration of applied forces can generate a heterogeneous cell population. Some cells exit the cell cycle and undergo necrosis, characterized by structural damage and the release of inflammatory distress signals. Another subset enters apoptosis, a programmed form of cell death that serves as a quality control mechanism. These stress-induced processes significantly affect cell viability, behavior, and overall bioprinting success.104
Stem cells are particularly sensitive to shear stress during extrusion, making them less suitable for extrusion-based approaches without specific protective strategies.107 Primary adult cells, with greater mechanical resilience, are often preferred for constructing simpler tissues. However, for fabricating complex organs that require diverse cell types in distinct bioinks, strategies must be developed to protect stem cells from adverse mechanical effects, such as modifying bioink properties or reducing shear forces during extrusion.
To mitigate shear stress-induced damage and adverse mechanotransduction effects and ensure cellular functionality and viability during bioprinting, optimizing key parameters such as extrusion pressure and nozzle size is essential.96,108 Blaeser et al.109 demonstrated that high shear forces in microvalve-based bioprinting systems can cause irreversible cellular damage to mesenchymal stem cells (MSCs), even over short periods. However, carefully controlled parameters can minimize cellular damage while maintaining printing resolution. ATPS-derived microgel bioinks, with their aqueous two-phase and tuned properties, are particularly suited for such optimizations. Also, the further use of advanced frameworks, such as computational modeling and machine learning, now enables more accurate predictions for improving cell viability during bioprinting.110
Shear forces and mechanical cues during bioprinting can be leveraged to modulate specific cell fates. Lee et al. demonstrated that blade-casting-assisted bioprinting could align collagen fibrils and transmit tension to embedded cells via the ECM. They demonstrated that this mechanical signaling triggers activation of cell-surface proteins like integrin receptors and mechanosensitive ion channels, such as transient receptor potential (TRP) and Piezo channels. These molecular sensors respond by modulating ion fluxes, which lead to the activation of downstream signaling pathways, including FAK, RhoA/ROCK, MAPK, and Wnt/β-catenin.111 These pathways collectively induce cytoskeletal reorganization, enhance cell alignment, and upregulate myogenesis-related genes, ultimately improving cellular proliferation and differentiation into aligned myotubes. The tunable properties of ATPS bioinks could similarly guide cell alignment and differentiation through phase-separated microenvironments.
3.1. Importance of stress relaxation in cells responding to mechanical cues of surrounding printed construct after printing
Cellular response to mechanical stress is fundamental to mechanotransduction, as these mechanical forces interact with the viscoelastic properties of the bioink and the surrounding matrix, forming a dynamic microenvironment that governs mechanotransduction. Biological native tissues exhibit viscoelastic properties that are essential for maintaining homeostasis and supporting functional cellular behavior by dynamically responding to mechanical stimuli. This mechanical feature arises from combining the elastic and viscous characteristics of tissues, enabling them to deform and adapt their shape during load application and to recover once the stress is removed.112 Each tissue exhibits distinct viscoelastic properties tailored to its function, such as cartilage absorbing shock, skin stretching with movement, and lungs or arteries adapting to cyclic forces. These dynamic mechanical behaviors are primarily governed by ECM composition, which includes collagen, elastin, and glycoproteins. Collagen provides tensile strength, elastin enables elasticity, and proteoglycans contribute to hydration and resistance to compressive forces.92
In bioprinting, mimicking these viscoelastic properties of native tissues is essential for recreating constructs that replicate the biophysical and biomechanical cues necessary for functional and physiologically relevant tissue formation.112–114 Stress relaxation, a key aspect of viscoelasticity, allows tissues to redistribute internal stress over time, facilitating cell migration, elongation, and matrix remodeling. ATPS-derived microgel bioinks with higher stress relaxation amplitudes enable cells to penetrate better and remodel the matrix, providing an adjustable environment for cellular elongation, migration, and proliferation. By mimicking the stress relaxation properties of native tissues, these bioinks create a dynamic mechanical microenvironment essential for successful bioprinting.115
An effective strategy to modulate stress relaxation in bioinks is by adjusting the matrix porosity. Porosity facilitates the redistribution of internal strain during relaxation, significantly influencing the ability of the material to deform under applied stress. Generally, higher void space within a matrix allows for easier material deformation and better stress redistribution. Modulating porosity, whether through traditional methods like freeze-drying or advanced approaches such as bioprinting, directly impacts mechanotransduction processes and enhances the mechanical properties of bioprinted constructs.116 For example, Li et al.117 developed an interpenetrating network hydrogel combining GelMA and fibrinogen. By modulating the concentration of these components, they achieved tunable fast stress relaxation. Faster stress relaxation enhanced 3D myoblast proliferation, differentiation, and organization into myotubes in vitro while also improving muscle functional recovery in a rat model of volumetric muscle loss.117 Similarly, Wang et al.118 developed a GelMA-based hydrogel modified with ε-poly-L-lysine (EPL) and phenylboronic acid (PBA) through photopolymerization under blue light. The bioink exhibited dynamic covalent bonding, tunable porosity, and positive surface charge. These features improved ECM adsorption, provided a viscoelastic environment mimicking native tissue, and promoted efficient nutrient diffusion. Cellular analysis showed chondrocyte viability, proliferation, and ECM secretion, as well as MSC differentiation into chondrocytes.118
In addition to porosity and architecture, the physical and mechanical properties of bioink are critical determinants of cellular behavior in bioprinted constructs.119 After surviving the bioprinting process and being accommodated within the scaffold, cells directly interact with the scaffold walls. At this interface, properties such as stiffness and elasticity play critical roles in modulating cellular behavior and guiding their responses. These mechanical properties influence mechanotransduction pathways, impacting cell adhesion, proliferation, and differentiation. Stiffness, for instance, can guide stem cell lineage specification, while elasticity affects cytoskeletal dynamics and tissue functionality.120
