PLA-based bone tissue engineering scaffolds incorporating hydroxyapatite and bioactive glass using digital light processing
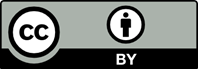
Bone tissue engineering (BTE) aims to repair bone defects using biocompatible materials with tailored geometries and pore structures, providing appropriate mechanical support and control over biodegradation kinetics to promote bone growth. In this study, we utilized digital light processing (DLP) 3D printing to fabricate scaffolds with varying pore sizes using polymer–ceramic slurries composed of polylactic acid (PLA) as the main polymer matrix, incorporated with hydroxyapatite (HA) and bioactive borate glass (BBG) at various ratios. We studied the effect of composition on rheological behavior, printability, mechanical properties, bioactivity, degradation rate, and biocompatibility. While HA increased viscosity and reduced printing accuracy, it also improved mechanical properties and bioactivity. BBG increased the hydrophilicity and shape fidelity of the scaffold. Both HA and BBG enhanced the compressive mechanical properties by reinforcing the polymer matrix with ceramic particles. To study the scaffold’s bioactivity, samples were immersed in simulated body fluid for 4 weeks. Both ceramic additives enhanced the bioactivity of PLA scaffolds, evidenced by the formation of a secondary HA layer on the scaffold surface. Among the scaffolds studied, PLA-BBG exhibited the highest osteocyte viability, followed by PLA-HA and then plain PLA samples. Our findings highlight the potential of DLP 3D printing for the fabrication of tailored polymer–ceramic scaffolds for BTE and other biomedical applications.

- Xue N, Ding X, Huang R, et al. Bone tissue engineering in the treatment of bone defects. Pharmaceuticals. 2022; 15(7):879. doi: 10.3390/ph15070879
- Zhang W, Jiang Z, Chi J, et al. A novel porous butyryl chitin– animal derived hydroxyapatite composite scaffold for cranial bone defect repair. Int J Mol Sci. 2023;24(10):8519. doi: 10.3390/ijms24108519
- Wei H, Cui J, Lin K, Xie J, Wang X. Recent advances in smart stimuli-responsive biomaterials for bone therapeutics and regeneration. Bone Res. 2022;10(1):17. doi: 10.1038/s41413-021-00180-y
- Omar O, Engstrand T, Linder LKB, et al. In situ bone regeneration of large cranial defects using synthetic ceramic implants with a tailored composition and design. Proc Natl Acad Sci U S A. 2020;117(43):26660-26671. doi: 10.1073/pnas.2007635117
- Xu W, Pranovich A, Uppstu P, et al. Novel biorenewable composite of wood polysaccharide and polylactic acid for three dimensional printing. Carbohydr Polym. 2018;187(January):51-58. doi: 10.1016/j.carbpol.2018.01.069
- Gillman CE, Jayasuriya AC. FDA-approved bone grafts and bone graft substitute devices in bone regeneration. Mater Sci Eng C. 2021;130(July):112466. doi: 10.1016/j.msec.2021.112466
- Wang W, Yeung KWK. Bone grafts and biomaterials substitutes for bone defect repair: a review. Bioact Mater. 2017;2(4):224-247. doi: 10.1016/j.bioactmat.2017.05.007
