3D-printed microfluidic cell culture devices and hydrogel integration: Trends, challenges, and solutions
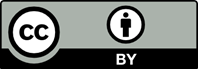
Three-dimensional (3D) cell cultures are increasingly being used in a variety of contexts (e.g., drug discovery, disease modeling, and tissue engineering), as they offer the potential to increase physiological relevance compared to traditional monolayer cultures, while simultaneously reducing cost and time compared to in vivo models. Taking a cue from nature, researchers often create 3D cell cultures using hydrogels that can closely mimic the extracellular matrix that most mammalian cells are surrounded by in vivo. However, aside from the collective physical 3D arrangement itself, the physiology of the culture depends highly on the microenvironment, which is defined by the 3D cell culture shape and the complex combination of biochemical, biophysical, and biomechanical stimuli. Microfluidic devices offer researchers the tantalizing opportunity to precisely define and influence this microenvironment. Furthermore, they additionally enable the integration of external functional components for active stimulation and monitoring of cultured cells. Pushing for ever-more-realistic culture conditions has, however, increased the complexity that is required of these microfluidic culture systems, making their fabrication more difficult. In this regard, 3D printing is becoming an increasingly popular solution, as it offers researchers not only the ability to fabricate highly complex structures but also to benefit from rapid prototyping and customization of existing designs. This review discusses common challenges that researchers currently face when integrating hydrogel-embedded cells into 3D-printed microfluidic cell culture devices and seeks to offer a comprehensive overview of recent advancements aimed at addressing these challenges.

- Castiaux AD, Spence DM, Martin RS. Review of 3D cell culture with analysis in microfluidic systems. Anal Method. 2019;11(33):4220-4232. doi: 10.1039/C9AY01328H
- Sośniak J, Opiela J. 3D cell culture technology - a new insight into in vitro research - a review. Ann Anim Sci. 2021;21(4):1257-1273. doi: 10.2478/aoas-2021-0039
- Rosser J, Olmos Calvo I, Schlager M, Purtscher M, Jenner F, Ertl P. Recent advances of biologically inspired 3D microfluidic hydrogel cell culture systems. Cell Biol Cell Metab. 2015;2(1):1-14. doi: 10.24966/cbcm-1943/100005
- Saydé T, Hamoui O El, Alies B, Gaudin K, Lespes G, Battu S. Biomaterials for three-dimensional cell culture: from applications in oncology to nanotechnology. Nanomaterials. 2021;11(2):1-29. doi: 10.3390/nano11020481
- Zommiti M, Connil N, Tahrioui A, et al. Organs-on-chips platforms are everywhere: a zoom on biomedical investigation. Bioengineering. 2022;9(11):646. doi: 10.3390/bioengineering9110646
- Song K, Li G, Zu X, Du Z, Liu L, Hu Z. The fabrication and application mechanism of microfluidic systems for high throughput biomedical screening: a review. Micromachines (Basel). 2020;11(3):297. doi: 10.3390/mi11030297
- Campbell SB, Wu Q, Yazbeck J, Liu C, Okhovatian S, Radisic M. Beyond polydimethylsiloxane: alternative materials for fabrication of organ-on-a-chip devices and microphysiological systems. ACS Biomater Sci Eng. 2021;7(7):2880-2899. doi: 10.1021/acsbiomaterials.0c00640