The influence of these parameters on bioprinting is well-established, and for years, researchers have been studying the properties of biomaterials to identify the most suitable compositions for specific applications. For example, alginate-gelatin hydrogels have been demonstrated to direct distinct differentiation pathways depending on their stiffness, mediated by the modulation of YAP/TAZ signaling. Increased stiffness enhances YAP nuclear localization, promoting both sweat gland cell differentiation and osteogenic lineage commitment, whereas reduced stiffness favors adipogenic differentiation.121 By tailoring these parameters, the microenvironment can be optimized to support the desired cellular responses, ensuring better integration and performance of the bioengineered tissue. Understanding and controlling these interactions are essential for advancing tissue engineering and regenerative medicine applications.120
Microgels encapsulation have been highlighted as effective tools in bioprinting for preserving cellular integrity during bioprinting and guiding differentiation pathways, which influence tissue formation. By mimicking the extracellular matrix, microgels support cell adhesion, proliferation, and differentiation through their customizable properties, such as stiffness, elasticity, and porosity. These features facilitate the modulation of mechanotransduction pathways, steering lineage specification, and enhancing tissue functionality.122 For example, Jia et al.29 utilized methacrylated acellular cartilage matrix (ACMMA), GelMA, and PEO to develop a bioprinted microgel that produced a porous matrix with significant potential for auricular cartilage regeneration. Their study demonstrated that the microporous bioink enhanced chondrocyte viability, uniform distribution, effective infiltration, and directed differentiation. Recent studies consistently highlight the unique advantages of ATPS microgels in bioprinting, particularly in mitigating negative mechanotransduction responses. Wang et al. introduced a tunable macroporous hydrogel system incorporating phosphorylated cellulose nanofibrils (pCNF) into a GelMA-dextran ATPS bioink. This design offered adjustable stiffness and enhanced energy dissipation, effectively reducing stress transmission to cells during bioprinting while providing optimized mechanical cues. As a result, the system demonstrated significant improvements in cell viability, spreading, and differentiation, evidenced by the upregulation of osteogenic and chondrogenic markers.28
4. Oxygen-delivering functions of ATPS-derived microgel composite bioinks
Recent advancements in bioprinting have emphasized the critical need for oxygenation within tissue-engineered constructs to ensure their survival and functionality, particularly in areas where tissue is damaged or ischemic.123–126 Among the most promising innovations are ATPS-derived microgel composite bioinks, which combine the benefits of oxygen-generating materials with cellular encapsulation and support. These bioinks are designed to enhance oxygen delivery, facilitate cellular retention, and improve the biocompatibility of printed structures, making them highly suitable for the regeneration of complex tissues.
Jeong et al.26 explored the integration of nanostructures, such as nCaO2 nanoparticles and MnO2 nanosheets, into polymeric microparticles, which, when combined with viscoelastic colloidal hydrogels, significantly enhance shear-thinning behavior and improve printability. This is crucial for the creation of macroscopic tissue constructs. The nanoparticle-encapsulated systems prevent issues of agglomeration and clogging, even when higher concentrations of oxygen-generating microparticles (OMPs) are used. By employing a formulation of few-micron-sized spherical hydrogel particles incorporated with OMPs, they have successfully 3D bioprinted scaled-down models of complex human tissues, including bone, heart, brain, and hair, all infused with oxygen-releasing materials. This approach, as illustrated in Figure 8A–D, holds immense promise for achieving the optimal oxygen levels required to support the differentiation of specific cells into target tissues, while offering both a highly porous structure and localized oxygen delivery to surrounding cells.
Figure 8. Overview of colloidal-OMP bioink development and applications. Section 1 depicts the printing of colloidal OMP-hMSCs bioinks: (A) schematic illustrating the development of gelatin/GelMA colloidal bioinks combined with nCaO₂ and nMnO₂ nanoparticles encapsulated in PCL microspheres, designed to mimic physiological oxygen levels in targeted tissues by modulating OMP content. (B) Characterization of OMPs, showcasing their structural and distribution properties, along with tunable oxygen-releasing capabilities, enabling tailored oxygen microenvironments to enhance cell viability and functionality post-bioprinting. (C) Fluorescent images and photographs of 3D-printed tissue-mimicking constructs (bone, heart, brain, and hair) printed using 0.1% OMP-Gel bioinks. (D) Live (green)/dead (red) fluorescent images of 3D-printed constructs (bone, heart, brain, and hair) using HDF-laden 0% and 0.1% OMP-Gel bioinks after 7 days of culture under hypoxic conditions (1% oxygen). Constructs with 0.1% OMP-Gel exhibited higher cell viability and improved spreading compared to 0% OMP-Gel, highlighting the role of OMPs in enhancing localized oxygen delivery and creating a supportive microenvironment for cell growth. Reproduced with permission. Copyright © 2024 Springer Nature.26 Section 2 illustrates adhesive colloidal OMP-hMSC hydrogel systems for ischemic disease treatment. (E) Conceptual schematic illustrating oxygenating and cell-tethering colloidal hydrogels, which enhance paracrine factor production, promote blood flow restoration, and induce muscle regeneration in hindlimb ischemia models. (F) Histological evaluation of subcutaneous implantation of hMSC-laden TOC hydrogels after 2 weeks, showing vascular integration via hematoxylin-eosin and Masson’s trichrome (M&T) staining. (G) In vivo blood flow restoration in hindlimb of ischemia-treated mice, demonstrated through intramuscular delivery of hMSC-laden TOC hydrogels and corresponding LDI imaging at 0, 3, 7, and 14 days post-injection. Reproduced with permission. Copyright © 2024 John Wiley and Sons.53 Abbreviations: HDF, human dermal fibroblasts; hMSC, human mesenchymal stem cell; LDI, laser Doppler imaging; OMP, oxygen-generating microparticles; PCL, polycaprolactone; TOC, tyramine-conjugated colloidal hydrogels.