- Fernandez de Grado G, Keller L, Idoux-Gillet Y, et al. Bone substitutes: a review of their characteristics, clinical use, and perspectives for large bone defects management. J Tissue Eng. 2018;9:2041731418776819. doi: 10.1177/2041731418776819
- Oryan A, Alidadi S, Moshiri A. Current concerns regarding healing of bone defects. Hard Tissue. 2013;2(2);13. doi: 10.13172/2050-2303-2-2-374
- Murphy CM. Understanding the effect of mean pore size on cell activity in collagen-glycosaminoglycan scaffolds. Cell Adh Migr. 2010;4(3):377-381. doi: 10.4161/cam.4.3.11747
- Sobral JM, Caridade SG, Sousa RA, Mano JF, Reis RL. Three-dimensional plotted scaffolds with controlled pore size gradients : Effect of scaffold geometry on mechanical performance and cell seeding efficiency. Acta Biomater. 2011;7:1009-1018. doi: 10.1016/j.actbio.2010.11.003
- Abbasi N, Hamlet S, Love RM, Nguyen NT. Porous scaffolds for bone regeneration. J Sci: Adv Mater Devices. 2020;5(1):1-9. doi: 10.1016/j.jsamd.2020.01.007
- Fayyazbakhsh F, Solati-Hashjin M, Keshtkar A, Shokrgozar MA, Dehghan MM, Larijani B. Novel layered double hydroxides-hydroxyapatite/gelatin bone tissue engineering scaffolds: fabrication, characterization, and in vivo study. Mater Sci Eng C. 2017;76:701-714. doi: 10.1016/j.msec.2017.02.172
- Fayyazbakhsh F, Solati-Hashjin M, Keshtkar A, Shokrgozar MA, Dehghan MM, Larijani B. Release behavior and signaling effect of vitamin D3 in layered double hydroxides-hydroxyapatite/gelatin bone tissue engineering scaffold: an in vitro evaluation. Colloids Surf B Biointerfaces. 2017;158:697-708. doi: 10.1016/j.colsurfb.2017.07.004
- Chinnasami H, Dey MK, Devireddy R. Three-dimensional scaffolds for bone tissue engineering. Bioengineering (Basel). 2023;10(7):759. doi: 10.3390/bioengineering10070759
- Adel IM, Elmeligy MF, Elkasabgy NA. Conventional and recent trends of scaffolds fabrication: a superior mode for tissue engineering. Pharmaceutics. 2022;14(2):306. doi: 10.3390/pharmaceutics14020306
- Percival KM, Paul V, Husseini GA. Recent advancements in bone tissue engineering: integrating smart scaffold technologies and bio-responsive systems for enhanced regeneration. Int J Mol Sci. 2024;25(11):6012. doi: 10.3390/ijms25116012
- Nayak VV, Sanjairaj V, Behera RK, et al. Direct inkjet writing of polylactic acid/β‐tricalcium phosphate composites for bone tissue regeneration: a proof‐of‐concept study. J Biomed Mater Res B Appl Biomater. 2024;112(4):e35402. doi: 10.1002/jbm.b.35402
- Ait Said H, Mabroum H, Lahcini M, et al. Manufacturing methods, properties, and potential applications in bone tissue regeneration of hydroxyapatite-chitosan biocomposites: a review. Int J Biol Macromol. 2023;243:125150. doi: 10.1016/j.ijbiomac.2023.125150
- Aytac Z, Dubey N, Daghrery A, et al. Innovations in craniofacial bone and periodontal tissue engineering–from electrospinning to converged fabrication. Int Mater Rev. 2022;67(4):347-384. doi: 10.1080/09506608.2021.1946236