- Shinha K, Nihei W, Ono T, Nakazato R, Kimura H. A pharmacokinetic–pharmacodynamic model based on multi-organ-on-a-chip for drug–drug interaction studies. Biomicrofluidics. 2020;14(4):044108. doi: 10.1063/5.0011545
- Khan I, Prabhakar A, Delepine C, Tsang H, Pham V, Sur M. A low-cost 3D printed microfluidic bioreactor and imaging chamber for live-organoid imaging. Biomicrofluidics. 2021;15(2):024105. doi: 10.1063/5.0041027
- Akther F, Little P, Li Z, Nguyen NT, Ta HT. Hydrogels as artificial matrices for cell seeding in microfluidic devices. RSC Adv. 2020;10(71):43682-43703. doi: 10.1039/d0ra08566a
- Tenje M, Cantoni F, Porras Hernández AM, et al. A practical guide to microfabrication and patterning of hydrogels for biomimetic cell culture scaffolds. Organs-on-a-Chip. 2020;2:100003. doi: 10.1016/j.ooc.2020.100003
- Saraswathibhatla A, Indana D, Chaudhuri O. Cell– extracellular matrix mechanotransduction in 3D. Nat Rev Mol Cell Biol. 2023;24(7):495-516. doi: 10.1038/s41580-023-00583-1
- Yan Z, Kavanagh T, da Silva Harrabi R, et al. FRET sensor‐modified synthetic hydrogels for real‐time monitoring of cell‐derived matrix metalloproteinase activity using fluorescence lifetime imaging. Adv Funct Mater. 2024; 34(21):adfm.202309711. doi: 10.1002/adfm.202309711
- Young EWK, Beebe DJ. Fundamentals of microfluidic cell culture in controlled microenvironments. Chem Soc Rev. 2010;39(3):1036. doi: 10.1039/b909900j
- An S, Han SY, Cho S-W. Hydrogel-integrated microfluidic systems for advanced stem cell engineering. Biochip J. 2019;13(4):306-322. doi: 10.1007/s13206-019-3402-5
- Paggi CA, Venzac B, Karperien M, Leijten JCH, Le Gac S. Monolithic microfluidic platform for exerting gradients of compression on cell-laden hydrogels, and application to a model of the articular cartilage. Sens Actuators B Chem. 2020;315:127917. doi: 10.1016/j.snb.2020.127917
- Moldero IL, Chandra A, Cavo M, et al. Probing the pH microenvironment of mesenchymal stromal cell cultures on additive-manufactured scaffolds. Small. 2020;16(34):e2002258. doi: 10.1002/smll.202002258
- Leung CM, de Haan P, Ronaldson-Bouchard K, et al. A guide to the organ-on-a-chip. Nat Rev Methods Primers. 2022;2(1):33. doi: 10.1038/s43586-022-00118-6
- Cui L, Yao Y, Yim EKF. The effects of surface topography modification on hydrogel properties. APL Bioeng. 2021;5(3):031509. doi: 10.1063/5.004607620.
- Siller IG, Enders A, Gellermann P, et al. Characterization of a customized 3D-printed cell culture system using clear, translucent acrylate that enables optical online monitoring. Biomed Mater. 2020;15(5):055007. doi: 10.1088/1748-605X/ab8e97
- Khan S, Ullah A, Ullah K, Rehman N. Insight into hydrogels. Des Monomers Polym. 2016;19(5):456-478. doi: 10.1080/15685551.2016.1169380
- Mehta V, Rath SN. 3D printed microfluidic devices: a review focused on four fundamental manufacturing approaches and implications on the field of healthcare. Biodes Manuf. 2021;4(2):311-343. doi: 10.1007/s42242-020-00112-5
- Heuer C, Preuß J, Habib T, Enders A, Bahnemann J. 3D printing in biotechnology—an insight into miniaturized and microfluidic systems for applications from cell culture to bioanalytics. Eng Life Sci. 2022;22(12):744-759. doi: 10.1002/elsc.202100081