Lee et al.53 newly designed the cell-tethering and hypoxic conditioning hydrogels composed of ATPS granular gels, and hMSCs along with OMP systems. These gels not only convert a severe hypoxic microenvironment into a mild hypoxic one (~8 mmHg pO2) through oxygen release but also provide cell-adhesion capabilities via a tyramine-derivatized functional group and feature interconnected microporous networks. As shown in Figure 8E, this architecture enables encapsulated human MSCs to efficiently adhere to the hydrogel matrix while simultaneously releasing a range of secreted factors, including vascular endothelial growth factor (VEGF), angiogenin (ANG), insulin-like growth factor binding protein (IGFBP), pentraxin 3 (PTX3), placental growth factor (PIGF), and stromal cell-derived factor 1 alpha (SDF-1α). In a murine hindlimb ischemia (HLI) model, blood flow recovery in the oxygenating colloidal gel (TOC)-treated mice was nearly double that in untreated HLI mice (Figure 8G). Histological analysis revealed significantly larger muscle mass in the TOC-treated group, attributed to the presence of more abundant and larger-diameter blood vessels than in the control group. Furthermore, the TOC-treated hydrogel exhibited the highest expression levels of key angiogenic growth factors (VEGF, PIGF, and IGFBP3) at the treatment site (Figure 8F). The recruitment of hematopoietic stem cells (HSCs) to the hydrogel was indirectly confirmed through the chemokine SDF-1α released from TOC treated hydrogel.
Collectively, these findings underscore the potential of highly functional ATPS-based hydrogel as a highly effective therapeutic tool to enhance the paracrine effects of hMSCs. This system holds great promise for advancing vascular function and offers valuable therapeutic applications, particularly in the treatment of ischemic diseases. Moreover, when integrated into a bioprinting system, it could enable the creation of patient-specific designs, allowing for the fabrication of more complex, hierarchical structures that can be precisely implanted. Such an approach would provide enhanced versatility and customization in therapeutic interventions, offering significant potential for personalized medicine and optimization of treatment outcomes in regenerative medicine.
In summary, the integration of oxygen-generating materials within ATPS-derived microgel composite bioinks represents a major breakthrough in tissue bioprinting, offering enhanced cell survival, localized oxygen delivery, and improved regenerative capacity. The development of these bioinks holds great potential for advancing tissue engineering and regenerative medicine, particularly for the treatment of ischemic conditions and other pathologies requiring effective vascular regeneration.
5. Conclusions and future perspectives
The ATPS-derived microgel not only provides an ideal environment for serving as a cell carrier and support matrix but also facilitates in-depth therapeutic interventions for ischemic tissues by enabling hypoxic conditioning and incorporating cell-tethering functionality. Recent developments in composite ATPS-derived microgel bioinks have demonstrated the potential to address these needs effectively. This study also explored the surface roughness and mechanical strength of each ATPS-derived microgel component, which are crucial for optimizing performance. However, while the incorporation of hMSCs in the bioink showed successful cell tethering and maintained paracrine factor release in the printed constructs, the impact of surrounding materials—such as the stiffness of gelatin-based GelMA microgels and the mechanical properties of oxygen-releasing microparticles—on hMSC behavior post-printing has yet to be fully explored. The process of cell tethering likely influences the interaction between cells and the microgel as well as the composite material’s surface, affecting the mechanotransduction pathways of hMSCs. A deeper understanding of these interactions, particularly in the context of an ischemic muscle injury model as discussed in this paper, could lead to the design of tissue-specific ATPS-derived microgel bioinks that are programmed to promote cell differentiation into muscle cells, for example. This would allow for the creation of bioinks tailored to the specific mechanical properties and cellular needs of different tissues. In this regard, the review paper holds significant value in offering a platform for the systematic selection and optimization of materials that cater to the specific requirements of diverse applications, thereby contributing to more effective tissue engineering strategies.
Beyond addressing these macroscopic considerations, advancing the field of ATPS-derived microgel bioinks or overall bioinks demands a shift toward exploring the molecular mechanisms underlying mechanotransduction pathways. Few studies have delved deeply into the precise molecular mechanisms underlying mechanotransduction pathways triggered by the interaction between cells and ATPS microgels. Overall, current research often prioritizes evaluating basic cell survival and simpler performance metrics. When a material fails to meet these initial benchmarks, it is frequently dismissed from consideration for tissue engineering applications. However, this superficial approach overlooks the potential of molecular-level insights to revolutionize material design. Understanding the molecular protagonists involved in cellular mechanotransduction responses could provide critical information for modifying materials to enhance their functionality. For example, identifying specific proteins or signaling pathways activated during cell–material interactions could inform the development of advanced biomaterials engineered to target these molecules. Such materials would not only support cell survival but also actively modulate cellular behavior to promote tissue-specific differentiation and functionality. By integrating these molecular insights with material design, researchers could create optimized bioinks that simultaneously address mechanical, structural, and biological requirements. This deeper exploration of molecular mechanisms would open new avenues for achieving highly effective regenerative medicine and tissue engineering strategies.
1.Chen F, Li X, Yu Y, et al. Phase-separation facilitated one-step fabrication of multiscale heterogeneous two-aqueous-phase gel. Nat Commun. 2023;14(1):2793.
doi: 10.1038/s41467-023-38394-9
2.An C, Zhang S, Xu J, et al. The microparticulate inks for bioprinting applications. Mater Today Bio. 2023; 24:100930.
doi: 10.1016/j.mtbio.2023.100930
3.Wang Q, Karadas Ö, Backman O, et al. Aqueous two-phase emulsion bioresin for facile one-step 3D microgel-based bioprinting. Adv Healthc Mater. 2023;12(19):e2203243.