- Germaini MM, Belhabib S, Guessasma S, Deterre R, Corre P, Weiss P. Additive manufacturing of biomaterials for bone tissue engineering – a critical review of the state of the art and new concepts. Prog Mater Sci. 2022;130:100963. doi: 10.1016/j.pmatsci.2022.100963
- Mok SW, Nizak R, Fu SC, et al. From the printer: potential of three-dimensional printing for orthopaedic applications. J Orthop Translat. 2016;6:42-49. doi: 10.1016/j.jot.2016.04.003
- Zadpoor AA. Bone tissue regeneration: the role of scaffold geometry. Biomater Sci. 2015;3(2):231-245. doi: 10.1039/c4bm00291a
- Cheah CM, Chua CK, Leong KF, Cheong CH, Naing MW. Automatic algorithm for generating complex polyhedral scaffold structures for tissue engineering. Tissue Eng. 2004;10(3-4):595-610. doi: 10.1089/107632704323061951
- Chua CK, Leong KF, Cheah CM, Chua SW. Development of a tissue engineering scaffold structure library for rapid prototyping. Part 1: investigation and classification. Int J Adv Manuf Technol. 2003;21(4):291-301. doi: 10.1007/s001700300034
- Jia Z, Xu X, Zhu D, Zheng Y. Design, printing, and engineering of regenerative biomaterials for personalized bone healthcare. Prog Mater Sci. 2023;134:101072. doi: 10.1016/j.pmatsci.2023.101072
- Kadry H, Wadnap S, Xu C, Ahsan F. Digital light processing (DLP) 3D-printing technology and photoreactive polymers in fabrication of modified-release tablets. Eur J Pharm Sci. 2019;135:60-67. doi: 10.1016/j.ejps.2019.05.008
- Mohammed AA, Algahtani MS, Ahmad MZ, Ahmad J, Kotta S. 3D printing in medicine: technology overview and drug delivery applications. Ann 3D Printed Med. 2021;4:100037. doi: 10.1016/j.stlm.2021.100037
- Lv Y, Wang B, Liu G, et al. Metal material, properties and design methods of porous biomedical scaffolds for additive manufacturing: a review. Front Bioeng Biotechnol. 2021;9:641130. doi: 10.3389/fbioe.2021.641130
- Zeng Y, Yan Y, Yan H, et al. 3D printing of hydroxyapatite scaffolds with good mechanical and biocompatible properties by digital light processing. J Mater Sci. 2018;53(9):6291-6301. doi: 10.1007/s10853-018-1992-2
- İyibilgin O, Gepek E. Additive manufacturing technologies and its future in industrial applications. Int J Integr Eng. 2021;13(5):245-257. doi: 10.30880/ijie.2021.13.07.028
- Arifin N, Sudin I, Ngadiman NHA, Ishak MSA. A comprehensive review of biopolymer fabrication in additive manufacturing processing for 3D-tissue-engineering scaffolds. Polymers (Basel). 2022;14(10):2119. doi: 10.3390/polym14102119
- Yao Q, Cosme JGL, Xu T, et al. Three dimensional electrospun PCL/PLA blend nanofibrous scaffolds with significantly improved stem cells osteogenic differentiation and cranial bone formation. Biomaterials. 2017;115:115-127. doi: 10.1016/j.biomaterials.2016.11.018
- Fayyazbakhsh F, Khayat MJ, Sadler C, Day D, Huang YW, Leu MC. 3D-printed hydrogels dressings with bioactive borate glass for continuous hydration and treatment of second-degree burns. Int J Bioprint. 2023;9(6):0118. doi: 10.36922/ijb.0118
- Caroline M, Kolan K, Li W, Semon J, Day D, Leu M. 3D bioprinting of stem cells and polymer/bioactive glass composite scaffolds for bone tissue engineering. Int J Bioprint. 2017;3(1):53-63. doi: 10.18063/IJB.2017.01.005
