Design, fabrication, and biocompatibility of 3D-printed poly(LLA-ran-PDO-ran-GA)/poly (D-lactide) composite scaffolds for bone tissue engineering
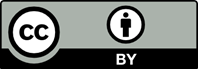
The long-term nonunion of bone defects remains a significant challenge in the field of orthopedics. Poly(L-lactic acid) (PLLA), widely used in bone tissue engineering, offers hope for addressing this issue. In our previous study, we aimed to enhance the poor toughness and slow degradation of PLLA by introducing flexible p-dioxanone (PDO) and highly reactive glycolic acid units into the molecular chain of PLLA to prepare PLLA-ran-PDO-ran-GA (PLPG) scaffolds, effectively mitigating the limitations of PLLA. However, the crystallization capacity of PLPG copolymers was weakened, resulting in insufficient mechanical properties. Therefore, in this study, poly(D-lactic acid) (PDLA) was introduced into PLPG via solution blending to enhance its crystallization properties through the in situ generation of stereocomplex poly(lactic acid) (SC-PLA). Subsequently, PLPG/PDLA scaffolds were prepared using 3D printing technology. The results demonstrated that PLPG/PDLA composites exhibited good machinability, while the scaffolds exhibited satisfactory mechanical and degradation properties. Additionally, cell experiments indicated that PLPG/PDLA scaffolds are biocompatible, supporting cell growth and proliferation on their surfaces. We believe that PLPG/PDLA scaffolds have significant potential for application in bone tissue engineering, effectively addressing the issue of long-term non-healing bone defects.

- Chen Y, Huang J, Liu J, et al. Tuning filament composition and microstructure of 3D-printed bioceramic scaffolds facilitate bone defect regeneration and repair. Regen Biomater. 2021;8:rbab007. doi: 10.1093/rb/rbab007
- Wei S, Ma J, Xu L, et al. Biodegradable materials for bone defect repair. Military Med Res. 2020;7:1-25. doi: 10.1186/s40779-020-00280-6
- Xu L, Ma F, Leung FK, et al. Chitosan-strontium chondroitin sulfate scaffolds for reconstruction of bone defects in aged rats. Carbohyd Polym. 2021;273:118532. doi: 10.1016/j.carbpol.2021.118532
- Cendrero AM, Martínez FF, Requejo WGS, et al. Open-source library of tissue engineering scaffolds. Mater Design. 2022;223:111154. doi: 10.1016/j.matdes.2022.111154
- Wang C, Huang W, Zhou Y, et al. 3D printing of bone tissue engineering scaffolds. Bioact Mater. 2020;5:82-91. doi: 10.1016/j.bioactmat.2020.01.004
- Asghari F, Faradonbeh DR, Malekshahi ZV, et al. Hybrid PCL/chitosan-PEO nanofibrous scaffolds incorporated with A. euchroma extract for skin tissue engineering application. Carbohyd Polym. 2022;278:118926. doi: 10.1016/j.carbpol.2021.118926
- Kuang T, Chen F, Chang L, et al. Facile preparation of open-cellular porous poly (l-lactic acid) scaffold by supercritical carbon dioxide foaming for potential tissue engineering applications. Chem Eng J. 2017;307:1017-1025. doi: 10.1016/j.cej.2016.09.023
- Bertsch C, Maréchal H, Gribova V, et al. Biomimetic bilayered scaffolds for tissue engineering: from current design strategies to medical applications. Adv Healthc Mater. 2023;12:2203115. doi: 10.1002/adhm.202203115
- Nigmatullin R, Taylor CS, Basnett P, et al. Medium chain length polyhydroxyalkanoates as potential matrix materials for peripheral nerve regeneration. Regen Biomater. 2023;10:rbad063. doi: 10.1093/rb/rbad063
- Duan R, Wang Y, Su D, et al. The effect of blending poly(l-lactic acid) on in vivo performance of 3D-printed poly(l-lactide-co-caprolactone)/PLLA scaffolds. Biomater Adv. 2022;138:212948. doi: 10.1016/j.bioadv.2022.212948
- Zheng S, Li W, Chen Y, et al. Synergistic effect of stereo-complexation and interfacial compatibility in ammonium polyphosphate grafted polylactic acid fibers for simultaneously improved toughness and flame retardancy. Int J Biol Macromol. 2024;261(Pt 2):129943. doi: 10.1016/j.ijbiomac.2024.129943
- Yang Y, Zan J, Yang W, et al. Metal organic frameworks as a compatible reinforcement in a biopolymer bone scaffold. Mater Chem Front. 2020;4:973-984. doi: 10.1039/C9QM00772E
- Fan T, Qin J, Li J, et al. Fabrication and evaluation of 3D printed poly(l-lactide) copolymer scaffolds for bone tissue engineering. Int J Biol Macromol. 2023;245:125525. doi: 10.1016/j.ijbiomac.2023.125525
- Liu H, Bai D, Bai H, et al. Constructing stereocomplex structures at the interface for remarkably accelerating matrix crystallization and enhancing the mechanical properties of poly(L-lactide)/multi-walled carbon nanotube nanocomposites. J Mater Chem A. 2015;3:13835-13847. doi: 10.1039/c5ta02017d
- Cheng Y, Jiao Z, Li M, et al. A new class of nucleating agents for poly(L-lactic acid): environmentally-friendly metal salts with biomass-derived ligands and advanced nucleation ability. Int J Biol Macromol. 2023;225:1599-1606. doi: 10.1016/j.ijbiomac.2022.11.216