4.Tuftee C, Alsberg E, Ozbolat IT, Rizwan M. Emerging granular hydrogel bioinks to improve biological function in bioprinted constructs. Trends Biotechnol. 2024;42(3):339-352.
doi: 10.1016/j.tibtech.2023.09.007
5.He W, Deng J, Ma B, et al. Recent advancements of bioinks for 3D bioprinting of human tissues and organs. ACS Appl Bio Mater. 2024;7(1):17-43.
6.Jain P, Kathuria H, Dubey N. Advances in 3D bioprinting of tissues/organs for regenerative medicine and in-vitro models. Biomaterials. 2022;287:121639.
doi: 10.1016/j.biomaterials.2022.121639
7.Xin S, Deo KA, Dai J, et al. Generalizing hydrogel microparticles into a new class of bioinks for extrusion bioprinting. Sci Adv. 2021;7(42):eabk3087.
8.Riley L, Schirmer L, Segura T. Granular hydrogels: emergent properties of jammed hydrogel microparticles and their applications in tissue repair and regeneration. Curr Opin Biotechnol. 2019;60:1-8.
doi: 10.1016/j.copbio.2018.11.001
9.Highley CB, Song KH, Daly AC, Burdick JA. Jammed microgel inks for 3D printing applications. Adv Sci (Weinh). 2018;6(1):1801076.
10.Kajtez J, Wesseler MF, Birtele M, et al. Embedded 3D printing in self-healing annealable composites for precise patterning of functionally mature human neural constructs. Adv Sci (Weinh). 2022;9(25):e2201392.
11.Daly AC, Riley L, Segura T, Burdick JA. Hydrogel microparticles for biomedical applications. Nat Rev Mater. 2020;5(1):20-43.
doi: 10.1038/s41578-019-0148-6
12.Daly AC. Granular hydrogels in biofabrication: recent advances and future perspectives. Adv Healthc Mater. 2024;13(25):e2301388.
13.Wang C, Zhang Z, Wang Q, et al. Aqueous two-phase emulsions toward biologically relevant applications. Trends Chem. 2023;5(1):61-75.
doi: 10.1016/j.trechm.2022.10.009
14.Ying GL, Jiang N, Maharjan S, et al. Aqueous two-phase emulsion Bioink-enabled 3D bioprinting of porous hydrogels. Adv Mater. 2018;30(50):e1805460.
15.Zhang Y, Luo Y, Zhao J, et al. Emerging delivery systems based on aqueous two-phase systems: a review. Acta Pharm Sin B. 2024;14(1):110-132.
doi: 10.1016/j.apsb.2023.08.024
16.Mierke CT. Bidirectional mechanical response between cells and their microenvironment. Front Phys. 2021;9:749830.
17.Chao Y, Shum HC. Emerging aqueous two-phase systems: from fundamentals of interfaces to biomedical applications. Chem Soc Rev. 2020;49(1):114-142.
doi: 10.1039/C9CS00466A
18.Iqbal M, Tao Y, Xie S, et al. Aqueous two-phase system (ATPS): an overview and advances in its applications. Biol Proced Online. 2016;18:18.
doi: 10.1186/s12575-016-0048-8
19.Fick C, Khan Z, Srivastava S. Interfacial stabilization of aqueous two-phase systems: a review. Mater Adv. 2023;4(20):4665-4678.
doi: 10.1039/D3MA00307H
20.Dumas F, Roger E, Rodriguez R, et al. Aqueous two-phase systems: simple one-step process formulation and phase diagram for characterisation. Colloid Polymer Sci. 2020;298(12):1629-1636.
doi: 10.1007/s00396-020-04748-8
21.Liu L, Ngai T. Pickering emulsions stabilized by binary mixtures of colloidal particles: synergies between contrasting properties. Langmuir. 2022;38(44):13322-13329.
doi: 10.1021/acs.langmuir.2c02338
22.Yi S, Liu Q, Luo Z, et al. Micropore-forming gelatin methacryloyl (GelMA) bioink toolbox 2.0: designable tunability and adaptability for 3D bioprinting applications. Small. 2022;18(25):e2106357.
23.Ouyang L, Wojciechowski JP, Tang J, Guo Y, Stevens MM. Tunable microgel-templated porogel (MTP) bioink for 3D bioprinting applications. Adv Healthc Mater. 2022;11(8):e2200027.
24.Chengcheng D. The application and prospects of 3D printable microgel in biomedical science and engineering. IJB. 2023;9(5):753.
doi: 10.18063/ijb.753
25.Cheng W, Zhang J, Liu J, et al. Granular hydrogels for 3D bioprinting applications. VIEW 2020;1(3):20200060.
doi: 10.1002/VIW.20200060
26.Jeong S.-H, Hiemstra J, Blokzijl PV, et al. An oxygenating colloidal bioink for the engineering of biomimetic tissue constructs. Bio-Des Manuf. 2024;7(3):240-261.
doi: 10.1007/s42242-024-00281-7
27.Ying G, Jiang N, Parra C, et al. Bioprinted injectable hierarchically porous gelatin methacryloyl hydrogel constructs with shape-memory properties. Adv Funct Mater. 2020;30(46):2003740.
28.Liu T, Yi S, Liu G, et al. Aqueous two-phase emulsions-templated tailorable porous alginate beads for 3D cell culture. Carbohydr Polym. 2021;258:117702.
doi: 10.1016/j.carbpol.2021.117702
29.Jia L, Hua Y, Zeng J, et al. Bioprinting and regeneration of auricular cartilage using a bioactive bioink based on microporous photocrosslinkable acellular cartilage matrix. Bioact Mater. 2022;16:66-81.
doi: 10.1016/j.bioactmat.2022.02.032
30.Benwood C, Chrenek J, Kirsch RL, et al. Natural biomaterials and their use as bioinks for printing tissues. Bioengineering (Basel). 2021;8(2):27.
doi: 10.3390/bioengineering8020027
31.Moraes C, Simon AB, Putnam AJ, Takayama S. Aqueous two-phase printing of cell-containing contractile collagen microgels. Biomaterials. 2013;34(37):9623-9631.
doi: 10.1016/j.biomaterials.2013.08.046
32.Wang Q, Karadas O, Rosenholm JM, et al. Bioprinting macroporous hydrogel with aqueous two-phase emulsion-based bioink: in vitro mineralization and differentiation empowered by phosphorylated cellulose nanofibrils. Adv Funct Mater. 2024;34(29):2400431.