- Fayyazbakhsh F, Solati-Hashjin M, Shokrgozar MA, et al. Biological evaluation of a novel tissue engineering scaffold of Layered Double Hydroxides (LDHs). Key Eng Mater. 2012;493-494:902-908. doi: 10.4028/www.scientific.net/KEM.493-494.902
- Zhang Q, Mochalin VN, Neitzel I, et al. Mechanical properties and biomineralization of multifunctional nanodiamond- PLLA composites for bone tissue engineering. Biomaterials. 2012;33(20):5067-5075. doi: 10.1016/j.biomaterials.2012.03.063
- Zhang Q, Mochalin VN, Neitzel I, et al. Fluorescent PLLA-nanodiamond composites for bone tissue engineering. Biomaterials. 2011;32(1):87-94. doi: 10.1016/j.biomaterials.2010.08.090
- Siavashani AZ, Nazarpak MH, Bakhsh FF, Toliyat T, Solati- Hashjin M. Preparation of mesoporous silica nanoparticles for insulin drug delivery. Adv Mat Res. 2014;829:251-257. doi: 10.4028/www.scientific.net/amr.829.251
- Azari Z, Kermani F, Mollazadeh S, et al. Fabrication and characterization of cobalt- and copper-doped mesoporous borate bioactive glasses for potential applications in tissue engineering. Ceram Int. 2023;49(23):38773-38788. doi: 10.1016/j.ceramint.2023.09.214
- Liang R, Gu Y, Wu Y, Bunpetch V, Zhang S. Lithography-based 3D bioprinting and bioinks for bone repair and regeneration. ACS Biomater Sci Eng. 2021;7(3):806-816. doi: 10.1021/acsbiomaterials.9b01818
- Zhang B, Xing F, Chen L, et al. DLP fabrication of customized porous bioceramics with osteoinduction ability for remote isolation bone regeneration. Biomater Adv. 2023; 145:213261. doi: 10.1016/j.bioadv.2022.213261
- Wang Y, Chen S, Liang H, Liu Y, Bai J, Wang M. Digital light processing (DLP) of nano biphasic calcium phosphate bioceramic for making bone tissue engineering scaffolds. Ceram Int. 2022;48(19):27681-27692. doi: 10.1016/j.ceramint.2022.06.067
- Ghahri T, Salehi Z, Aghajanpour S, et al. Development of osteon-like scaffold-cell construct by quadruple coaxial extrusion-based 3D bioprinting of nanocomposite hydrogel. Biomater Adv. 2023;145:213254. doi: 10.1016/j.bioadv.2022.213254
- Alam F, Shukla VR, Varadarajan KM, Kumar S. Microarchitected 3D printed polylactic acid (PLA) nanocomposite scaffolds for biomedical applications. J Mech Behav Biomed Mater. 2020;103:103576. doi: 10.1016/j.jmbbm.2019.103576
- Li N, Li Y, Liu S. Rapid prototyping of continuous carbon fiber reinforced polylactic acid composites by 3D printing. J Mater Process Technol. 2016;238:218-225. doi: 10.1016/j.jmatprotec.2016.07.025
- Bernardo MP, da Silva BCR, Hamouda AEI, et al. PLA/ Hydroxyapatite scaffolds exhibit in vitro immunological inertness and promote robust osteogenic differentiation of human mesenchymal stem cells without osteogenic stimuli. Sci Rep. 2022;12(1):1-15. doi: 10.1038/s41598-022-05207-w
- Horvat G, Rožanc J, Maver U, Finšgar M, Knez Ž, Novak Z. Reinforcing ethyl cellulose aerogels with poly(lactic acid) for enhanced bone regeneration. Cellulose. 2024;31:4421-4439. doi: 10.1007/s10570-024-05905-w