- Kotz F, Helmer D, Rapp BE. Emerging technologies and materials for high-resolution 3D printing of microfluidic chips. In: Advances in Biochemical Engineering/Biotechnology, Vol 179. Deutschland GmbH: Springer Science and Business Media; 2020:37-66. doi: 10.1007/10_2020_141
- Carvalho V, Gonçalves I, Lage T, et al. 3D printing techniques and their applications to organ-on-a-chip platforms: a systematic review. Sensors. 2021;21(9):3304. doi: 10.3390/S21093304
- Bunea A-I, del Castillo Iniesta N, Droumpali A, Wetzel AE, Engay E, Taboryski R. Micro 3D printing by two-photon polymerization: configurations and parameters for the nanoscribe system. Micro. 2021;1(2):164-180. doi: 10.3390/micro1020013
- Luitz M, Kırpat Konak BM, Sherbaz A, et al. Fabrication of embedded microfluidic chips with single micron resolution using two‐photon lithography. Adv Mater Technol. 2023;8(22):2300667. doi: 10.1002/admt.202300667
- Li F, Macdonald NP, Guijt RM, Breadmore MC. Increasing the functionalities of 3D printed microchemical devices by single material, multimaterial, and print-pause-print 3D printing. Lab Chip. 2019;19(1):35-49. doi: 10.1039/C8LC00826D
- Song Q, Chen Y, Hou P, et al. Fabrication of multi-material pneumatic actuators and microactuators using stereolithography. Micromachines (Basel). 2023; 14(2):244. doi: 10.3390/mi14020244
- Winkler S, Menke J, Meyer KV, Kortmann C, Bahnemann J. Automation of cell culture assays using a 3D-printed servomotor-controlled microfluidic valve system. Lab Chip. 2022;22(23):4656-4665. doi: 10.1039/d2lc00629d
- van den Driesche S, Lucklum F, Bunge F, Vellekoop M. 3D printing solutions for microfluidic chip-to-world connections. Micromachines (Basel). 2018;9(2):71. doi: 10.3390/mi9020071
- Meyer KV, Winkler S, Lienig P, Dräger G, Bahnemann J. 3D-printed microfluidic perfusion system for parallel monitoring of hydrogel-embedded cell cultures. Cells. 2023;12(14):1816. doi: 10.3390/cells12141816
- Priyadarshini BM, Dikshit V, Zhang Y. 3D-printed bioreactors for in vitro modeling and analysis. Int J Bioprint. 2020;6(4):267. doi: 10.18063/ijb.v6i4.267
- Terrell JA, Jones CG, Kabandana GKM, Chen C. From cells-on-a-chip to organs-on-a-chip: scaffolding materials for 3D cell culture in microfluidics. J Mater Chem B. 2020;8(31):6667-6685. doi: 10.1039/D0TB00718H
- Maji S, Lee H. Engineering hydrogels for the development of three-dimensional in vitro models. Int J Mol Sci. 2022;23(5):2662. doi: 10.3390/ijms23052662
- Ma Y, Han T, Yang Q, et al. Viscoelastic cell microenvironment: hydrogel‐based strategy for recapitulating dynamic ECM mechanics. Adv Funct Mater. 2021;31(24):2100848. doi: 10.1002/adfm.202100848
- El-Husseiny HM, Mady EA, Hamabe L, et al. Smart/stimuli-responsive hydrogels: cutting-edge platforms for tissue engineering and other biomedical applications. Mater Today Bio. 2022;13:100186. doi: 10.1016/j.mtbio.2021.100186
- Kim YT, Bohjanen S, Bhattacharjee N, Folch A. Partitioning of hydrogels in 3D-printed microchannels. Lab Chip. 2019; 19(18):3086-3093. doi: 10.1039/C9LC00535H
- Fuchs S, Rieger V, Tjell A, et al. Optical glucose sensor for microfluidic cell culture systems. Biosens Bioelectron. 2023;237:115491. doi: 10.1016/j.bios.2023.11549140.
