Development of a high-precision 3D bioprinter system using a screw-based dispenser for microextrusion
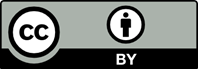
3D bioprinting creates biological structures by layering bioinks with living cells or biomaterials. Microextrusion, a type of 3D bioprinting, uses pneumatic, piston, or screw methods to extrude bioink precisely. The reliability of 3D bioprinting depends on bioink characteristics, printing conditions, and printer accuracy. Thus, a 3D bioprinter that effectively controls these factors is essential to facilitate 3D bioprinting. In this study, we developed a high-precision 3D bioprinter system (HP-BPS) with a high-accuracy 3D plotting system and a screw-based dispenser. Evaluation of reducers installed on the X- and Y-axis driving systems decreased motion error by up to 97%. Geometric errors of the HP-BPS were measured using a laser interferometry system. Through the application of iterative position error compensation techniques, a position accuracy within ±2.0 μm was achieved. In specific carboxymethyl cellulose concentrations (15% and 20%), the HP-BPS was able to produce uncollapsed bioink struts. The HP-BPS successfully fabricated 1 × 1 mm2 bioscaffolds with 0.2 mm struts by design of experiments and response surface methodology. These results suggest the potential of the HP-BPS for various tissue engineering applications in soft tissue construction, such as skin and blood vessels.

- Ambrosi A, Pumera M. 3D-printing technologies for electrochemical applications. Chem Soc Rev. 2016;45(10):2740-2755. doi: 10.1039/C5CS00714C
- Ho CMB, Ng SH, Yoon Y. A review on 3D printed bioimplants. Int J Precis Eng Manuf. 2015;16:1035-1046. doi: 10.1007/s12541-015-0134-x
- Kjar A, Huang Y. Application of micro-scale 3D printing in pharmaceutics. Pharmaceutics. 2019;11(8):390. doi: 10.3390/pharmaceutics11080390
- Norman J, Madurawe RD, Moore CM, et al. A new chapter in pharmaceutical manufacturing: 3D-printed drug products. Adv Drug Deliv Rev. 2017;108:39-50. doi: 10.1016/j.addr.2016.03.001
- Stansbury JW, Idacavage MJ. 3D printing with polymers: challenges among expanding options and opportunities. Dental Mater. 2016;32(1):54-64. doi: 10.1016/j.dental.2015.09.018
- Park S, Lee J, Kim J, et al. Osseointegrative and immunomodulative 3D-printing Ti6Al4V-based implants embedded with biogenic hydroxyapatite. Mater Des. 2024;240:112822. doi: 10.1016/j.matdes.2024.112822
- De Mori A, Peña Fernández M, Blunn G, et al. 3D printing and electrospinning of composite hydrogels for cartilage and bone tissue engineering. Polymers. 2018;10(3):285. doi: 10.3390/polym10030285
- Cho E, Kim JE, Lee J, et al. Development of 3D printable calcium phosphate cement scaffolds with cockle shell powders. Materials. 2023;16(18):6154. doi: 10.3390/ma16186154
- Liu Y, Hamid Q, Snyder J, et al. Evaluating fabrication feasibility and biomedical application potential of in situ 3D printing technology. Rapid Prototyping J. 2016;22(6):947-955. doi: 10.1108/RPJ-07-2015-0090
- Lee J, Park S, Shin B, et al. Latent stem cell-stimulating radially aligned electrospun nanofibrous patches for chronic tympanic membrane perforation therapy. Acta Biomater. 2024;188:212-222. doi: 10.1016/j.actbio.2024.09.019
- Ventola CL. Medical applications for 3D printing: current and projected uses. Pharm Ther. 2014;39(10):704.