33.Levato R, Lim KS, Li W, et al. High-resolution lithographic biofabrication of hydrogels with complex microchannels from low-temperature-soluble gelatin bioresins. Mater Today Bio. 2021;12:100162.
doi: 10.1016/j.mtbio.2021.100162
34.Tao J, Zhu S, Zhou N, et al. Nanoparticle-stabilized emulsion bioink for digital light processing based 3D bioprinting of porous tissue constructs. Adv Healthc Mater. 2022;11(12):e2102810.
35.Luo G, Yu Y, Yuan Y, Chen X, Liu Z, Kong T. Freeform, reconfigurable embedded printing of all-aqueous 3D architectures. Adv Mater. 2019;31(49):e1904631.
36.Cui H, Zhang Y, Shen Y, et al. Dynamic assembly of viscoelastic networks by aqueous liquid-liquid phase separation and liquid-solid phase separation (AqLL-LS PS2). Adv Mater. 2022;34(51):e2205649.
37.Zhang S, Qi C, Zhang W, et al. In situ endothelialization of free-form 3D network of interconnected tubular channels via interfacial coacervation by aqueous-in-aqueous embedded bioprinting. Adv Mater. 2023;35(7):e2209263. doi: 10.1002/adma.202209263
38.Becker M, Gurian M, Schot M, Leijten J. Aqueous two‐phase enabled low viscosity 3D (LoV3D) bioprinting of living matter. Adv Sci (Weinh). 2023;10(8):2370046.
39.Jin Z, Seong H-G, Srivastava S, et al., 3D printing of aqueous two-phase systems with linear and bottlebrush polyelectrolytes. Angew Chem Int Ed. 2024;63(25):e202404382.
40.Beldengrün Y, Aragon J, Prazeres SF, Montalvo G, Miras J, Esquena J. Gelatin/maltodextrin water-in-water (W/W) emulsions for the preparation of cross-linked enzyme-loaded microgels. Langmuir. 2018;34(33):9731-9743.
doi: 10.1021/acs.langmuir.8b01599.
41.Wang A, Madden LA, Paunov VN. Vascularized co-culture clusteroids of primary endothelial and Hep-G2 cells based on aqueous two-phase pickering emulsions. Bioengineering (Basel). 2022;9(3):126.
doi: 10.3390/bioengineering9030126.
42.Mytnyk S, Ziemecka I, Olive AGL, et al. Microcapsules with a permeable hydrogel shell and an aqueous core continuously produced in a 3D microdevice by all-aqueous microfluidics. RSC Adv. 2017;7(19):11331-11337.
doi: 10.1039/C7RA00452D
43.He H, Hong M, Yang F, et al. Preparation of controlled multicompartmental gel microcarriers based on aqueous two-phase emulsions for 3D partitioned cell coculture in vitro. Biomacromolecules. 2024;25(7):4469-4481.
doi: 10.1021/acs.biomac.4c00516
44.Hori A, Watabe Y, Yamada M, et al. One-step formation of microporous hydrogel sponges encapsulating living cells by utilizing bicontinuous dispersion of aqueous polymer solutions. ACS Appl Bio Mater. 2019;2(5):2237-2245.
45.Tavana H, Mosadegh B, Takayama S. Polymeric aqueous biphasic systems for non-contact cell printing on cells: engineering heterocellular embryonic stem cell niches. Adv Mater. 2010;22(24):2628-2631.
46.Robinson S, Chang J, Parigoris E, Hecker L, Takayama S. Aqueous two-phase deposition and fibrinolysis of fibroblast-laden fibrin micro-scaffolds. Biofabrication. 2021;13(3):10.1088/1758-5090/abdb85.
47.Aydın D, Kızılel S. Water-in-water emulsion based synthesis of hydrogel nanospheres with tunable release kinetics. JOM. 2017;69(7):1185-1194.
doi: 10.1007/s11837-016-1969-z
48.Oh H, Kang M, Bae E. et al. Fabrication of hydrogel microchannels using aqueous two-phase printing for 3D blood brain barrier. BioChip J 2023;17:369-383.
doi: 10.1007/s13206-023-00110-6
49.Zhu J, He Y, Wang Y, et al. Voxelated bioprinting of modular double-network bio-ink droplets. Nat Commun. 2024; 15:5902.
doi: 10.1038/s41467-024-49705-z.
50.Tang G, Luo Z, Lian L, et al. Liquid-embedded (bio)printing of alginate-free, standalone, ultrafine, and ultrathin-walled cannular structures. Proc Natl Acad Sci U S A. 2023;120(7):e2206762120.
51.Wang M, Li W, Luo Z, et al. A multifunctional micropore-forming bioink with enhanced anti-bacterial and anti-inflammatory properties. Biofabrication. 2022;14(2):10.1088/1758-5090/ac5936.
52.Xu Y, Liao X, Zhang L, et al. Digital light processing-based bioprinting of microtissue hydrogel arrays using dextran-induced aqueous emulsion ink. J Bioact Compat Polym. 2024;39(3):162-174.
doi: 10.1177/08839115241237327
53.Lee MC, Lee JS, Kim S, et al. Synergistic effect of hypoxic conditioning and cell-tethering colloidal gels enhanced productivity of MSC paracrine factors and accelerated vessel regeneration. Adv Mater. 2025;37(3):e2408488.
