Osteogenesis induced by magnetic responsive composite scaffolds under a static magnetic field
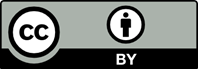
Currently, traditional osteogenesis methods face significant challenges in terms of therapeutic efficiency and biocompatibility, particularly in the context of bone repair where higher precision and efficacy are required. In this study, we fabricated a novel composite scaffold composed of polycaprolactone (PCL), polydopamine (PDA), and iron oxide nanoparticles (IONPs) and investigated its osteogenic potential. The incorporation of IONPs imparts magnetic responsiveness to the scaffold, thereby enabling the application of an external magnetic field to stimulate osteogenesis. Characterization of the scaffold confirmed its structural integrity, porosity, and biocompatibility, whereas the inclusion of PDA improved its hydrophilicity and cell adhesion properties. In vitro studies demonstrated that an external magnetic field significantly enhanced cell proliferation, osteogenic differentiation, and mineral deposition of osteoprogenitor cells cultured on the scaffolds. Furthermore, in vivo evaluation revealed that when the scaffold was exposed to magnetic stimulation, bone regeneration was accelerated, and integration of the defect site was improved. The magnetic-field-mediated approach proposed in this study effectively enhanced the osteogenic rate by augmenting the magnetic responsiveness of IONPs and combining the biocompatibility and cell-adhesion-promoting functions of PCL/PDA. This method offers a more controllable and biologically responsive alternative strategy for bone tissue regeneration with considerable potential for clinical applications.

- Park J, Lee SJ, Jung TG, et al. Surface modification of a three-dimensional polycaprolactone scaffold by polydopamine, biomineralization, and BMP-2 immobilization for potential bone tissue applications. Colloids Surf B Biointerfaces. 2021;199:111528. doi: 10.1016/j.colsurfb.2020.111528
- Li J, Yao Q, Xu Y, et al. Lithium chloride-releasing 3D printed scaffold for enhanced cartilage regeneration. Med Sci Monit. 2019;25:4041-4050. doi: 10.12659/MSM.916918
- Dong Q, Zhang M, Zhou X, et al. 3D-printed Mg-incorporated PCL-based scaffolds: a promising approach for bone healing. Mater Sci Eng C Mater Biol Appl. 2021;129:112372. doi: 10.1016/j.msec.2021.112372
- Elahi N, Rizwan M. Progress and prospects of magnetic iron oxide nanoparticles in biomedical applications: a review. Artif Organs. 2021;45(11):1272-1299. doi: 10.1111/aor.14027
- Alcala-Orozco CR, Mutreja I, Cui X, et al. Hybrid biofabrication of 3D osteoconductive constructs comprising Mg-based nanocomposites and cell-laden bioinks for bone repair. Bone. 2022;154:116198. doi: 10.1016/j.bone.2021.116198
- Li J, Li L, Zhou J, et al. 3D printed dual-functional biomaterial with self-assembly micro-nano surface and enriched nano argentum for antibacterial and bone regeneration. Appl Mater Today. 2019;17:206-215. doi: 10.1016/j.apmt.2019.06.012
- Kong N, Yang H, Tian R, et al. An injectable self-adaptive polymer as a drug carrier for the treatment of nontraumatic early stage osteonecrosis of the femoral head. Bone Res. 2022;10(1):28. doi: 10.1038/s41413-022-00196-y
- Cheng YL, Chen YW, Wang K, et al. Enhanced adhesion and differentiation of human mesenchymal stem cells inside apatite-mineralized/poly(dopamine)-coated poly(epsilon-caprolactone) scaffolds by stereolithography. J Mater Chem B. 2016;4(38): 6307-6315. doi: 10.1039/c6tb01377e
- Lutter G, Puehler T, Cyganek L, et al. Biodegradable poly- ε-caprolactone scaffolds with ECFCs and iMSCs for tissue-engineered heart valves. Int J Mol Sci. 2022;23(1):527. doi: 10.3390/ijms23010527
