Designing methacrylic anhydride-based hydrogels for 3D bioprinting
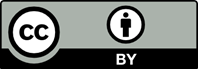
Methacrylic anhydride (MA)-based hydrogels, derived by introducing methacryloyl groups to various polymer side chains, are promising bioinks for three-dimensional (3D) printing in medical applications. These hydrogels combine the inherent biocompatibility and therapeutic benefits of their parent polymers with the unique photocrosslinking properties conferred by the methacryloyl groups, allowing precise control over their mechanical properties through light-curing parameters. Using 3D biological compression, these hydrogels serve as bioinks for producing scaffolds with optimal porosity, facilitating cell adhesion, proliferation, and differentiation. This technology enables the precise spatial distribution of bioactive substances, offering targeted therapeutic treatment and controlled release. This review delved into recent advancements in bioprinting technologies, outlined the preparation of MA-based bioinks, and summarized factors influencing the resulting biological and mechanical properties of bioscaffolds. Additionally, the properties and applications of methylpropionic anhydride-based hydrogels in various medical fields were discussed, addressing current limitations and future challenges in integrating these hydrogels with 3D bioprinting for clinical applications.

- Ravi M, Paramesh V, Kaviya SR, Anuradha E, Solomon FD. 3D cell culture systems: advantages and applications. J Cell Physiol. 2015;230:16-26. doi: 10.1002/jcp.24683
- Shankar V, van Blitterswijk C, Vrij E, Giselbrecht S. Automated, high-throughput phenotypic screening and analysis platform to study pre- and post-implantation morphogenesis in stem cell-derived embryo-like structures. Adv Sci (Weinh). 2023;11(4):2304987. doi: 10.1002/advs.202304987
- Jensen C, Teng Y. Is it time to start transitioning from 2D to 3D cell culture?, Front Mol Biosci. 2020;7:33. doi: 10.3389/fmolb.2020.00033
- Groll J, Burdick JA, Cho DW, et al. A definition of bioinks and their distinction from biomaterial inks. Biofabrication. 2018;11:013001. doi: 10.1088/1758-5090/aaec52
- Raja IS, Kang MS, Hong SW, et al. State-of-the-art techniques for promoting tissue regeneration: combination of three-dimensional bioprinting and carbon nanomaterials. Int J Bioprint. 2023;9:635. doi: 10.18063/ijb.v9i1.635
- Mandrycky C, Wang Z, Kim K, Kim DH. 3D bioprinting for engineering complex tissues. Biotechnol Adv. 2016;34:422-434. doi: 10.1016/j.biotechadv.2015.12.011
- Peng X, Wu S, Sun X, et al. 4D printing of freestanding liquid crystal elastomers via hybrid additive manufacturing. Adv Mater. 2022;34:e2204890. doi: 10.1002/adma.202204890
- Mainik P, Spiegel CA, Blasco E. Recent advances in multi-photon 3D laser printing: active materials and applications. Adv Mater. 2024;36(11):e2310100. doi: 10.1002/adma.202310100
- Tuan RS, Boland G, Tuli R. Adult mesenchymal stem cells and cell-based tissue engineering. Arthritis Res Ther. 2003;5:32-45. doi: 10.1186/ar614
- Klebe RJ. Cytoscribing: a method for micropositioning cells and the construction of two- and three-dimensional synthetic tissues. Exp Cell Res. 1988;179:362-373. doi: 10.1016/0014-4827(88)90275-3
- Li X, Liu B, Pei B, et al. Inkjet bioprinting of biomaterials. Chem Rev. 2020;120:10793-10833. doi: 10.1021/acs.chemrev.0c00008