- Nie J, Fu J, He Y. Hydrogels: the next generation body materials for microfluidic chips? Small. 2020;16(46): 1-26. doi: 10.1002/smll.202003797
- Haefner S, Koerbitz R, Frank P, Elstner M, Richter A. High integration of microfluidic circuits based on hydrogel valves for MEMS control. Adv Mater Technol. 2018;3(1):1-10. doi: 10.1002/admt.201700108
- Hsu M-C, Mansouri M, Ahamed NNN, et al. A miniaturized 3D printed pressure regulator (μPR) for microfluidic cell culture applications. Sci Rep. 2022;12(1):10769. doi: 10.1038/s41598-022-15087-9
- Yazdi SAFF, Corigliano A, Ardito R. 3-D design and simulation of a piezoelectric micropump. Micromachines (Basel). 2019;10(4):259. doi: 10.3390/mi10040259
- Ameri AR, Imanparast A, Passandideh-Fard M, Mousavi Shaegh SA. A whole-thermoplastic microfluidic chip with integrated on-chip micropump, bioreactor and oxygenator for cell culture applications. Anal Chim Acta. 2022;1221:340093. doi: 10.1016/j.aca.2022.340093
- Vollertsen AR, de Boer D, Dekker S, et al. Modular operation of microfluidic chips for highly parallelized cell culture and liquid dosing via a fluidic circuit board. Microsyst Nanoeng. 2020;6(1):107. doi: 10.1038/s41378-020-00216-z
- Beckwith AL, Borenstein JT, Velasquez-Garcia LF. Monolithic, 3D-printed microfluidic platform for recapitulation of dynamic tumor microenvironments. J Microelectromech Syst. 2018;27(6):1009-1022. doi: 10.1109/JMEMS.2018.2869327
- Huang C, Wippold JA, Stratis-Cullum D, Han A. Eliminating air bubble in microfluidic systems utilizing integrated in-line sloped microstructures. Biomed Microdevices. 2020; 22(4):76. doi: 10.1007/s10544-020-00529-w
- Xu J, Vaillant R, Attinger D. Use of a porous membrane for gas bubble removal in microfluidic channels: physical mechanisms and design criteria. Microfluid Nanofluidics. 2010;9(4–5):765-772. doi: 10.1007/s10404-010-0592-5
- Fritschen A, Bell AK, Königstein I, Stühn L, Stark RW, Blaeser A. Investigation and comparison of resin materials in transparent DLP-printing for application in cell culture and organs-on-a-chip. Biomater Sci. 2022;10(8): 1981-1994. doi: 10.1039/D1BM01794B
- Winkler S, Meyer KV, Heuer C, Kortmann C, Dehne M, Bahnemann J. In vitro biocompatibility evaluation of a heat-resistant 3D printing material for use in customized cell culture devices. Eng Life Sci. 2022;22(11):699-708. doi: 10.1002/elsc.202100104
- Ferràs-Tarragó J, Sabalza-Baztán O, Sahuquillo-Arce JM, et al. Autoclave sterilization of an in-house 3D-printed polylactic acid piece: biological safety and heat-induced deformation. Eur J Trauma Emerg Surg. 2022;48(5):3901-3910. doi: 10.1007/s00068-021-01672-6
- Valls-Esteve A, Lustig-Gainza P, Adell-Gomez N, Tejo-Otero A, Englí-Rueda M, Julian-Alvarez E. A state-of-the-art guide about the effects of sterilization processes on 3D-printed materials for surgical planning and medical applications: a comparative study. Int J Bioprint. 2023;9(5):756. doi: 10.18063/ijb.756
- Told R, Ujfalusi Z, Pentek A, et al. A state-of-the-art guide to the sterilization of thermoplastic polymers and resin materials used in the additive manufacturing of medical devices. Mater Des. 2022;223:111119. doi: 10.1016/j.matdes.2022.111119
- Guttridge C, Shannon A, O’Sullivan A, O’Sullivan KJ, O’Sullivan LW. Biocompatible 3D printing resins for medical applications: a review of marketed intended use, biocompatibility certification, and post-processing guidance. Ann 3D Printed Med. 2021;5:100044. doi: 10.1016/j.stlm.2021.100044