- Panda S, Hajra S, Mistewicz K, et al. A focused review on three-dimensional bioprinting technology for artificial organ fabrication. Biomater Sci. 2022;10(18):5054-5080. doi: 10.1039/D2BM00797E
- Ferris CJ, Gilmore KJ, Beirne S, et al. Bio-ink for on-demand printing of living cells. Biomater Sci. 2013;1(2):224-230. doi: 10.1039/C2BM00114D
- Gu Z, Fu J, Lin H, et al. Development of 3D bioprinting: from printing methods to biomedical applications. Asian J Pharma Sci. 2020;15(5):529-557. doi: 10.1016/j.ajps.2019.11.003
- Byambaa B, Annabi N, Yue K, et al. Bioprinted osteogenic and vasculogenic patterns for engineering 3D bone tissue. Adv Healthc Mater. 2017;6(16):1700015. doi: 10.1002/adhm.201700015
- Ashammakhi N, Ahadian S, Xu C, et al. Bioinks and bioprinting technologies to make heterogeneous and biomimetic tissue constructs. Mater Today Bio. 2019;1:100008. doi: 10.1016/j.mtbio.2019.100008
- Trauner KB. The emerging role of 3D printing in arthroplasty and orthopedics. J Arthroplasty. 2018;33(8):2352-2354. doi: 10.1016/j.arth.2018.02.033
- Hu G, Bodaghi M. Direct fused deposition modeling 4D printing and programming of thermoresponsive shape memory polymers with autonomous 2D-to-3D shape transformations. Adv Eng Mater. 2023;25(19):2300334. doi: 10.1002/adem.202300334
- DeBari MK, Keyser MN, Bai MA, et al. 3D printing with silk: considerations and applications. Connect Tissue Res. 2020;61(2):163-173. doi: 10.1080/03008207.2018.1553959
- Lee J, Lee S, Lim JW, et al. Development of plum seed-derived carboxymethylcellulose bioink for 3D bioprinting. Polymers. 2023;15(23):4473. doi: 10.3390/polym15234473
- Fu Z, Naghieh S, Xu C, et al. Printability in extrusion bioprinting. Biofabrication. 2021;13(3):033001. doi: 10.1088/1758-5090/abe7ab
- Melchels FP, Domingos MA, Klein TJ, et al. Additive manufacturing of tissues and organs. Prog Polymer Sci. 2012;37(8):1079-1104. doi: 10.1016/j.progpolymsci.2011.11.007
- Moroni L, Burdick JA, Highley C, et al. Biofabrication strategies for 3D in vitro models and regenerative medicine. Nat Rev Mater. 2018;3(5):21-37. doi: 10.1038/s41578-018-0006-y
- Gillispie G, Prim P, Copus J, et al. Assessment methodologies for extrusion-based bioink printability. Biofabrication. 2020;12(2):022003. doi: 10.1088/1758-5090/ab6f0d
- Paxton N, Smolan W, Böck T, et al. Proposal to assess printability of bioinks for extrusion-based bioprinting and evaluation of rheological properties governing bioprintability. Biofabrication. 2017;9(4):044107. doi: 10.1088/1758-5090/aa8dd8
- Karamimoghadam M, Dezaki ML, Zolfagharian A, et al. Influence of post-processing CO2 laser cutting and FFF 3D printing parameters on the surface morphology of PLAs:statistical modelling and RSM optimisation. Int J Lightweight Mater Manuf. 2023;6(2):285-295. doi: 10.1016/j.ijlmm.2023.01.004
- Madić M, Radovanović M, Manić M, et al. Optimization of CO2 laser cutting process using taguchi and dual response surface methodology. Tribol Ind. 2014;36(3):236.