- Miri AK, Mirzaee I, Hassan S, et al. Effective bioprinting resolution in tissue model fabrication. Lab Chip. 2019;19: 2019-2037. doi: 10.1039/c8lc01037d
- Cui X, Boland T, D’Lima DD, Lotz MK. Thermal inkjet printing in tissue engineering and regenerative medicine. Recent Pat Drug Deliv Formul. 2012;6:149-155. doi: 10.2174/187221112800672949
- Guillemot F, Souquet A, Catros S, et al. High-throughput laser printing of cells and biomaterials for tissue engineering. Acta Biomater. 2010;6:2494-2500. doi: 10.1016/j.actbio.2009.09.029
- Pepper ME, Seshadri V, Burg TC, Burg KJ, Groff RE. Characterizing the effects of cell settling on bioprinter output. Biofabrication. 2012;4:011001. doi: 10.1088/1758-5082/4/1/011001
- Courson R, Bratash O, Maziz A, et al. Rapid prototyping of a polymer MEMS droplet dispenser by laser-assisted 3D printing. Microsyst Nanoeng. 2023;9:85. doi: 10.1038/s41378-023-00559-3
- Gruene M, Deiwick A, Koch L, et al. Laser printing of stem cells for biofabrication of scaffold-free autologous grafts. Tissue Eng Part C Methods. 2011;17:79-87. doi: 10.1089/ten.TEC.2010.0359
- Daly AC, Prendergast ME, Hughes AJ, Burdick JA. Bioprinting for the biologist. Cell. 2021;184:18-32. doi: 10.1016/j.cell.2020.12.002
- Askari M, Afzali Naniz M, Kouhi M, Saberi A, Zolfagharian A, Bodaghi M. Recent progress in extrusion 3D bioprinting of hydrogel biomaterials for tissue regeneration: a comprehensive review with focus on advanced fabrication techniques. Biomater Sci. 2021;9:535-573. doi: 10.1039/d0bm00973c
- Rocca M, Fragasso A, Liu W, Heinrich MA, Zhang YS. Embedded multimaterial extrusion bioprinting. SLAS Technol. 2018;23:154-163. doi: 10.1177/2472630317742071
- Xu L, Varkey M, Jorgensen A, et al. Bioprinting small diameter blood vessel constructs with an endothelial and smooth muscle cell bilayer in a single step. Biofabrication. 2020;12:045012. doi: 10.1088/1758-5090/aba2b6
- Lian L, Zhou C, Tang G, et al. Uniaxial and coaxial vertical embedded extrusion bioprinting. Adv Healthc Mater. 2022;11(9):e2102411. doi: 10.1002/adhm.202102411
- Malekpour A, Chen X. Printability and cell viability in extrusion-based bioprinting from experimental, computational, and machine learning views. J Funct Biomater. 2022;13(2):40. doi: 10.3390/jfb13020040
- Lan X, Adesida A, Boluk Y. Rheological and viscoelastic properties of collagens and their role in bioprinting by micro-extrusion. Biomed Mater. 2022;17(6). doi: 10.1088/1748-605X/ac9b06
- Tappa K, Jammalamadaka U. Novel biomaterials used in medical 3D printing techniques. J Funct Biomater. 2018;9(1):17. doi: 10.3390/jfb9010017
- Ismail KI, Yap TC, Ahmed R. 3D-printed fiber-reinforced polymer composites by fused deposition modelling (FDM): fiber length and fiber implementation techniques. Polymers (Basel). 2022;14(21):4659. doi: 10.3390/polym14214659
- Krueger L, Miles JA, Popat A. 3D printing hybrid materials using fused deposition modelling for solid oral dosage forms. J Control Release. 2022;351:444-455. doi: 10.1016/j.jconrel.2022.09.032
- Kumar H, Kim K. Stereolithography 3D bioprinting. Methods Mol Biol. 2020;2140:93-108. doi: 10.1007/978-1-0716-0520-2_6
- Morano C, Pagnotta L. Additive manufactured parts produced using selective laser sintering technology: comparison between porosity of pure and blended polymers. Polymers (Basel). 2023;15(22):4446. doi: 10.3390/polym15224446
- Mazzoli A. Selective laser sintering in biomedical engineering. Med Biol Eng Comput. 2013;51(3):245-256. doi: 10.1007/s11517-012-1001-x