- Hassanajili S, Karami-Pour A, Oryan A, Talaei-Khozani T. Preparation and characterization of PLA/PCL/HA composite scaffolds using indirect 3D printing for bone tissue engineering. Mater Sci Eng C Mater Biol Appl. 2019;104:109960. doi: 10.1016/j.msec.2019.109960
- Türk S, Altınsoy İ, ÇelebiEfe G, Ipek M, Özacar M, Bindal C. Microwave–assisted biomimetic synthesis of hydroxyapatite using different sources of calcium. Mater Sci Eng C. 2017;76:528-535. doi: 10.1016/j.msec.2017.03.116
- Rajendran AK, Anthraper MSJ, Hwang NS, Rangasamy J. Osteogenesis and angiogenesis promoting bioactive ceramics. Mater Sci Eng R: Rep. 2024;159:100801. doi: 10.1016/j.mser.2024.100801
- Abodunrin OD, El Mabrouk K, Bricha M. A review on borate bioactive glasses (BBG): effect of doping elements, degradation, and applications. J Mater Chem B. 2023;11(5):955-973. doi: 10.1039/d2tb02505a
- Mistry S, Kundu D, Datta S, Basu D. Comparison of bioactive glass coated and hydroxyapatite coated titanium dental implants in the human jaw bone. Aust Dent J. 2011;56(1):68-75. doi: 10.1111/j.1834-7819.2010.01305.x
- Aslam AA, Akram J, Mehmood RA, et al. Boron-based bioactive glasses: properties, processing, characterization and applications. Ceram Int. 2023;49(12):19595-19605. doi: 10.1016/j.ceramint.2023.03.164
- Han J, Wu J, Xiang X, et al. Biodegradable BBG/PCL composite scaffolds fabricated by selective laser sintering for directed regeneration of critical-sized bone defects. Mater Des. 2023;225:111543. doi: 10.1016/j.matdes.2022.111543
- Turnbull G, Clarke J, Picard F, et al. 3D bioactive composite scaffolds for bone tissue engineering. Bioact Mater. 2018;3(3):278-314. doi: 10.1016/j.bioactmat.2017.10.001
- Ege D, Zheng K, Boccaccini AR. Borate bioactive glasses (BBG): bone regeneration, wound healing applications, and future directions. ACS Appl Bio Mater. 2022;5(8):3608-3622. doi: 10.1021/acsabm.2c00384
- Fayyazbakhsh F, Tusar HM, Huang YW, Leu CM. Effect of bioactive borate glass on printability and physical properties of hydrogels. Mater Sci Addit Manuf. 2024;3(1):2845. doi: 10.36922/msam.2845
- Lin YM, Chen H, Lin CH, Huang PJ, Lee SY. Development of polycaprolactone/hydroxyapatite composite resin for 405 nm digital light projection 3D printing. Rapid Prototyp J. 2020;26(5):951-958. doi: 10.1108/RPJ-06-2019-0166
- Song P, Li M, Zhang B, et al. DLP fabricating of precision GelMA/HAp porous composite scaffold for bone tissue engineering application. Compos B Eng. 2022; 244:110163. doi: 10.1016/j.compositesb.2022.110163
- Gepek E, Fayyazbakhsh F, Iyibilgin O, Suliandziga L, Leu M. PLA-HA/BBG composite scaffolds fabricated by digital-light-processing 3D printing for cranial bone regeneration. In: Proceedings of the 35th Annual International Solid Freeform Fabrication Symposium – An Additive Manufacturing Conference. University of Texas at Austin; 2024. doi: 10.26153/tsw/58069
- Vieira BM, Costa M de O, Thiré RM da S, Araujo AC. Comparison of the porosity of scaffolds manufactured by two additive manufacturing technologies: SLA and FDM. In: 24th ABCM International Congress of Mechanical Engineering; 2018.