- Abdelaziz D, Hefnawy A, Al-Wakeel E, et al. New biodegradable nanoparticles-in-nanofiber-based membranes for guided periodontal tissue and bone regeneration with enhanced antibacterial activity. J Adv Res. 2021;28:51-62. doi: 10.1016/j.jare.2020.06.014
- Dikici S, Aldemir Dikici B, MacNeil S, et al. Decellularized extracellular matrix decorated PCL PolyHIPE scaffolds for enhanced cellular activity, integration, and angiogenesis. Biomater Sci. 2021;9(21):7297-7310. doi: 10.1039/d1bm01262b
- Wei P, Xu Y, Zhang H, et al. Continued sustained insulin-releasing PLGA nanoparticles modified 3D-Printed PCL composite scaffolds for osteochondral repair. Chem Eng J. 2021;422:130051. doi: 10.1016/j.cej.2021.130051
- Kao CY, Lin TL, Lin YH, et al. Synergistic effect of static magnetic fields and 3D-printed iron-oxide-nanoparticle-containing calcium silicate/poly-ε-caprolactone scaffolds for bone tissue engineering. Cells. 2022;11(24):3967. doi: 10.3390/cells11243967
- Li L, Li J, Guo J, et al. 3D molecularly functionalized cell-free biomimetic scaffolds for osteochondral regeneration. Adv Funct Mater. 2019;29(6):1807356. doi: 10.1002/adfm.201807356
- Liu Y, Peng L, Li L, et al. 3D-bioprinted BMSC-laden biomimetic multiphasic scaffolds for efficient repair of osteochondral defects in an osteoarthritic rat model. Biomaterials. 2021;279:121216. doi: 10.1016/j.biomaterials.2021.121216
- Ge R, Li X, Lin M, et al. Fe3O4@polydopamine composite theranostic superparticles employing packed Fe3O4 nanoparticles as the core. ACS Appl Mater Interfaces. 2016;8(35):22942-22952. doi: 10.1021/acsami.6b07997
- Karahaliloğlu Z, Yalçın E, Demirbilek M, et al., 2017, Magnetic silk fibroin e-gel scaffolds for bone tissue engineering applications. J Bioact Compat Polym. 2017;32(6):596-614. doi: 10.1177/0883911517693635
- Fini M, Cadossi R, Canè V, et al. The effect of pulsed electromagnetic fields on the osteointegration of hydroxyapatite implants in cancellous bone: a morphologic and microstructural in vivo study. J Orthop Res. 2002;20(4):756-763. doi: 10.1016/s0736-0266(01)00158-9
- Wu XD, Kang L, Tian J, et al. Exosomes derived from magnetically actuated bone mesenchymal stem cells promote tendon-bone healing through the miR-21-5p/ SMAD7 pathway. Mater Today Bio. 2022;15:100319. doi: 10.1016/j.mtbio.2022.100319
- Sun J, Liu X, Huang J, et al. Magnetic assembly mediated enhancement of differentiation of mouse bone marrow cells cultured on magnetic colloidal assemblies. Sci Rep. 2014;4:5125. doi: 10.1038/srep05125
- Huang Z, He Y, Chang X, et al. A magnetic iron oxide/ polydopamine coating can improve osteogenesis of 3D-printed porous titanium scaffolds with a static magnetic field by upregulating the TGFβ-Smads pathway. Adv Healthc Mater. 2020;9(14):e2000318. doi: 10.1002/adhm.202000318
- Yan Z, Sun T, Tan W, et al. Magnetic field boosts the transmembrane transport efficiency of magnesium ions from PLLA bone scaffold. Small. 2023;19(40): e2301426. doi: 10.1002/smll.202301426
- Wang Q, Chen B, Ma F, et al. Magnetic iron oxide nanoparticles accelerate osteogenic differentiation of mesenchymal stem cells via modulation of long noncoding RNA INZEB2. Nano Res. 2016;10(2):626-642. doi: 10.1007/s12274-016-1322-4
- Abar E, Vandghanooni S, Torab A, et al. A comprehensive review on nanocomposite biomaterials based on gelatin for bone tissue engineering. Int J Biol Macromol. 2024; 254(Pt 1):127556. doi: 10.1016/j.ijbiomac.2023.127556
- García-Lamas L, Lozano D, Jiménez-Díaz, V, et al. Enriched mesoporous bioactive glass scaffolds as bone substitutes in critical diaphyseal bone defects in rabbits. Acta Biomater. 2024;180:104-114. doi: 10.1016/j.actbio.2024.04.005