- Shirazi SF, Gharehkhani S, Mehrali M, et al. A review on powder-based additive manufacturing for tissue engineering: selective laser sintering and inkjet 3D printing. Sci Technol Adv Mater. 2015;16:033502. doi: 10.1088/1468-6996/16/3/033502
- Su X, Wang T, Guo S. Applications of 3D printed bone tissue engineering scaffolds in the stem cell field. Regen Ther. 2021;16:63-72. doi: 10.1016/j.reth.2021.01.007
- Kafle A, Luis E, Silwal R, Pan HM, Shrestha PL, Bastola AK. 3D/4D printing of polymers: fused deposition modelling (FDM), selective laser sintering (SLS), and stereolithography (SLA). Polymers (Basel). 2021;13(18):3101. doi: 10.3390/polym13183101
- Li YC, Zhang YS, Akpek A, Shin SR, Khademhosseini A. 4D bioprinting: the next-generation technology for biofabrication enabled by stimuli-responsive materials. Biofabrication. 2016;9:012001. doi: 10.1088/1758-5090/9/1/012001
- Ong CS, Nam L, Ong K, et al. 3D and 4D bioprinting of the myocardium: current approaches, challenges, and future prospects. Biomed Res Int. 2018;2018: 6497242. doi: 10.1155/2018/6497242
- Miao S, Cui H, Nowicki M, et al. Stereolithographic 4D bioprinting of multiresponsive architectures for neural engineering. Adv Biosyst. 2018;2(9):1800101. doi: 10.1002/adbi.201800101
- Hua M, Wu D, Wu S, Ma Y, Alsaid Y, He X. 4D printable tough and thermoresponsive hydrogels. ACS Appl Mater Interfaces. 2021;13:12689-12697. doi: 10.1021/acsami.0c17532
- Guo Y, Belgodere JA, Ma Y, Jung JP, Bharti B. Directed printing and reconfiguration of thermoresponsive Silica-pNIPAM nanocomposites. Macromol Rapid Commun. 2019;40(13):e1900191. doi: 10.1002/marc.201900191
- Pan HM, Goto A. Topology-dependent pH-responsive actuation and shape memory programming for biomimetic 4D printing. Macromol Rapid Commun. 2023;44:e2300074. doi: 10.1002/marc.202300074
- Tahouni Y, Cheng T, Lajewski S, et al. Codesign of biobased cellulose-filled filaments and mesostructures for 4D printing humidity responsive smart structures. 3D Print Addit Manuf. 2023;10(1):1-14. doi: 10.1089/3dp.2022.0061
- Wychowaniec JK, Brougham DF. Emerging magnetic fabrication technologies provide controllable hierarchically-structured biomaterials and stimulus response for biomedical applications. Adv Sci (Weinh). 2022;9:e2202278.doi: 10.1002/advs.202202278.
- Capakova Z, Radaszkiewicz KA, Acharya TH, et al. The biocompatibility of polyaniline and polypyrrole 2: doping with organic phosphonates. Mater Sci Eng C Mater Biol Appl. 2020;113:110986. doi: 10.1016/j.msec.2020.110986.
- Noroozi R, Bodaghi M, Jafari H, Zolfagharian A, Fotouhi M. Shape-adaptive metastructures with variable Bandgap regions by 4D printing. Polymers (Basel). 2020;12(3):519. doi: 10.3390/polym12030519
- Ashammakhi N, Ahadian S, Zengjie F, et al. Advances and future perspectives in 4D bioprinting. Biotechnol J. 2018;13:e1800148. doi: 10.1002/biot.201800148
- Kuribayashi-Shigetomi K, Onoe H, Takeuchi S. Cell origami: self-folding of three-dimensional cell-laden microstructures driven by cell traction force. PLoS One. 2012;7:e51085. doi: 10.1371/journal.pone.0051085
- Devillard R, Pages E, Correa MM, et al. Cell patterning by laser-assisted bioprinting. Methods Cell Biol. 2014;119:159-174. doi: 10.1016/B978-0-12-416742-1.00009-3
- Placone JK, Engler AJ. Recent advances in extrusion-based 3D printing for biomedical applications. Adv Healthc Mater. 2018;7:e1701161. doi: 10.1002/adhm.201701161.