- Kokubo T, Takadama H. How useful is SBF in predicting in vivo bone bioactivity? Biomaterials. 2006;27(15): 2907-2915. doi: 10.1016/j.biomaterials.2006.01.017
- Zhang J, Huang D, Liu S, et al. Zirconia toughened hydroxyapatite biocomposite formed by a DLP 3D printing process for potential bone tissue engineering. Mater Sci Eng C. 2019;105:110054. doi: 10.1016/j.msec.2019.110054
- Xu X, Zhou S, Wu J, Zhang C, Liu X. Inter-particle interactions of alumina powders in UV-curable suspensions for DLP stereolithography and its effect on rheology, solid loading, and self-leveling behavior. J Eur Ceram Soc. 2021;41(4):2763-2774. doi: 10.1016/j.jeurceramsoc.2020.12.004
- Wu D, Spanou A, Diez-Escudero A, Persson C. 3D-printed PLA/HA composite structures as synthetic trabecular bone: a feasibility study using fused deposition modeling. J Mech Behav Biomed Mater. 2020;103:103608. doi: 10.1016/j.jmbbm.2019.103608
- Tanase-Opedal M, Espinosa E, Rodríguez A, Chinga- Carrasco G. Lignin: a biopolymer from forestry biomass for biocomposites and 3D printing. Materials. 2019; 12(18):3006. doi: 10.3390/ma12183006
- Nim B, Rahayu SS, Thananukul K, et al. Sizing down and functionalizing polylactide (PLA) resin for synthesis of PLA ‑based polyurethanes for use in biomedical applications. Sci Rep. 2023;13(1):2284. doi: 10.1038/s41598-023-29496-x
- Liu S, Zheng Y. Preparation and characterization of a novel polylactic acid / hydroxyapatite composite scaffold with biomimetic micro-nano fi brous porous structure. J Mater Sci Mater Med. 2020;31(8):74. doi: 10.1007/s10856-020-06415-4
- Mofokeng JP, Luyt AS, Tábi T, Kovács J. Comparison of injection moulded, natural fibre-reinforced composites with PP and PLA as matrices. J Thermoplastic Compos Mater. 2012;25(8):927-948. doi: 10.1177/0892705711423291
- González-Benito J, Zuñiga-Prado S, Najera J, Olmos D. Non-woven fibrous polylactic acid/hydroxyapatite nanocomposites obtained via solution blow spinning: morphology, thermal and mechanical behavior. Nanomaterials. 2024;14(2):196. doi: 10.3390/nano14020196
- Alam K, Gafur MA, Md. Hasan Mahmud KAS. Chemical characteristics of hydroxyapatite from oyster shell by thermo-chemical process. Int J Innov Res Sci Eng Technol. 2015;04(07):5039-5047. doi: 10.15680/IJIRSET.2015.0407002
- Umapathi R, Lim JH. Effect of infill pattern and lattice structure on the mechanical properties of 3d printed metal polylactide filament. J Phys Conf Ser. 2021;2120(1):012019. doi: 10.1088/1742-6596/2120/1/012019
- Vu MC, Jeong T, Kim J, Choi WK, Kim DH, Kim S. 3D printing of copper particles and poly(methyl methacrylate) beads containing poly(lactic acid) composites for enhancing thermomechanical properties. J Appl Polym Sci. 2021;138(5):1-10. doi: 10.1002/app.49776
- Ruz-Cruz MA, Herrera-Franco PJ, Flores-Johnson EA, Moreno-Chulim MV, Galera-Manzano LM, Valadez- González A. Thermal and mechanical properties of PLA-based multiscale cellulosic biocomposites. J Mater Res Technol. 2022;18:485-495. doi: 10.1016/j.jmrt.2022.02.072
- Inkinen S, Hakkarainen M, Albertsson AC, Södergård A. From lactic acid to poly(lactic acid) (PLA): characterization and analysis of PLA and its precursors. Biomacromolecules. 2011;12(3):523-532. doi: 10.1021/bm101302t
- Akindoyo JO, Beg MDH, Ghazali S, Heim HP, Feldmann M. Impact modified PLA-hydroxyapatite composites – thermo-mechanical properties. Compos Part A Appl Sci Manuf. 2018;107:326-333. doi: 10.1016/j.compositesa.2018.01.017