- Zheng L, Zhuang Z, Li Y, et al. Bone targeting antioxidative nano-iron oxide for treating postmenopausal osteoporosis. Bioact Mater. 2022;14:250-261. doi: 10.1016/j.bioactmat.2021.11.012
- Kim EC, Leesungbok R, Lee SW, et al. Effects of moderate-intensity static magnetic fields on human bone marrow-derived mesenchymal stem cells. Bioelectromagnetics. 2015;36(4):267-276. doi: 10.1002/bem.21903
- Liao W, Lu J, Wang Q, et al. Osteogenesis of iron oxide nanoparticle-labeled human precartilaginous stem cells in penetrating network table hydrogel. Front Bioeng Biotechnol. 2022;10:872149. doi: 10.3389/fbioe.2022.872149
- Fu C, Jiang Y, Yang X, et al. Mussel-inspired gold nanoparticles and PLGA/L-Lysine-g-graphene oxide composite scaffolds for bone defect repair. Int J Nanomedicine. 2021;16:6693-6718. doi: 10.2147/ijn.S328390
- Ghorbani F, Kim M, Monavari M, et al. Mussel-inspired polydopamine-decorated alginate dialdehyde-gelatin 3D printed scaffolds for bone tissue engineering applications. Front Bioeng Biotechnol. 2022;10:940070. doi: 10.3389/fbioe.2022.940070
- Li Y, Wu R, Yu L, et al. Rational design of nonstoichiometric bioceramic scaffolds via digital light processing: tuning chemical composition and pore geometry evaluation. J Biol Eng. 2021;15(1):1. doi: 10.1186/s13036-020-00252-3
- Gharibshahian M, Salehi M, Beheshtizadeh N, et al. Recent advances on 3D-printed PCL-based composite scaffolds for bone tissue engineering. Front Bioeng Biotechnol. 2023;11:1168504. doi: 10.3389/fbioe.2023.1168504
- Zhang X, Yan Q, Liu X, et al. LncRNA00638 promotes the osteogenic differentiation of periodontal mesenchymal stem cells from periodontitis patients under static mechanical strain. Stem Cell Res Ther. 2023;14(1):177. doi: 10.1186/s13287-023-03404-6
- Bedford EE, Méthivier CM, Pradier C, et al. Nanostructured and spiky gold shell growth on magnetic particles for SERS applications. Nanomaterials (Basel). 2020;10(11):2136. doi: 10.3390/nano10112136
- Song X, Liu X, Ma Y, et al. Synthesis of Ce/Gd@HA/PLGA scaffolds contributing to bone repair and MRI enhancement. Front Bioeng Biotechnol. 2022;10:834226. doi: 10.3389/fbioe.2022.834226
- Li X, Wei Z, Lv H, et al. Iron oxide nanoparticles promote the migration of mesenchymal stem cells to injury sites. Int J Nanomedicine. 2019;14:573-589. doi: 10.2147/IJN.S184920
- Wang S, Gu R, Wang F, et al. 3D-printed PCL/Zn scaffolds for bone regeneration with a dose-dependent effect on osteogenesis and osteoclastogenesis. Mater Today Bio. 2022;13:100202. doi: 10.1016/j.mtbio.2021.100202
- Li D, Zheng S, Wei P, et al. Synchronized long-term delivery of growth hormone and insulin-like growth factor 1 through poly (lactic-co-glycolic acid) nanoparticles on polycaprolactone scaffolds for enhanced osteochondral regeneration. Int J Biol Macromol. 2024;282(Pt 4):136781. doi: 10.1016/j.ijbiomac.2024.136781
- Xu J, Liu K, Chen T, et al. Rotating magnetic field delays human umbilical vein endothelial cell aging and prolongs the lifespan of Caenorhabditis elegans. Aging (Albany NY). 2019;11(22):10385-10408. doi: 10.18632/aging.102466
- Chen G, Zhuo Y, Tao B, et al. Moderate SMFs attenuate bone loss in mice by promoting directional osteogenic differentiation of BMSCs. Stem Cell Res Ther. 2020;11(1):487. doi: 10.1186/s13287-020-02004-y
- Marycz K, Alicka M, Kornicka-Garbowska K, et al. Promotion through external magnetic field of osteogenic differentiation potential in adipose-derived mesenchymal stem cells: design of polyurethane/poly(lactic) acid sponges doped with iron oxide nanoparticles. J Biomed Mater Res B Appl Biomater. 2020;108(4):1398-1411. doi: 10.1002/jbm.b.34488
- Meng J, Xiao B, Zhang Y, et al. Super-paramagnetic responsive nanofibrous scaffolds under static magnetic field enhance osteogenesis for bone repair in vivo. Sci Rep. 2013;3:2655. doi: 10.1038/srep02655