- Grab M, Jaud S, Thierfelder N, et al. Flexible 3D-printable materials for application in medical research: a comprehensive comparison of commercially available materials. 3D Print Addit Manuf. 2024. doi: 10.1089/3dp.2023.0179
- Williams DF. There is no such thing as a biocompatible material. Biomaterials. 2014;35(38):10009-10014. doi: 10.1016/j.biomaterials.2014.08.035
- Williams DF. The language of biomaterials-based technologies. Regen Eng Transl Med. 2019;5(1):53-60. doi: 10.1007/s40883-018-0088-5
- Bernard M, Jubeli E, Pungente MD, Yagoubi N. Biocompatibility of polymer-based biomaterials and medical devices – regulations, in vitro screening and risk-management. Biomater Sci. 2018;6(8):2025-2053. doi: 10.1039/C8BM00518D
- Trebuňová M, Petroušková P, Balogová AF, et al. Evaluation of biocompatibility of PLA/PHB/TPS polymer scaffolds with different additives of ATBC and OLA plasticizers. J Funct Biomater. 2023;14(8):412. doi: 10.3390/jfb14080412
- Chakraborty R, Anoop AG, Thakur A, Mohanta GC, Kumar P. Strategies to modify the surface and bulk properties of 3D-printed solid scaffolds for tissue engineering applications. ACS Omega. 2023;8(6):5139-5156. doi: 10.1021/acsomega.2c05984
- Diniță A, Neacșa A, Portoacă AI, Tănase M, Ilinca CN, Ramadan IN. Additive manufacturing post-processing treatments, a review with emphasis on mechanical characteristics. Materials. 2023;16(13):4610. doi: 10.3390/ma16134610
- Karakurt I, Lin L. 3D printing technologies: techniques, materials, and post-processing. Curr Opin Chem Eng. 2020;28:134-143. doi: 10.1016/j.coche.2020.04.001
- Männel MJ, Weigel N, Hauck N, Heida T, Thiele J. Combining hydrophilic and hydrophobic materials in 3D printing for fabricating microfluidic devices with spatial wettability. Adv Mater Technol. 2021;6(9):2100094. doi: 10.1002/admt.202100094
- Sharaf A, Roos B, Timmerman R, Kremers GJ, Bajramovic JJ, Accardo A. Two-photon polymerization of 2.5D and 3D microstructures fostering a Ramified resting phenotype in primary microglia. Front Bioeng Biotechnol. 2022;10:926642. doi: 10.3389/fbioe.2022.926642
- Hart C, Didier CM, Sommerhage F, Rajaraman S. Biocompatibility of blank, post-processed and coated 3D printed resin structures with electrogenic cells. Biosensors (Basel). 2020;10(11):152. doi: 10.3390/BIOS10110152
- Jeršovaitė J, Šarachovaitė U, Matulaitienė I, Niaura G, Baltriukienė D, Malinauskas M. Biocompatibility enhancement via post-processing of microporous scaffolds made by optical 3D printer. Front Bioeng Biotechnol. 2023;11:1-14. doi: 10.3389/fbioe.2023.1167753
- Del Rosario M, Heil HS, Mendes A, Saggiomo V, Henriques R. The field guide to 3D printing in optical microscopy for life sciences. Adv Biol. 2022;6(4):e2100994. doi: 10.1002/adbi.202100994
- Mora-Boza A, Mulero-Russe A, Caprio ND, Burdick JA, Singh A, García AJ. Facile photopatterning of perfusable microchannels in synthetic hydrogels to recreate microphysiological environments. Adv Mater. 2023;35(52):2306765. doi: 10.1002/adma.202306765
- Virumbrales-Muñoz M, Ayuso JM, Lacueva A, et al. Enabling cell recovery from 3D cell culture microfluidic devices for tumour microenvironment biomarker profiling. Sci Rep. 2019;9(1):6199. doi: 10.1038/s41598-019-42529-8
- Shanti A, Samara B, Abdullah A, et al. Multi-compartment 3D-cultured organ-on-a-chip: towards a biomimetic lymph node for drug development. Pharmaceutics. 2020; 12(5):464. doi: 10.3390/pharmaceutics12050464