- Hwang HH, Zhu W, Victorine G, Lawrence N, Chen S. 3D-printing of functional biomedical microdevices via light- and extrusion-based approaches. Small Methods. 2018;2(2):1700277. doi: 10.1002/smtd.201700277
- Murphy SV, Atala A. 3D bioprinting of tissues and organs. Nat Biotechnol. 2014;32:773-785. doi: 10.1038/nbt.2958
- Cadamuro F, Nicotra F, Russo L. 3D printed tissue models: from hydrogels to biomedical applications. J Control Release. 2023;354:726-745. doi: 10.1016/j.jconrel.2023.01.048
- Jia L, Hua Y, Zeng J, et al. Bioprinting and regeneration of auricular cartilage using a bioactive bioink based on microporous photocrosslinkable acellular cartilage matrix. Bioact Mater. 2022;16:66-81. doi: 10.1016/j.bioactmat.2022.02.032
- Ojansivu M, Rashad A, Ahlinder A, et al. Wood-based nanocellulose and bioactive glass modified gelatin-alginate bioinks for 3D bioprinting of bone cells. Biofabrication. 2019;11:035010. doi: 10.1088/1758-5090/ab0692
- Moncal KK, Ozbolat V, Datta P, Heo DN, Ozbolat IT. Thermally-controlled extrusion-based bioprinting of collagen. J Mater Sci Mater Med. 2019;30:55. doi: 10.1007/s10856-019-6258-2
- Benson K, Galla HJ, Kehr NS. Cell adhesion behavior in 3D hydrogel scaffolds functionalized with D- or L-aminoacids. Macromol Biosci. 2014;14:793-798. doi: 10.1002/mabi.201300485
- Sigaux N, Pourchet L, Breton P, Brosset S, Louvrier A, Marquette CA. 3D bioprinting:principles, fantasies and prospects. J Stomatol Oral Maxillofac Surg. 2019;120:128-132. doi: 10.1016/j.jormas.2018.12.014
- Yao B, Hu T, Cui X, Song W, Fu X, Huang S. Enzymatically degradable alginate/gelatin bioink promotes cellular behavior and degradation in vitro and in vivo. Biofabrication. 2019;11:045020. doi: 10.1088/1758-5090/ab38ef
- Duan B, Hockaday LA, Kang KH, Butcher JT. 3D bioprinting of heterogeneous aortic valve conduits with alginate/gelatin hydrogels. J Biomed Mater Res A. 2013;101:1255-1264. doi: 10.1002/jbm.a.34420
- Michael S, Sorg H, Peck CT, et al. Tissue engineered skin substitutes created by laser-assisted bioprinting form skin-like structures in the dorsal skin fold chamber in mice. PLoS One. 2013;8:e57741. doi: 10.1371/journal.pone.0057741
- Visser J, Peters B, Burger TJ, et al. Biofabrication of multi-material anatomically shaped tissue constructs. Biofabrication. 2013;5:035007. doi: 10.1088/1758-5082/5/3/035007
- Owens CM, Marga F, Forgacs G, Heesch CM. Biofabrication and testing of a fully cellular nerve graft. Biofabrication. 2013;5:045007. doi: 10.1088/1758-5082/5/4/045007
- Xiao S, Zhao T, Wang J, et al. Gelatin methacrylate (GelMA)- based hydrogels for cell transplantation: an effective strategy for tissue engineering. Stem Cell Rev Rep. 2019;15:664-679. doi: 10.1007/s12015-019-09893-4
- Ozbolat IT, Yu Y. Bioprinting toward organ fabrication: challenges and future trends. IEEE Trans Biomed Eng. 2013;60:691-699. doi: 10.1109/TBME.2013.2243912
- Kolesky DB, Truby RL, Gladman AS, Busbee TA, Homan KA, Lewis JA. 3D bioprinting of vascularized, heterogeneous cell-laden tissue constructs. Adv Mater. 2014;26:3124-3130. doi: 10.1002/adma.201305506
- Abdollahi Baghban S, Ebrahimi M, Bagheri-Khoulenjani S, Khorasani M. A highly efficient microwave-assisted synthesis of an LED-curable methacrylated gelatin for bio applications. RSC Adv. 2021;11:14996-15009. doi: 10.1039/d1ra01269j
- Purcell BP, Elser JA, Mu A, Margulies KB, Burdick JA. Synergistic effects of SDF-1alpha chemokine and hyaluronic acid release from degradable hydrogels on directing bone marrow derived cell homing to the myocardium. Biomaterials. 2012;33:7849-7857.doi: 10.1016/j.biomaterials.2012.07.005