- Moradi M, Karami Moghadam M, Shamsborhan M, et al. Post-processing of FDM 3D-printed polylactic acid parts by laser beam cutting. Polymers. 2020;12(3):550. doi: 10.3390/polym12030550
- Lee S, Son M, Lee J, et al. Computational fluid dynamics analysis and empirical evaluation of carboxymethylcellulose/ alginate 3d bioprinting inks for screw-based microextrusion. Polymers. 2024;16(8):1137. doi: 10.3390/polym16081137
- Tripathi S, Mandal SS, Bauri S, et al. 3D bioprinting and its innovative approach for biomedical applications. MedComm. 2023;4(1):e194. doi: 10.1002/mco2.194
- Cooke ME, Rosenzweig DH. The rheology of direct and suspended extrusion bioprinting. APL Bioeng. 2021;5(1):011502. doi: 10.1063/5.0031475
- Ozbolat IT, Hospodiuk M. Current advances and future perspectives in extrusion-based bioprinting. Biomaterials. 2016;76:321-343. doi: 10.1016/j.biomaterials.2015.10.076
- Hölzl K, Lin S, Tytgat L, et al. Bioink properties before, during and after 3D bioprinting. Biofabrication. 2016;8(3):032002. doi: 10.1088/1758-5090/8/3/032002
- Ning L, Chen X. A brief review of extrusion‐based tissue scaffold bio‐printing. Biotechnol J. 2017;12(8):1600671. doi: 10.1002/biot.201600671
- Derakhshanfar S, Mbeleck R, Xu K, et al. 3D bioprinting for biomedical devices and tissue engineering: a review of recent trends and advances. Bioactive Mater. 2018;3(2):144-156. doi: 10.1016/j.bioactmat.2017.11.008
- Kim SH, Park SJ, Xu B, et al. Development of polycaprolactone grafts with improved physical properties and body stability using a screw extrusion-type 3D bioprinter. Int J Bioprint. 2022;9(2):652. doi: 10.18063/ijb.v9i2.652
- Zhang J, Wehrle E, Rubert M, et al. 3D bioprinting of human tissues: biofabrication, bioinks, and bioreactors. Int J Mol Sci. 2021;22(8):3971. doi: 10.3390/ijms22083971
- Fang H, Xu J, Ma H, et al. Functional materials of 3D bioprinting for wound dressings and skin tissue engineering applications: a review. Int J Bioprint. 2023;9(5):757. doi: 10.18063/ijb.757
- Pavlychev AA, Avrunin AS, Vinogradov AS, et al. Local electronic structure and nanolevel hierarchical organization of bone tissue: theory and NEXAFS study. Nanotechnology. 2016;27(50):504002. doi: 10.1088/0957-4484/27/50/504002
- Sun X, Jiao X, Yang X, et al. 3D bioprinting of osteon-mimetic scaffolds with hierarchical microchannels for vascularized bone tissue regeneration. Biofabrication. 2022;14(3):035008. doi: 10.1088/1758-5090/ac6700
- Singh A, Shiekh PA, Das M, et al. Aligned chitosan-gelatin cryogel-filled polyurethane nerve guidance channel for neural tissue engineering: fabrication, characterization, and in vitro evaluation. Biomacromolecules. 2018;20(2):662-673. doi: 10.1021/acs.biomac.8b01308
- Huang L, Gao J, Wang H, et al. Fabrication of 3D scaffolds displaying biochemical gradients along longitudinally oriented microchannels for neural tissue engineering. ACS Appl Mater Interfaces. 2020;12(43):48380-48394. doi: 10.1021/acsami.0c15185
- Tisler M, Alkmin S, Chang H, et al. Analysis of fibroblast migration dynamics in idiopathic pulmonary fibrosis using image-based scaffolds of the lung extracellular matrix. Am J Physiol Lung Cell Mol Physiol. 2020;318(2):L276-L286. doi: 10.1152/ajplung.00087.2019
- Isik M, Karakaya E, Arslan TS, et al. 3D printing of extracellular matrix‐based multicomponent, all‐natural, highly elastic, and functional materials toward vascular tissue engineering. Adv Healthc Mater. 2023;12(20):2203044. doi: 10.1002/adhm.202203044
- Ngo TD, Kashani A, Imbalzano G, et al. Additive manufacturing (3D printing): a review of materials, methods, applications and challenges. Compos B: Eng. 2018;143:172-196. doi: 10.1016/j.compositesb.2018.02.012
- Kolesky DB, Truby RL, Gladman AS, et al. 3D bioprinting of vascularized, heterogeneous cell‐laden tissue constructs. Adv Mater. 2014;26(19):3124-3130. doi: 10.1002/adma.201305506
- Hospodiuk M, Dey M, Sosnoski D, et al. The bioink: a comprehensive review on bioprintable materials. Biotechnol Adv. 2017;35(2):217-239. doi: 10.1016/j.biotechadv.2016.12.006
- Ramesh S, Harrysson OL, Rao PK, et al. Extrusion bioprinting: recent progress, challenges, and future opportunities. Bioprinting. 2021;21:e00116. doi: 10.1016/j.bprint.2020.e00116
- Okafor AC, Ertekin YM. Vertical machining center accuracy characterization using laser interferometer: part 1. Linear positional errors. J Mater Process Technol. 2000;105(3):394-406. doi: 10.1016/S0924-0136(00)00661-0
- Lee J, Lee H, Yang S. Total measurement of geometric errors of a three-axis machine tool by developing a hybrid technique. Int J Precis Eng Manuf. 2016;17:427-432. doi: 10.1007/s12541-016-0053-5
- Schwenke H, Knapp W, Haitjema H, et al. Geometric error measurement and compensation of machines—an update. CIRP Annals. 2018;57(2):660-675. doi: 10.1016/j.cirp.2008.09.008
- ISO BS. Test Code for Machine Tools–Part 1: Geometric Accuracy of Machines Operating Under No-Load or Quasi- Static Conditions. ISO230-1; 2012.