- Schneider S, Gruner D, Richter A, Loskill P. Membrane integration into PDMS-free microfluidic platforms for organ-on-chip and analytical chemistry applications. Lab Chip. 2021;21(10):1866-1885. doi: 10.1039/d1lc00188d
- Warr CA, Crawford NG, Nordin GP, Pitt WG. Surface modification of 3D printed microfluidic devices for controlled wetting in two-phase flow. Micromachines (Basel). 2023;14(1):6. doi: 10.3390/mi14010006
- Stöbener DD, Weinhart M. “Fuzzy hair” promotes cell sheet detachment from thermoresponsive brushes already above their volume phase transition temperature. Biomater Adv. 2022;141:213101. doi: 10.1016/j.bioadv.2022.213101
- Ying G, Jiang N, Parra‐Cantu C, et al. Bioprinted injectable hierarchically porous gelatin methacryloyl hydrogel constructs with shape‐memory properties. Adv Funct Mater. 2020;30(46):2003740. doi: 10.1002/adfm.202003740
- Li S, Li H, Shang X, He J, Hu Y. Recent advances in 3D printing sacrificial templates for fabricating engineered vasculature. MedComm - Biomater Appl. 2023;2(3):e46. doi: 10.1002/mba2.46
- Advincula RC, Dizon JRC, Caldona EB, et al. On the progress of 3D-printed hydrogels for tissue engineering. MRS Commun. 2021;11(5):539-553. doi: 10.1557/s43579-021-00069-1
- Miksch CE, Skillin NP, Kirkpatrick BE, et al. 4D printing of extrudable and degradable poly(ethylene glycol) microgel scaffolds for multidimensional cell culture. Small. 2022;18(36):2200951. doi: 10.1002/smll.202200951
- Tabatabaei Rezaei N, Kumar H, Liu H, Lee SS, Park SS, Kim K. Recent advances in organ‐on‐chips integrated with bioprinting technologies for drug screening. Adv Healthc Mater. 2023;12(20):e202203172. doi: 10.1002/adhm.202203172
- Raees S, Ullah F, Javed F, et al. Classification, processing, and applications of bioink and 3D bioprinting: a detailed review. Int J Biol Macromol. 2023;232:123476. doi: 10.1016/j.ijbiomac.2023.123476
- Mobaraki M, Ghaffari M, Yazdanpanah A, Luo Y, Mills DK. Bioinks and bioprinting: a focused review. Bioprinting. 2020;18:e00080. doi: 10.1016/j.bprint.2020.e00080
- Rothbauer M, Eilenberger C, Spitz S, et al. Recent advances in additive manufacturing and 3D bioprinting for organs-on-a-chip and microphysiological systems. Front Bioeng Biotechnol. 2022;10:837087. doi: 10.3389/fbioe.2022.837087
- Barros da Silva P, Coelho M, Bidarra SJ, Neves SC, Barrias CC. Reshaping in vitro models of breast tissue: integration of stromal and parenchymal compartments in 3D printed hydrogels. Front Bioeng Biotechnol. 2020;8:494. doi: 10.3389/fbioe.2020.00494
- Yang J, Bai R, Chen B, Suo Z. Hydrogel adhesion: a supramolecular synergy of chemistry, topology, and mechanics. Adv Funct Mater. 2020;30(2):1901693. doi: 10.1002/adfm.201901693
- Qiao Y, Gong J, Jin Z, Tu Y, Yang X. An optimized method of culturing neurons based on polyacrylamide gel. Biophys Rep. 2024;10(1):41. doi: 10.52601/bpr.2023.230033
- Sun W, Liu T, Zhang X, et al. Aquatic diatoms‐inspired universal adhesive coacervates triggered by water. Adv Healthc Mater. 2023;12(24):e2300669. doi: 10.1002/adhm.202300669
- Gao Y, Chen J, Han X, et al. A universal strategy for tough adhesion of wet soft material. Adv Funct Mater. 2020;30(36):2003207. doi: 10.1002/adfm.202003207
- Hernández-Castro JA, Li K, Daoud J, Juncker D, Veres T. Two-level submicron high porosity membranes (2LHPM) for the capture and release of white blood cells (WBCs). Lab Chip. 2019;19(4):589-597. doi: 10.1039/C8LC01256C