- Burkhart A, Ritter H. Influence of cyclodextrin on the UCST- and LCST-behavior of poly(2-methacrylamido-caprolactam)-co-(N,N-dimethylacrylamide). Beilstein J Org Chem. 2014;10:1951-1958. doi: 10.3762/bjoc.10.203
- Yue K, Trujillo-de Santiago G, Alvarez MM, Tamayol A, Annabi N, Khademhosseini A. Synthesis, properties, and biomedical applications of gelatin methacryloyl (GelMA) hydrogels. Biomaterials. 2015;73:254-271. doi: 10.1016/j.biomaterials.2015.08.045
- Schuurmans CCL, Mihajlovic M, Hiemstra C, Ito K, Hennink WE, Vermonden T. Hyaluronic acid and chondroitin sulfate (meth)acrylate-based hydrogels for tissue engineering: synthesis, characteristics and pre-clinical evaluation. Biomaterials. 2021;268:120602. doi: 10.1016/j.biomaterials.2020.120602
- Nichol JW, Koshy ST, Bae H, Hwang CM, Yamanlar S, Khademhosseini A. Cell-laden microengineered gelatin methacrylate hydrogels. Biomaterials. 2010;31: 5536-5544. doi: 10.1016/j.biomaterials.2010.03.064
- Sun M, Sun X, Wang Z, Guo S, Yu G, Yang H. Synthesis and properties of gelatin methacryloyl (GelMA) hydrogels and their recent applications in load-bearing tissue. Polymers (Basel). 2018;10(11):1290. doi: 10.3390/polym10111290
- Zhu M, Wang Y, Ferracci G, Zheng J, Cho NJ, Lee BH. Gelatin methacryloyl and its hydrogels with an exceptional degree of controllability and batch-to-batch consistency. Sci Rep. 2019;9:6863. doi: 10.1038/s41598-019-42186-x
- Janmaleki M, Liu J, Kamkar M, Azarmanesh M, Sundararaj U, Nezhad AS. Role of temperature on bio-printability of gelatin methacryloyl bioink in two-step cross-linking strategy for tissue engineering applications. Biomed Mater. 2020;16:015021. doi: 10.1088/1748-605X/abbcc9
- He Q, Cheng Y, Deng Y, Wen F, Lai Y, Li H. Conductive hydrogel for flexible bioelectronic device: current progress and future perspective. Adv Funct Mater. 2024; 34(1):2308974. doi: 10.1002/adfm.202308974
- Goodarzi Hosseinabadi H, Dogan E, Miri AK, Ionov L. Digital light processing bioprinting advances for microtissue models. ACS Biomater Sci Eng. 2022;8:1381-1395. doi: 10.1021/acsbiomaterials.1c01509
- Li L, Scheiger JM, Levkin PA. Design and applications of photoresponsive hydrogels. Adv. Mater. 2019;31:e1807333. doi: 10.1002/adma.201807333
- Ruiz-Cantu L, Gleadall A, Faris C, Segal J, Shakesheff K, Yang J. Multi-material 3D bioprinting of porous constructs for cartilage regeneration. Mater Sci Eng C Mater Biol Appl. 2020;109:110578. doi: 10.1016/j.msec.2019.110578
- Raees S, Ullah F, Javed F, et al. Classification, processing, and applications of bioink and 3D bioprinting: a detailed review. Int J Biol Macromol.2023;232:123476. doi: 10.1016/j.ijbiomac.2023.123476.
- Lu D, Zeng Z, Geng Z, et al. Macroporous methacrylated hyaluronic acid hydrogel with different pore sizes for in vitro and in vivo evaluation of vascularization. Biomed Mater. 2022;17. doi: 10.1088/1748-605X/ac494b
- Chimene D, Kaunas R, Gaharwar AK. Hydrogel bioink reinforcement for additive manufacturing: a focused review of emerging strategies. Adv Mater. 2020;32:e1902026. doi: 10.1002/adma.201902026
- Klotz BJ, Gawlitta D, Rosenberg A, Malda J, Melchels FPW. Gelatin-methacryloyl hydrogels: towards biofabrication-based tissue repair. Trends Biotechnol. 2016;34:394-407. doi: 10.1016/j.tibtech.2016.01.002
- Kurian AG, Singh RK, Patel KD, Lee JH, Kim HW. Multifunctional GelMA platforms with nanomaterials for advanced tissue therapeutics. Bioact Mater. 2022;8:267-295. doi: 10.1016/j.bioactmat.2021.06.027
- Dong Z, Yuan Q, Huang K, Xu W, Liu G, Gu Z. Gelatin methacryloyl (GelMA)-based biomaterials for bone regeneration. RSC Adv. 2019;9:17737-17744. doi: 10.1039/c9ra02695a.