- International Organization for Standardization. Test Code for Machine Tools: Part 2: Determination of Accuracy and Repeatability of Positioning Numerically Controlled Axes. International Organization for Standardization; 2006.
- Lee H, Chen J, Pan S, et al. Relationship between ISO 230- 2/-6 test results and positioning accuracy of machine tools using LaserTRACER. Appl Sci. 2016;6(4):105. doi: 10.3390/app6040105
- Jain P, Kathuria H, Ramakrishna S, et al. In situ bioprinting: process, bioinks, and applications. ACS Appl Bio Mater. 2024;7(12):7987-8007. doi: 10.1021/acsabm.3c01303
- Panwar A, Tan LP. Current status of bioinks for micro-extrusion-based 3D bioprinting. Molecules. 2016; 21(6):685. doi: 10.3390/molecules21060685
- Leng J, Wu J, Chen N, et al. The development of a conical screw-based extrusion deposition system and its application in fused deposition modeling with thermoplastic polyurethane. Rapid Prototyping J. 2020;26(2): 409-417. doi: 10.1108/RPJ-05-2019-0139
- Wang C, Hu C, Cheng H, et al. A programmable handheld extrusion-based bioprinting platform for in situ skin wounds dressing: balance mobility and customizability. Adv Sci. 2024;11:2405823. doi: 10.1002/advs.202405823
- Derossi A, Paolillo M, Caporizzi R, et al. Extending the 3D food printing tests at high speed. Material deposition and effect of non-printing movements on the final quality of printed structures. J Food Eng. 2020;275:109865. doi: 10.1016/j.jfoodeng.2019.109865
- Alotaibi AM, Alnawmasi JS, Alshammari NA, et al. Industrial dye absorption and elimination from aqueous solutions through bio-composite construction of an organic framework encased in food-grade algae and alginate: adsorption isotherm, kinetics, thermodynamics, and optimization by Box-Behnken design. Int J Biol Macromol. 2024;274:133442. doi: 10.1016/j.ijbiomac.2024.133442
- Wei Q, An Y, Zhao X, et al. Optimal design of multi-biomaterials mixed extrusion nozzle for 3D bioprinting considering cell activity. Virtual Phys Prototyping. 2025;20(1):e2438897. doi: 10.1080/17452759.2024.2438897
- Ning L, Yang B, Mohabatpour F, et al. Process-induced cell damage: pneumatic versus screw-driven bioprinting. Biofabrication. 2020;12(2):025011. doi: 10.1088/1758-5090/ab5f53
- Aljohani W, Ullah MW, Zhang X, et al. Bioprinting and its applications in tissue engineering and regenerative medicine. Int J Biol Macromol. 2018;107: 261-275. doi: 10.1016/j.ijbiomac.2017.08.171
- Varma MV, Kandasubramanian B, Ibrahim SM. 3D printed scaffolds for biomedical applications. Mater Chem Phys. 2020;255:123642. doi: 10.1016/j.matchemphys.2020.123642
- Wang P, Sun Y, Shi X, et al. 3D printing of tissue engineering scaffolds: a focus on vascular regeneration. Bio-design Manufact. 2021;4(2):344-378. doi: 10.1007/s42242-020-00109-0