- Fuchs S, Johansson S, Tjell AØ, Werr G, Mayr T, Tenje M. In-line analysis of organ-on-chip systems with sensors: integration, fabrication, challenges, and potential. ACS Biomater Sci Eng. 2021;7(7):2926-2948. doi: 10.1021/acsbiomaterials.0c01110
- Ma HL, Urbaczek AC, Zeferino Ribeiro de Souza F, et al. Rapid fabrication of microfluidic devices for biological mimicking: a survey of materials and biocompatibility. Micromachines (Basel). 2021;12(3):346. doi: 10.3390/mi12030346
- Yao Y, Li L, Jiang J, Zhang Y, Chen G, Fan Y. Reversible bonding for microfluidic devices with UV release tape. Microfluid Nanofluidics. 2022;26(3):23. doi: 10.1007/s10404-022-02532-4
- Kim YT, Ahmadianyazdi A, Folch A. A ‘print–pause–print’ protocol for 3D printing microfluidics using multimaterial stereolithography. Nat Protoc. 2023;18(4):1243-1259. doi: 10.1038/s41596-022-00792-6
- Fernandes A, Hosseini V, Vogel V, Lovchik RD. Engineering solutions for biological studies of flow-exposed endothelial cells on orbital shakers. PLoS One. 2022; 17(1 January):e262044. doi: 10.1371/journal.pone.0262044
- Tamargo IA, Baek KI, Kim Y, Park C, Jo H. Flow-induced reprogramming of endothelial cells in atherosclerosis. Nat Rev Cardiol. 2023;20(11):738-753. doi: 10.1038/s41569-023-00883-1
- Chaudhuri O, Cooper-White J, Janmey PA, Mooney DJ, Shenoy VB. Effects of extracellular matrix viscoelasticity on cellular behaviour. Nature. 2020;584(7822):535-546. doi: 10.1038/s41586-020-2612-2
- Lavrentieva A, Fleischhammer T, Enders A, Pirmahboub H, Bahnemann J, Pepelanova I. Fabrication of stiffness gradients of GelMA hydrogels using a 3D printed micromixer. Macromol Biosci. 2020;20(7):2000107. doi: 10.1002/mabi.202000107
- Bhattacharjee I, Sudhiram S, Nithya M, Kumar B, Sarangi BR. Diffusion mediated rigidity-gradient hydrogel substrate for cellular mechanosensing. Eur Phys J Spec Top. 2024;233:3123-3131. doi: 10.1140/epjs/s11734-024-01125-2
- Kaliaraj G, Shanmugam D, Dasan A, Mosas K. Hydrogels—a promising materials for 3d printing technology. Gels. 2023;9(3):260. doi: 10.3390/gels9030260 doi: 10.1021/acsnano.0c05197
- Kasiński A, Zielińska-Pisklak M, Oledzka E, Sobczak M. Smart hydrogels – synthetic stimuli-responsive antitumor drug release systems. Int J Nanomedicine. 2020;15:4541-4572. doi: 10.2147/IJN.S248987
- Lei L, Ma B, Xu C, Liu H. Emerging tumor-on-chips with electrochemical biosensors. TrAC Trends Anal Chem. 2022;153:116640. doi: 10.1016/j.trac.2022.116640
- Lyu Z, Park J, Kim KM, et al. A neurovascular-unit-on-a-chip for the evaluation of the restorative potential of stem cell therapies for ischaemic stroke. Nat Biomed Eng. 2021;5(8):847-863. doi: 10.1038/s41551-021-00744-7
- Melo-Fonseca F, Carvalho O, Gasik M, Miranda G, Silva FS. Mechanical stimulation devices for mechanobiology studies: a market, literature, and patents review. Biodes Manuf. 2023;6(3):340-371. doi: 10.1007/s42242-023-00232-8
- Shiwarski DJ, Tashman JW, Eaton AF, Apodaca G, Feinberg AW. 3D printed biaxial stretcher compatible with live fluorescence microscopy. HardwareX. 2020;7:e00095. doi: 10.1016/j.ohx.2020.e00095
- Hinnen H, Viglione M, Munro TR, Woolley AT, Nordin GP. 3D-printed microfluidic one-way valves and pumps. Micromachines (Basel). 2023;14(7):1286. doi: 10.3390/mi14071286