54.Asim S, Tabish TA, Liaqat U, Ozbolat IT, Rizwan M. Advances in gelatin bioinks to optimize bioprinted cell functions. Adv Healthc Mater. 2023;12(17):e2203148.
55.Qin X-S, Wang M, Li W, et al. Biosurfactant-stabilized micropore-forming GelMA inks enable improved usability for 3D printing applications. Regen Eng Transl Med. 2022;8(3):471-481.
doi: 10.1007/s40883-022-00250-5
56.Deo KA, Murali A, Tronolone JJ, et al. Granular biphasic colloidal hydrogels for 3D bioprinting. Adv Healthc Mater. 2024;13(25):e2303810.
57.Widener AE, Duraivel S, Angelini TE, Phelps EA. Injectable microporous annealed particle hydrogel based on guest-host-interlinked polyethylene glycol maleimide microgels. Adv Nanobiomed Res. 2022;2(10):2200030.
58.Wang A. Advanced Biomedical Applications of Cell Clusteroids Based on Aqueous Two-phase Pickering Emulsion Systems. University of Hull; 2022.
59.Muir VG, Qazi TH, Shan J, Groll J, Burdick JA. Influence of microgel fabrication technique on granular hydrogel properties. ACS Biomater Sci Eng. 2021;7(9): 4269-4281.
doi: 10.1021/acsbiomaterials.0c01612
60.Qazi TH, Muir VG, Burdick JA. Methods to characterize granular hydrogel rheological properties, porosity, and cell invasion. ACS Biomater Sci Eng. 2022;8(4):1427-1442.
doi: 10.1021/acsbiomaterials.1c01440
61.Wang A, Madden LA, Paunov VN. Advanced biomedical applications based on emerging 3D cell culturing platforms. J Mater Chem B. 2020;8(46):10487-10501.
doi: 10.1039/D0TB01658F
62.Teixeira AG, Agarwal R, Ko KR, et al. Emerging biotechnology applications of aqueous two-phase systems. Adv Healthc Mater. 2018;7(6):e1701036.
63.Deng X, Qi C, Meng S, et al. All-aqueous embedded 3D printing for freeform fabrication of biomimetic 3D constructs. Adv Mater. 2024;36(50):e2406825.
64.Chairez-Cantu K, González-González M, Rito-Palomares M. Novel approach for neuronal stem cell differentiation using aqueous two-phase systems in 3D cultures. J Chem Technol Biotechnol. 2021;96(1):8-13.
doi: 10.1002/jctb.6586
65.Lin Z, Beneyton T, Baret JC, Martin N. Coacervate droplets for synthetic cells. Small Methods. 2023;7(12):e2300496. doi: 10.1002/smtd.202300496
66.Keller S, Teora SP, Boujemaa M, et al. Exploring new horizons in liquid compartmentalization via microfluidics. Biomacromolecules. 2021;22(5):1759-1769.
doi: 10.1021/acs.biomac.0c01796
67.Dumas F, Benoit JP, Saulnier P, Roger E. A new method to prepare microparticles based on an Aqueous Two-Phase system (ATPS), without organic solvents. J Colloid Interface Sci. 2021;599:642-649.
doi: 10.1016/j.jcis.2021.03.141
68.Bai L, Huan S, Zhao B, et al. All-aqueous liquid crystal nanocellulose emulsions with permeable interfacial assembly. ACS Nano. 2020;14(10):13380-13390.
69.Qian X, Peng G, Ge L, Wu D. Water-in-water Pickering emulsions stabilized by the starch nanocrystals with various surface modifications. J Colloid Interface Sci. 2022;607 (Pt 2):1613-1624.
doi: 10.1016/j.jcis.2021.09.085
70.Peddireddy KR, Nicolai T, Benyahia L, Capron I. Stabilization of water-in-water emulsion by nanorods. ACS Macro Lett. 2016;5(3):283-286.
doi: 10.1021/acsmacrolett.5b00953
71.Ahmed T, Yamanishi C, Kojima T, Takayama S. Aqueous two-phase systems and microfluidics for microscale assays and analytical measurements. Annu Rev Anal Chem (Palo Alto Calif). 2021;14(1):231-255.
doi: 10.1146/annurev-anchem-091520-101759.
72.Ying G, Jiang N, Yu C, et al. Three-dimensional bioprinting of gelatin methacryloyl (GelMA). Bio-des. Manuf. 2018;1:215-224.
doi: 10.1007/s42242-018-0028-8
73.Duraivel S, Subramaniam V, Chisolm S, et al. Leveraging ultra-low interfacial tension and liquid-liquid phase separation in embedded 3D bioprinting. Biophys Rev (Melville). 2022;3(3):031307.
doi: 10.1063/5.0087387
74.Flégeau K, Puiggali-Jou A, Zenobi-Wong M. Cartilage tissue engineering by extrusion bioprinting utilizing porous hyaluronic acid microgel bioinks. Biofabrication. 2022;14(3):034105.
75.Pereira JFB, Coutinho JAP. Chapter 5 - aqueous two-phase systems. In: Poole CF, editor. Liquid-Phase Extraction. Elsevier; 2020:157-182.
doi: 10.1016/B978-0-12-816911-7.00005-0
76.Qazi TH, Wu J, Muir VG, et al. Anisotropic rod-shaped particles influence injectable granular hydrogel properties and cell invasion. Adv Mater. 2022;34(12):e2109194.
77.Guo Z, Zhang S, Guo Y. et al. Bioinspired coacervate-based bioinks for construction of multiscale tissue engineering scaffolds. Nano Res. 2024;17:8209–8219.
doi: 10.1007/s12274-024-6844-6
78.Leung BM, Labuz JM, Moraes C, et al. Chapter 9 - Bioprinting using aqueous two-phase system. In: Atala A, Yoo JJ, editors. Essentials of 3D Biofabrication and Translation. Boston: Academic Press; 2015:165–178.