- Yu B, Meng L, Fu S, et al. Morphology and internal structure control over PLA microspheres by compounding PLLA and PDLA and effects on drug release behavior. Colloid Surface B. 2018;172:105-112. doi: 10.1016/j.colsurfb.2018.08.037
- Zhang Y, Wang Y, Wang B, et al. Exclusive formation of poly(lactide) stereocomplexes with enhanced melt stability via regenerated cellulose assisted Pickering emulsion approach. Compos Commun. 2022;32:101138. doi: 10.1016/j.coco.2022.101138
- Zhou W, Chen X, Yang K, et al. Achieving morphological evolution and interfacial enhancement in fully degradable and supertough polylactide/polyurethane elastomer blends by interfacial stereocomplexation. Appl Surf Sci. 2022;572:151393. doi: 10.1016/j.apsusc.2021.151393
- Zhang H, Bai H, Deng S, et al. Achieving all-polylactide fibers with significantly enhanced heat resistance and tensile strength via in situ formation of nanofibrilized stereocomplex polylactide. Polymer. 2019;166:13-20. doi: 10.1016/j.polymer.2019.01.040
- Jalali A, Romero-Diez S, Nofar M, et al. Entirely environment-friendly polylactide composites with outstanding heat resistance and superior mechanical performance fabricated by spunbond technology: exploring the role of nanofibrillated stereocomplex polylactide crystals. Int J Biol Macromol. 2021;193:2210-2220. doi: 10.1016/j.ijbiomac.2021.11.052
- Li J, Ye W, Fan Z, et al. A novel stereocomplex poly (lactic acid) with shish-kebab crystals and bionic surface structures as bioimplant materials for tissue engineering applications. ACS Appl Mater Interfaces. 2021;13:5469-5477. doi: 10.1021/acsami.0c17465
- Feng L, Bian X, Li G, et al. Thermal properties and structural evolution of poly(l-lactide)/poly(d-lactide) blends. Macromolecules. 2021;54:10163-10176. doi: 10.1021/acs.macromol.1c01866
- Körber S, Moser K, Diemert J. Development of high temperature resistant stereocomplex PLA for injection moulding. Polymers. 2022;14:384. doi: 10.3390/polym14030384
- Yan Q, Dong H, Su J, et al. A review of 3D printing technology for medical applications. Engineering. 2018;4:729-742. doi: 10.1016/j.eng.2018.07.021
- Liu X, Zhang J, Cheng X, et al. Integrated printed BDNF-stimulated HUCMSCs-derived exosomes/collagen/chitosan biological scaffolds with 3D printing technology promoted the remodelling of neural networks after traumatic brain injury. Regen Biomater. 2023;10:rbac085. doi: 10.1093/rb/rbac085
- Jiang S, Wang M, He J. A review of biomimetic scaffolds for bone regeneration: toward a cell‐free strategy. Bioeng Transl Med. 2021;6:e10206. doi: 10.1002/btm2.10206
- Jia W, Li H, Wang Z, et al. 3D composite lithium metal with multilevel micro-nano structure combined with surface modification for stable lithium metal anodes. Appl Surf Sci. 2021;570:151159.doi: 10.1016/j.apsusc.2021.151159
- Feng C, Ma B, Xu M, et al. Three-dimensional printing of scaffolds with synergistic effects of micro–nano surfaces and hollow channels for bone regeneration. ACS Biomater Sci Eng. 2020;7:872-880. doi: 10.1021/acsbiomaterials.9b01824
- Fan T, Qin J, Dong F, et al. Effects on the crystallization behavior and biocompatibility of poly(LLA-ran-PDO-ran- GA) with poly(d-lactide) as nucleating agents. RSC Adv. 2022;12:10711-10724. doi: 10.1039/d2ra00525e
- Yu H, Chen X, Cai J, et al. Novel porous three-dimensional nanofibrous scaffolds for accelerating wound healing. Chem Eng J. 2019;369:253-262. doi: 10.1016/j.cej.2019.03.091
- Peng MW, Yu XL, Guan Y, et al. Underlying promotion mechanism of high concentration of silver nanoparticles on anammox process. ACS Nano. 2019;13:14500-14510. doi: 10.1021/acsnano.9b08263
- Zhang Y, Ge T, Li Y, et al. Anti-fouling and anti-biofilm performance of self-polishing waterborne polyurethane with Gemini quaternary ammonium salts. Polymers. 2023;15:317. doi: 10.3390/polym15020317
- Zhao Z, Yang L, Hu Y, et al. Enzymatic degradation of block copolymers obtained by sequential ring opening polymerization of l-lactide and ε-caprolactone. Polym Degrad Stabil. 2007;92:1769-1777. doi: 10.1016/j.polymdegradstab.2007.07.012
- Chen Z, Ding D, Yu T, et al. Enzymatic degradation behaviors and kinetics of bio-degradable jute/poly (lactic acid)(PLA) composites. Compos Commun. 2022;33:101227. doi: 10.1016/j.coco.2022.101227
- Huang Q, Hiyama M, Kabe T, et al. Enzymatic self-biodegradation of poly(l-lactic acid) films by embedded heat-treated and immobilized proteinase K. Biomacromolecules. 2020;21:3301-3307. doi: 10.1021/acs.biomac.0c00759
- Arbeiter D, Lebahn K, Reske T, et al. Comparison of accelerated and enzyme-associated real-time degradation of HMW PLLA and HMW P3HB films. Polym Test. 2022;107:107471. doi: 10.1016/j.polymertesting.2021.107471