- Schuurman W, Levett PA, Pot MW, et al. Gelatin-methacrylamide hydrogels as potential biomaterials for fabrication of tissue-engineered cartilage constructs. Macromol Biosci. 2013;13:551-561. doi: 10.1002/mabi.201200471
- Zheng H, Zuo B. Functional silk fibroin hydrogels: preparation, properties and applications. J Mater Chem B. 2021;9:1238-1258. doi: 10.1039/d0tb02099k
- Liu H, Sun Z, Guo C. Chemical modification of silk proteins: current status and future prospects. Adv Fiber Mater. 2022;4:705-719. doi: 10.1007/s42765-022-00144-9.
- Sun W, Gregory DA, Tomeh MA, Zhao X. Silk fibroin as a functional biomaterial for tissue engineering. Int J Mol Sci. 2021;22(3):1499. doi: 10.3390/ijms22031499
- Kim SH, Hong H, Ajiteru O, et al. 3D bioprinted silk fibroin hydrogels for tissue engineering. Nat Protoc. 2021;16:5484-5532. doi: 10.1038/s41596-021-00622-1
- Kim SH, Seo YB, Yeon YK, et al. 4D-bioprinted silk hydrogels for tissue engineering. Biomaterials. 2020;260:120281. doi: 10.1016/j.biomaterials.2020.120281.
- Kim SH, Yeon YK, Lee JM, et al. Precisely printable and biocompatible silk fibroin bioink for digital light processing 3D printing. Nat Commun. 2018;9:1620. doi: 10.1038/s41467-018-03759-y
- Liu J, Shi L, Deng Y, et al. Silk sericin-based materials for biomedical applications. Biomaterials. 2022;287: 121638. doi: 10.1016/j.biomaterials.2022.121638
- Qi C, Liu J, Jin Y, et al. Photo-crosslinkable, injectable sericin hydrogel as 3D biomimetic extracellular matrix for minimally invasive repairing cartilage. Biomaterials. 2018;163:89-104. doi: 10.1016/j.biomaterials.2018.02.016
- Spada A, Emami J, Tuszynski JA, Lavasanifar A. The uniqueness of albumin as a Carrier in Nanodrug Delivery. Mol Pharm. 2021;18:1862-1894. doi: 10.1021/acs.molpharmaceut.1c00046
- Khanna S, Singh AK, Behera SP, Gupta S. Thermoresponsive BSA hydrogels with phase tunability. Mater Sci Eng C Mater Biol Appl. 2021;119:111590. doi: 10.1016/j.msec.2020.111590.