79.Rogers BA, Rembert KB, Poyton MF, et al. A stepwise mechanism for aqueous two-phase system formation in concentrated antibody solutions. Proc Natl Acad Sci U S A. 2019;116(32):15784-15791.
80.Zhu M, Wang Y, Ferracci G, Zheng J, Cho NJ, Lee BH. Gelatin methacryloyl and its hydrogels with an exceptional degree of controllability and batch-to-batch consistency. Sci Rep. 2019;9(1):6863.
doi: 10.1038/s41598-019-42186-x
81.Ben Messaoud G, Aveic S, Wachendoerfer M, Fischer H, Richtering W. 3D printable gelatin methacryloyl (GelMA)-dextran aqueous two-phase system with tunable pores structure and size enables physiological behavior of embedded cells in vitro. Small. 2023;19(44):e2208089.
82.Zhang Y, O’Mahony A, He Y, et al. Hydrodynamic shear stress’ impact on mammalian cell properties and its applications in 3D bioprinting. Biofabrication. 2024;16(2):022003.
83.Bercea M. Rheology as a tool for fine-tuning the properties of printable bioinspired gels. Molecules. 2023;28(6):2766. doi: 10.3390/molecules28062766.
84.Ning L, Gil CJ, Hwang B, et al. Biomechanical factors in three-dimensional tissue bioprinting. Appl Phys Rev. 2020;7(4):041319.
doi: 10.1063/5.0023206
85.Dubbin K, Hori Y, Lewis KK, Heilshorn SC. Dual-stage crosslinking of a gel-phase bioink improves cell viability and homogeneity for 3D bioprinting. Adv Healthc Mater. 2016;5(19):2488-2492.
86.Moon D, Lee MG, Sun JY, Song KH, Doh J. Jammed microgel-based inks for 3D printing of complex structures transformable via pH/temperature variations. Macromol Rapid Commun. 2022;43(19):e2200271.
87.Kessler M, Nassisi Q, Amstad E. Does the size of microgels influence the toughness of microgel-reinforced hydrogels? Macromol Rapid Commun. 2022;43(15):e2200196.
88.MaY, HanT, YangQ, et al.Viscoelastic cell microenvironment: hydrogel-based strategy for recapitulating dynamic ECM mechanics. Adv Funct Mater. 2021; 31(24): 2100848.
89.Chaudhuri O, Gu L, Klumpers D., et al. Hydrogels with tunable stress relaxation regulate stem cell fate and activity. Nature Mater 2016;15:326–334.
doi: 10.1038/nmat4489
90.Whitaker KA, Varga Z, Hsiao LC, Solomon MJ, Swan JW, Furst EM. Colloidal gel elasticity arises from the packing of locally glassy clusters. Nat Commun. 2019;10(1):2237.
doi: 10.1038/s41467-019-10039-w
91.Saraswathibhatla A, Indana D, Chaudhuri O. Cell-extracellular matrix mechanotransduction in 3D. Nat Rev Mol Cell Biol. 2023;24(7):495-516.
doi: 10.1038/s41580-023-00583-1
92.Di X, Gao X, Peng L, et al. Cellular mechanotransduction in health and diseases: from molecular mechanism to therapeutic targets. Signal Transduct Target Ther. 2023;8(1):282.
doi: 10.1038/s41392-023-01501-9
93.Bakhshandeh B, Sorboni SG, Ranjbar N, et al. Mechanotransduction in tissue engineering: insights into the interaction of stem cells with biomechanical cues. Exp Cell Res. 2023;431(2):113766.
doi: 10.1016/j.yexcr.2023.113766
94.Jafarinia H, Khalilimeybodi A, Barrasa-Fano J, et al. Insights gained from computational modeling of YAP/TAZ signaling for cellular mechanotransduction. npj Syst Biol Appl. 2024;10:90.
doi: 10.1038/s41540-024-00414-9
95.Li Y, Wang J, Zhong W. Regulation and mechanism of YAP/TAZ in the mechanical microenvironment of stem cells (Review). Mol Med Rep. 2021;24(1):506.
96.Naqvi SM, McNamara LM. Stem cell mechanobiology and the role of biomaterials in governing mechanotransduction and matrix production for tissue regeneration. Front Bioeng Biotechnol. 2020;8:597661.
doi: 10.3389/fbioe.2020.597661
97.Zebda N, Dubrovskyi O, Birukov KG. Focal adhesion kinase regulation of mechanotransduction and its impact on endothelial cell functions. Microvasc Res. 2012;83(1):71-81. doi: 10.1016/j.mvr.2011.06.007
98.Casarella S, Ferla F, Di Francesco D, et al. Focal adhesion’s role in cardiomyocytes function: from cardiomyogenesis to mechanotransduction. Cells. 2024;13(8):664.
99.Cao R, Tian H, Tian Y, et al. A hierarchical mechanotransduction system: from macro to micro. Adv Sci. 2024;11(11):2302327.
100.Wang J, Cui Z, Maniruzzaman M. Bioprinting: a focus on improving bioink printability and cell performance based on different process parameters. Int J Pharm. 2023;640: 123020.
doi: 10.1016/j.ijpharm.2023.123020
101.Gonzalez-Fernandez T, Tenorio AJ, Campbell KT, Silva EA, Leach JK. Alginate-based bioinks for 3D bioprinting and fabrication of anatomically accurate bone grafts. Tissue Eng Part A. 2021;27(17-18):1168-1181.
doi: 10.1089/ten.TEA.2020.0305
102.Somasekhar L, Huynh ND, Vecheck A, Kishore V, Bashur CA, Mitra K. Three-dimensional printing of cell-laden microporous constructs using blended bioinks. J Biomed Mater Res A. 2022;110(3):535-546.
doi: 10.1002/jbm.a.37303
103.Lee SJ, Seok JM, Lee JH, Lee J, Kim WD, Park SA. Three-Dimensional Printable Hydrogel Using a Hyaluronic Acid/Sodium Alginate Bio-Ink. Poly (Basel). 2021;13(5):794.