- Mouthuy PA, Somogyi Škoc M, Čipak Gašparović A, et al. Investigating the use of curcumin-loaded electrospun filaments for soft tissue repair applications. Int J Nanomedicine. 2017;12:3977-3991. doi: 10.2147/ijn.S133326
- Zhao YZ, Chen R, Xue PP, et al. Magnetic PLGA microspheres loaded with SPIONs promoted the reconstruction of bone defects through regulating the bone mesenchymal stem cells under an external magnetic field. Mater Sci Eng C Mater Biol Appl. 2021;122:111877. doi: 10.1016/j.msec.2021.111877
- Fujita T, Azuma Y, Fukuyama R, et al. Runx2 induces osteoblast and chondrocyte differentiation and enhances their migration by coupling with PI3K-Akt signaling. J Cell Biol. 2004;166(1):85-95. doi: 10.1083/jcb.200401138
- Madel MB, Ibáñez L, Ciucci T, et al. Dissecting the phenotypic and functional heterogeneity of mouse inflammatory osteoclasts by the expression of Cx3cr1. Elife. 2020;9:e54493. doi: 10.7554/eLife.54493
- Ruolan W, Liangjiao C, Longquan S. The mTOR/ULK1 signaling pathway mediates the autophagy-promoting and osteogenic effects of dicalcium silicate nanoparticles. J Nanobiotechnology. 2020;18(1):119. doi: 10.1186/s12951-020-00663-w
- Kaliannagounder VK, Hossain MA, Kim JH, et al. Magnetic hydroxyapatite composite nanoparticles for augmented differentiation of MC3T3-E1 cells for bone tissue engineering. Mar Drugs. 2023;21(2):85. doi: 10.3390/md21020085
- Liu TM, Lee EH. Transcriptional regulatory cascades in Runx2-dependent bone development. Tissue Eng Part B Rev. 2013;19(3):254-263. doi: 10.1089/ten.TEB.2012.0527
- Li W, Huang C, Ma T, et al. Low-frequency electromagnetic fields combined with tissue engineering techniques accelerate intervertebral fusion. Stem Cell Res Ther. 2021;12(1):143 doi: 10.1186/s13287-021-02207-x
- Wang Q, Chen B, Cao M, et al. Response of MAPK pathway to iron oxide nanoparticles in vitro treatment promotes osteogenic differentiation of hBMSCs. Biomaterials. 2016;86:11-20. doi: 10.1016/j.biomaterials.2016.02.004
- Gokduman K, Bestepe F, Li L, et al. Dose-, treatment- and time-dependent toxicity of superparamagnetic iron oxide nanoparticles on primary rat hepatocytes. Nanomedicine (Lond). 2018;13(11):1267-1284. doi: 10.2217/nnm-2017-0387
- Jain TK, Reddy MK, Morales MA, et al. Biodistribution, clearance, and biocompatibility of iron oxide magnetic nanoparticles in rats. Mol Pharm. 2008;5(2): 316-327. doi: 10.1021/mp7001285
- Levy M, Luciani N, Alloyeau D, et al. Long term in vivo biotransformation of iron oxide nanoparticles. Biomaterials. 2011;32(16):3988-3999. doi: 10.1016/j.biomaterials.2011.02.031
- Marsell R, Einhorn TA. The biology of fracture healing. Injury. 2011;42(6):551-555. doi: 10.1016/j.injury.2011.03.031
- Yang J, Gao H, Zhang D, et al. Static compressive behavior and material failure mechanism of trabecular tantalum scaffolds fabricated by laser powder bed fusion-based additive manufacturing. Int J Bioprint. 2022;8(1):438. doi: 10.18063/ijb.v8i1.438
- Karageorgiou V, Kaplan D. Porosity of 3D biomaterial scaffolds and osteogenesis. Biomaterials. 2005;26(27): 5474-5491. doi: 10.1016/j.biomaterials.2005.02.002
- Jiang P, Zhang Y, Zhu C, et al. Fe(3)O(4)/BSA particles induce osteogenic differentiation of mesenchymal stem cells under static magnetic field. Acta Biomater. 2016; 46:141-150. doi: 10.1016/j.actbio.2016.09.020
- Chen H, Sun J, Wang Z, et al. Magnetic cell-scaffold interface constructed by superparamagnetic IONP enhanced osteogenesis of adipose-derived stem cells. ACS Appl Mater Interfaces. 2018;10(51):44279-44289 doi: 10.1021/acsami.8b17427
- Yang J, Wu J, Guo Z, et al. Iron oxide nanoparticles combined with static magnetic fields in bone remodeling. Cells. 2022;11(20):3298. doi: 10.3390/cells11203298.