- Yang C, Xiao Y, Hu L, et al. Stimuli‐triggered multishape, multimode, and multistep deformations designed by microfluidic 3D droplet printing. Small. 2023;19(11):e2207073. doi: 10.1002/smll.202207073
- Arik YB, De Sa Vivas A, Laarveld D, et al. Collagen i based enzymatically degradable membranes for organ-on-a-chip barrier models. ACS Biomater Sci Eng. 2021;7(7):2998-3005. doi: 10.1021/acsbiomaterials.0c00297
- Buchanan BC, Yoon J-Y. Microscopic imaging methods for organ-on-a-chip platforms. Micromachines (Basel). 2022;13(2):328. doi: 10.3390/mi13020328
- Hamed H, Eldiasty M, Seyedi-Sahebari S-M, Abou-Ziki JD. Applications, materials, and fabrication of micro glass parts and devices: An overview. Mater Today. 2023;66: 194-220. doi: 10.1016/j.mattod.2023.03.005
- Kotz F, Helmer D, Rapp BE. In: Heinrich A, ed. 3D Printing of Transparent Glasses; 2021:169-184. doi: 10.1007/978-3-030-58960-8_4
- Zavadakova A, Vistejnova L, Belinova T, Tichanek F, Bilikova D, Mouton PR. Novel stereological method for estimation of cell counts in 3D collagen scaffolds. Sci Rep. 2023;13(1):7959. doi: 10.1038/s41598-023-35162-z
- Eschweiler D, Smith RS, Stegmaier J. Robust 3D cell segmentation: extending the view of cellpose. In: Proceedings - International Conference on Image Processing, ICIP. IEEE Computer Society; 2022:191-195. doi: 10.1109/ICIP46576.2022.9897942
- Ko J, Park D, Lee J, et al. Microfluidic high-throughput 3D cell culture. Nat Rev Bioeng. 2024;2(6):453-469. doi: 10.1038/s44222-024-00163-8
- Gonçalves IM, Rodrigues RO, Moita AS, et al. Recent trends of biomaterials and biosensors for organ-on-chip platforms. Bioprinting. 2022;26:e00202. doi: 10.1016/j.bprint.2022.e00202
- Buttkewitz MA, Heuer C, Bahnemann J. Sensor integration into microfluidic systems: trends and challenges. Curr Opin Biotechnol. 2023;83(1):102978. doi: 10.1016/j.copbio.2023.102978
- Fedi A, Vitale C, Giannoni P, Caluori G, Marrella A. Biosensors to monitor cell activity in 3d hydrogel-based tissue models. Sensors. 2022;22(4):1517. doi: 10.3390/s22041517
- Lou C, Yang H, Hou Y, et al. Microfluidic platforms for real-time in situ monitoring of biomarkers for cellular processes. Adv Mater. 2024;36(6):e2307051. doi: 10.1002/adma.202307051
- Cortesi M, Giordano E. Non-destructive monitoring of 3D cell cultures: new technologies and applications. PeerJ. 2022;10:e13338. doi: 10.7717/peerj.13338
- Li Z, Zhang R, Xu F, Yang J, Zhou L, Mao H. A cell state monitoring system with integrated in situ imaging and pH detection. Sensors (Basel). 2023;23(23):9340. doi: 10.3390/s23239340
- McLennan HJ, Blanch AJ, Wallace SJ, et al. Nano-liter perfusion microfluidic device made entirely by two-photon polymerization for dynamic cell culture with easy cell recovery. Sci Rep. 2023;13(1):562. doi: 10.1038/s41598-023-27660-x
- Simão D, Gomes CM, Alves PM, Brito C. Capturing the third dimension in drug discovery: spatially-resolved tools for interrogation of complex 3D cell models. Biotechnol Adv. 2022;55:107883. doi: 10.1016/j.biotechadv.2021.107883
- Mahboubian AR, Vllasaliu D, Dorkoosh FA, Stolnik S. Temperature-responsive methylcellulose-hyaluronic hydrogel as a 3D cell culture matrix. Biomacromolecules. 2020;21(12):4737-4746. doi: 10.1021/acs.biomac.0c00906
- Mahboubian AR, Vllasaliu D, Dorkoosh FA, Stolnik S. Temperature-responsive methylcellulose-hyaluronic hydrogel as a 3D cell culture matrix. Biomacromolecules. 2020;21(12):4737-4746. doi: 10.1021/acs.biomac.0c00906