- Chen Y, Zhai MJ, Mehwish N, et al. Comparison of globular albumin methacryloyl and random-coil gelatin methacryloyl: preparation, hydrogel properties, cell behaviors, and mineralization. Int J Biol Macromol. 2022;204:692-708. doi: 10.1016/j.ijbiomac.2022.02.028
- Patrawalla NY, Kajave NS, Albanna MZ, Kishore V. Collagen and beyond: a comprehensive comparison of human ECM properties derived from various tissue sources for regenerative medicine applications. J Funct Biomater. 2023;14(7):363. doi: 10.3390/jfb14070363
- Marques CF, Diogo GS, Pina S, Oliveira JM, Silva TH, Reis RL. Collagen-based bioinks for hard tissue engineering applications: a comprehensive review. J Mater Sci Mater Med. 2019;30:32. doi: 10.1007/s10856-019-6234-x
- Wang Z, Yang Y, Gao Y, Xu Z, Yang S, Jin M. Establishing a novel 3D printing bioinks system with recombinant human collagen. Int J Biol Macromol. 2022;211:400-409. doi: 10.1016/j.ijbiomac.2022.05.088
- Mostert D, Jorba I, Groenen BGW, et al. Methacrylated human recombinant collagen peptide as a hydrogel for manipulating and monitoring stiffness-related cardiac cell behavior. iScience. 2023;26:106423. doi: 10.1016/j.isci.2023.106423
- Zhou J, Wen B, Xie H, et al. Advances in the preparation and assessment of the biological activities of chitosan oligosaccharides with different structural characteristics. Food Funct. 2021;12:926-951. doi: 10.1039/d0fo02768e
- Yang M, Wang H, Li K, Chen Z, Seamirumi DT. A new soft tissue constructed with chitosan for wound dressings-incorporating nanoparticles for medical and nursing therapeutic efficacy. Regen Ther. 2023;24:103-111. doi: 10.1016/j.reth.2023.06.005
- Ailincai D, Cibotaru S, Anisiei A, et al. Marin, mesoporous chitosan nanofibers loaded with norfloxacin and coated with phenylboronic acid perform as bioabsorbable active dressings to accelerate the healing of burn wounds. Carbohydr Polym. 2023;318:121135. doi: 10.1016/j.carbpol.2023.121135.
- Yang Y, Ma Y, Wang J, et al. Chitosan-based mussel-inspired hydrogel for rapid self-healing and high adhesion of tissue adhesion and wound dressings. Carbohydr Polym. 2023;316:121083. doi: 10.1016/j.carbpol.2023.121083
- Wang W, Xue C, Mao X. Chitosan: structural modification, biological activity and application. Int J Biol Macromol. 2020;164:4532-4546. doi: 10.1016/j.ijbiomac.2020.09.042
- Shen Y, Tang H, Huang X, et al. DLP printing photocurable chitosan to build bio-constructs for tissue engineering. Carbohydr Polym. 2020;235:115970. doi: 10.1016/j.carbpol.2020.115970
- Aguirre G, Billon L. Water-borne synthesis of multi-responsive and biodegradable chitosan-crosslinked microgels: towards self-assembled films with adaptable properties. Carbohydr Polym. 2023;318:121099. doi: 10.1016/j.carbpol.2023.121099
- Song F, Kong Y, Shao C, et al. Chitosan-based multifunctional flexible hemostatic bio-hydrogel. Acta Biomater. 2021;136:170-183. doi: 10.1016/j.actbio.2021.09.056
- He X, Liu X, Yang J, et al. Tannic acid-reinforced methacrylated chitosan/methacrylated silk fibroin hydrogels with multifunctionality for accelerating wound healing. Carbohydr Polym. 2020;247:116689. doi: 10.1016/j.carbpol.2020.116689
- Wei H, Yu S, Zhang Y, et al. Injectable chitosan/xyloglucan composite hydrogel with mechanical adaptivity and endogenous bioactivity for skin repair. Carbohydr Polym. 2023;313:120904. doi: 10.1016/j.carbpol.2023.120904
- Yoon SJ, Kim SH, Choi JW, Chun HJ, Yang DH. Guided cortical and cancellous bone formation using a minimally invasive technique of BMSC- and BMP-2-laden visible light-cured carboxymethyl chitosan hydrogels. Int J Biol Macromol. 2023;227:641-653. doi: 10.1016/j.ijbiomac.2022.12.137
- Chen Y, Sheng W, Lin J, et al. Magnesium oxide nanoparticle coordinated phosphate-functionalized chitosan injectable hydrogel for osteogenesis and angiogenesis in bone regeneration. ACS Appl Mater Interfaces. 2022;14:7592-7608. doi: 10.1021/acsami.1c21260