- El-Hajje A, Kolos EC, Wang JK, et al. Physical and mechanical characterisation of 3D-printed porous titanium for biomedical applications. J Mater Sci Mater Med. 2014;25(11):2471-2480. doi: 10.1007/s10856-014-5277-2
- Koushik TM, Miller CM, Antunes E. Bone tissue engineering scaffolds: function of multi-material hierarchically structured scaffolds. Adv Healthc Mater. 2023;12(9):e2202766. doi: 10.1002/adhm.202202766
- Liu K, Zhou C, Hu J, et al. Fabrication of barium titanate ceramics via digital light processing 3D printing by using high refractive index monomer. J Eur Ceram Soc. 2021;41(12):5909-5917. doi: 10.1016/j.jeurceramsoc.2021.04.044
- Liang H, Wang Y, Chen S, Liu Y, Liu Z, Bai J. Nano-hydroxyapatite bone scaffolds with different porous structures processed by digital light processing 3D printing. Int J Bioprint. 2022;8(1):198-210. doi: 10.18063/IJB.V8I1.502
- Zhang Q, Weng S, Hamel CM, et al. Design for the reduction of volume shrinkage-induced distortion in digital light processing 3D printing. Extreme Mech Lett. 2021;48:101403. doi: doi: 10.1016/j.eml.2021.101403
- Jian Y, He Y, Jiang T, Li C, Yang W, Nie J. Polymerization shrinkage of (Meth) acrylate determined by reflective laser beam scanning. J Polym Sci B Polym Phys. 2012;50:923-928. doi: 10.1002/polb.23086
- Jaidev LR, Chatterjee K. Surface functionalization of 3D printed polymer scaffolds to augment stem cell response. Mater Des. 2019;161:44-54. doi: 10.1016/j.matdes.2018.11.018
- Mu M, Liu S, DeFlorio W, et al. Influence of surface roughness, nanostructure, and wetting on bacterial adhesion. Langmuir. 2023;39(15):5426-5439. doi: 10.1021/acs.langmuir.3c00091
- Subramaniyan M, Karuppan S, Helaili S, Ahmad I. Structural, mechanical, and in-vitro characterization of hydroxyapatite loaded PLA composites. J Mol Struct. 2024;1306:137862. doi: 10.1016/j.molstruc.2024.137862
- Gerhardt LC, Boccaccini AR. Bioactive glass and glass-ceramic scaffolds for bone tissue engineering. Materials. 2010;3(7):3867-3910. doi: 10.3390/ma3073867
- Darghiasi SF, Farazin A, Ghazali HS. Design of bone scaffolds with calcium phosphate and its derivatives by 3D printing: a review. J Mech Behav Biomed Mater. 2024;151:106391. doi: 10.1016/j.jmbbm.2024.106391
- Abushahba F, Tuukkanen J, Aalto-Setälä L, Miinalainen I, Hupa L, Närhi TO. Effect of bioactive glass air-abrasion on the wettability and osteoblast proliferation on sandblasted and acid-etched titanium surfaces. Eur J Oral Sci. 2020;128(2):160-169. doi: 10.1111/eos.12683
- Nazeer MA, Onder OC, Sevgili I, Yilgor E, Kavakli IH, Yilgor I. 3D printed poly(lactic acid) scaffolds modified with chitosan and hydroxyapatite for bone repair applications. Mater Today Commun. 2020;25:101515. doi: 10.1016/j.mtcomm.2020.101515
- Donate R, Monzón M, Alemán-Domínguez ME. Additive manufacturing of PLA-based scaffolds intended for bone regeneration and strategies to improve their biological properties. E-Polymers. 2020;20(1):571-599. doi: 10.1515/epoly-2020-0046
- Manzoor F, Golbang A, Jindal S, et al. 3D printed PEEK/ HA composites for bone tissue engineering applications: effect of material formulation on mechanical performance and bioactive potential. J Mech Behav Biomed Mater. 2021;121:104601. doi: 10.1016/j.jmbbm.2021.104601