104.Snyder J, Rin Son A, Hamid Q, Wang C, Lui Y, Sun W. Mesenchymal stem cell printing and process regulated cell properties. Biofabrication. 2015;7(4):044106.
doi: 10.1088/1758-5090/7/4/044106
105.Xu HQ, Liu JC, Zhang ZY, Xu CX. A review on cell damage, viability, and functionality during 3D bioprinting. Mil Med Res. 2022;9(1):70.
doi: 10.1186/s40779-022-00429-5
106.Nair K, Gandhi M, Khalil S, et al. Characterization of cell viability during bioprinting processes. Biotechnol J. 2009;4(8):1168-1177.
107.Sabzevari A, Rayat Pisheh H, Ansari M, Salati A. Progress in bioprinting technology for tissue regeneration. J Artif Organs. 2023;26(4):255-274.
doi: 10.1007/s10047-023-01394-z
108.Shafiee A, Kassis J, Atala A, et al. Acceleration of tissue maturation by mechanotransduction-based bioprinting. Phys Rev Res. 2021;3(1):013008.
doi: 10.1103/PhysRevResearch.3.013008
109.Blaeser A, Duarte Campos DF, Puster U, Richtering W, Stevens MM, Fischer H. Controlling shear stress in 3D bioprinting is a key factor to balance printing resolution and stem cell integrity. Adv Healthc Mater. 2016; 5(3):326-333.
110.Zhang C, Elvitigala KCML, Mubarok W, et al. Machine learning-based prediction and optimisation framework for as-extruded cell viability in extrusion-based 3D bioprinting. Virtual Phys Prototyp. 2024;19(1):e2400330.
doi: doi.org/10.1080/17452759.2024.2400330
111.Lee S, Kim W, Kim G. Efficient myogenic activities achieved through blade-casting-assisted bioprinting of aligned myoblasts laden in collagen bioink. Biomacromolecules. 2023;24(11):5219-5229.
doi: 10.1021/acs.biomac.3c00749.
112.Dey K, Roca E, Ramorino G, et al. Progress in the mechanical modulation of cell functions in tissue engineering. Biomater Sci. 2020;8(24):7033-7081.
doi: 10.1039/D0BM01255F
113.Liu S, Yu JM, Gan YC, et al. Biomimetic natural biomaterials for tissue engineering and regenerative medicine: new biosynthesis methods, recent advances, and emerging applications. Military Med Res. 2023;10:16.
doi: 10.1186/s40779-023-00448-w
114.Wu DT, Jeffreys N, Diba M, Mooney DJ. Viscoelastic biomaterials for tissue regeneration. Tissue Eng Part C Methods. 2022;28(7):289-300.
doi: 10.1089/ten.TEC.2022.0040
115.Hazur J, Endrizzi N, Schubert DW, et al. Stress relaxation amplitude of hydrogels determines migration, proliferation, and morphology of cells in 3-D culture. Biomater Sci. 2022;10(1):270-280.
doi: 10.1039/D1BM01089A
116.Statnik ES, Salimon AI, Gorshkova YE, Kaladzinskaya NS, Markova LV, Korsunsky AM. Analysis of stress relaxation in bulk and porous ultra-high molecular weight polyethylene (UHMWPE). Polymers (Basel). 2022;14(24):5374.
117.Li T, Hou J, Wang L, et al. Bioprinted anisotropic scaffolds with fast stress relaxation bioink for engineering 3D skeletal muscle and repairing volumetric muscle loss. Acta Biomater. 2023;156:21-36.
doi: 10.1016/j.actbio.2022.08.037
118.Wang KY, Jin XY, Ma YH, et al. Injectable stress relaxation gelatin-based hydrogels with positive surface charge for adsorption of aggrecan and facile cartilage tissue regeneration. J Nanobiotechnol. 2021;19:214.
doi: 10.1186/s12951-021-00950-0
119.Kamperman T, Henke S, van den Berg A, et al. Single cell microgel based modular bioinks for uncoupled cellular micro- and macroenvironments. Adv Healthcare Mater. 2017;6(3):1600913.
120.Rajendran AK, Sankar D, Amirthalingam S, Kim HD, Rangasamy J, Hwang NS. Trends in mechanobiology guided tissue engineering and tools to study cell-substrate interactions: a brief review. Biomater Res. 2023; 27(1):55.
doi: 10.1186/s40824-023-00393-8
121.Liu Y, Li J, Yao B, et al. The stiffness of hydrogel-based bioink impacts mesenchymal stem cells differentiation toward sweat glands in 3D-bioprinted matrix. Mater Sci Eng C Mater Biol Appl. 2021;118:111387.
doi: 10.1016/j.msec.2020.111387
122.Xuan L, Hou Y, Liang L, et al. Microgels for cell delivery in tissue engineering and regenerative medicine. Nanomicro Lett. 2024;16(1):218.
doi: 10.1007/s40820-024-01421-5
123.Suvarnapathaki S, Wu X, Lantigua D, et al. Breathing life into engineered tissues using oxygen-releasing biomaterials. NPG Asia Mater. 2019;11:65.
doi: 10.1038/s41427-019-0166-2
124.Wang LH, Ernst AU, An D, et al. A bioinspired scaffold for rapid oxygenation of cell encapsulation systems. Nat Commun. 2021;12(1):5846.
doi: 10.1038/s41467-021-26126-w.
125.Lu Z, Jiang X, Chen M, Feng L, Kang YJ. An oxygen-releasing device to improve the survival of mesenchymal stem cells in tissue engineering. Biofabrication. 2019;11(4):045012.
126.Guan Y, Niu H, Liu Z, et al. Sustained oxygenation accelerates diabetic wound healing by promoting epithelialization and angiogenesis and decreasing inflammation. Sci Adv. 2021;7(35):eabj0153.