- Amaravathy P, Kumar TSSS. Bioactivity enhancement by Sr doped Zn-Ca-P coatings on biomedical magnesium alloy. J Mag Alloys. 2019;7(4):584-596. doi: 10.1016/j.jma.2019.05.014
- Hadjittofis E, Vargas SM, Litster JD, Campbell KLS. Exploring the role of crystal habit in the Ostwald rule of stages. Proc Math Phys Eng Sci. 2022;478(2258):20210601. doi: 10.1098/rspa.2021.0601
- Guo X, Liu L, Wang W, Zhang J, Wang Y, Yu SH. Controlled crystallization of hierarchical and porous calcium carbonate crystals using polypeptide type block copolymer as crystal growth modifier in a mixed solution. CrystEngComm. 2011;13(6):2054-2061. doi: 10.1039/c0ce00202j
- Cheng H, Zhang X, Song H. Morphological investigation of calcium carbonate during ammonification-carbonization process of low concentration calcium solution. J Nanomater. 2014;503696:1-7. doi: 10.1155/2014/503696
- Liu H, Yazici H, Ergun C, Webster TJ, Bermek H. An in vitro evaluation of the Ca/P ratio for the cytocompatibility of nano-to-micron particulate calcium phosphates for bone regeneration. Acta Biomater. 2008;4(5):1472-1479. doi: 10.1016/j.actbio.2008.02.025
- He YH, Zhang YQ, Jiang YH, Zhou R. Microstructure evolution and enhanced bioactivity of Ti-Nb-Zr alloy by bioactive hydroxyapatite fabricated: via spark plasma sintering. RSC Adv. 2016;6(103):100939-100953. doi: 10.1039/c6ra22986g
- Fiume E, Tulyaganov D, Ubertalli G, Verné E, Baino F. Dolomite-foamed bioactive silicate scaffolds for bone tissue repair. Materials. 2020;13(3):1-13. doi: 10.3390/ma13030628
- Ma P, Wu W, Wei Y, Ren L, Lin S, Wu J. Biomimetic gelatin/chitosan/polyvinyl alcohol/nano-hydroxyapatite scaffolds for bone tissue engineering. Mater Des. 2021; 207:109865. doi: 10.1016/j.matdes.2021.109865
- Wang Q, Wang Q, Wan C. Preparation and evaluation of a biomimetic scaffold with porosity gradients in vitro. An Acad Bras Cienc. 2012;84(1):9-16. doi: 10.1590/S0001-37652012005000003
- Mohammadkhah M, Marinkovic D, Zehn M, Checa S. A review on computer modeling of bone piezoelectricity and its application to bone adaptation and regeneration. Bone. 2019;127:544-555. doi: 10.1016/j.bone.2019.07.024
- Zhang K, Alaohali A, Sawangboon N, Sharpe PT, Brauer DS, Gentleman E. A comparison of lithium-substituted phosphate and borate bioactive glasses for mineralised tissue repair. Dent Mater. 2019;35(6):919-927. doi: 10.1016/j.dental.2019.03.008
- Marquardt LM, Day D, Sakiyama-Elbert SE, Harkins AB. Effects of borate-based bioactive glass on neuron viability and neurite extension. J Biomed Mater Res A. 2014;102(8):2767-2775. doi: 10.1002/jbm.a.34944
- Haro Durand LA, Vargas GE, Romero NM, et al. Angiogenic effects of ionic dissolution products released from a boron-doped 45S5 bioactive glass. J Mater Chem B. 2015;3(6):1142-1148. doi: 10.1039/c4tb01840k
- Shafaghi R, Rodriguez O, Phull S, et al. Effect of TiO2 doping on degradation rate, microstructure and strength of borate bioactive glass scaffolds. Mater Sci Eng C. 2020;107:110351. doi: 10.1016/j.msec.2019.110351
- Decker S, Arango-Ospina M, Rehder F, et al. In vitro and in vivo impact of the ionic dissolution products of boron-doped bioactive silicate glasses on cell viability, osteogenesis and angiogenesis. Sci Rep. 2022;12(1):8510. doi: 10.1038/s41598-022-12430-y
- Luo SH, Xiao W, Wei XJ, et al. In vitro evaluation of cytotoxicity of silver-containing borate bioactive glass. J Biomed Mater Res B Appl Biomater. 2010;95B(2):441-448. doi: 10.1002/jbm.b.31735