- Carvalho CR, Wrobel S, Meyer C, et al. Gellan Gum-based luminal fillers for peripheral nerve regeneration: an in vivo study in the rat sciatic nerve repair model. Biomater Sci. 2018;6:1059-1075. doi: 10.1039/c7bm01101f
- Chen F, Huang G, Huang H. Preparation and application of dextran and its derivatives as carriers. Int J Biol Macromol. 2020;145:827-834. doi: 10.1016/j.ijbiomac.2019.11.151
- Sun G, Mao JJ. Engineering dextran-based scaffolds for drug delivery and tissue repair. Nanomedicine (Lond). 2012;7:1771-1784. doi: 10.2217/nnm.12.149
- Yang R, Xue W, Liao H, et al. Injectable polylysine and dextran hydrogels with robust antibacterial and ROS-scavenging activity for wound healing. Int J Biol Macromol. 2022;223:950-960. doi: 10.1016/j.ijbiomac.2022.11.065
- Zhao B, Zhang Y, Li D, Mo X, Pan J. Hofmeister effect-enhanced gelatin/oxidized dextran hydrogels with improved mechanical properties and biocompatibility for wound healing. Acta Biomater. 2022;151:235-253. doi: 10.1016/j.actbio.2022.08.009
- Kolahdoozan M, Rahimi T, Taghizadeh A, Aghaei H. Preparation of new hydrogels by visible light cross-linking of dextran methacrylate and poly(ethylene glycol)-maleic acid copolymer. Int J Biol Macromol. 2023;227:1221-1233. doi: 10.1016/j.ijbiomac.2022.11.309
- Huang S, Liu H, Huang S, Fu T, Xue W, Guo R. Dextran methacrylate hydrogel microneedles loaded with doxorubicin and trametinib for continuous transdermal administration of melanoma. Carbohydr Polym. 2020;246:116650. doi: 10.1016/j.carbpol.2020.116650
- Wang H, Zhou L, Liao J, et al. Cell-laden photocrosslinked GelMA-DexMA copolymer hydrogels with tunable mechanical properties for tissue engineering. J Mater Sci Mater Med. 2014;25:2173-2183. doi: 10.1007/s10856-014-5261-x
- Sun G, Zhang X, Shen YI, et al. Dextran hydrogel scaffolds enhance angiogenic responses and promote complete skin regeneration during burn wound healing. Proc Natl Acad Sci U S A. 2011;108: 20976-20981. doi: 10.1073/pnas.1115973108
- Dhamecha D, Movsas R, Sano U, Menon JU. Applications of alginate microspheres in therapeutics delivery and cell culture: past, present and future. Int J Pharm. 2019;569:118627. doi: 10.1016/j.ijpharm.2019.118627
- Rastogi P, Kandasubramanian B. Review of alginate-based hydrogel bioprinting for application in tissue engineering. Biofabrication. 2019;11:042001. doi: 10.1088/1758-5090/ab331e
- Charron PN, Fenn SL, Poniz A, Oldinski RA. Mechanical properties and failure analysis of visible light crosslinked alginate-based tissue sealants. J Mech Behav Biomed Mater. 2016;59:314-321. doi: 10.1016/j.jmbbm.2016.02.003
- Samorezov JE, Morlock CM, Alsberg E. Dual ionic and photo-crosslinked alginate hydrogels for micropatterned spatial control of material properties and cell behavior. Bioconjug Chem. 2015;26:1339-1347. doi: 10.1021/acs.bioconjchem.5b00117
- Gao Y, Jin X. Dual crosslinked methacrylated alginate hydrogel micron fibers and tissue constructs for cell biology. Mar Drugs. 2019;17:557. doi: 10.3390/md17100557
- Garcia-Lizarribar A, Villasante A, Lopez-Martin JA, et al. 3D bioprinted functional skeletal muscle models have potential applications for studies of muscle wasting in cancer cachexia. Biomater Adv. 2023;150:213426. doi: 10.1016/j.bioadv.2023.213426
- Fenn SL, Miao T, Scherrer RM, Floreani RA. Dual-cross-linked methacrylated alginate sub-microspheres for intracellular chemotherapeutic delivery. ACS Appl Mater Interfaces. 2016;8:17775-17783. doi: 10.1021/acsami.6b03245
- Wang Y, Li S, Xu X, Tan Y, Liu XW, Fang J. Chemoenzymatic synthesis of homogeneous chondroitin polymers and its derivatives. Carbohydr Polym. 2020;232:115822. doi: 10.1016/j.carbpol.2019.115822
- Bishnoi M, Jain A, Hurkat P, Jain SK. Chondroitin sulphate: a focus on osteoarthritis. Glycoconj J. 2016;33:693-705. doi: 10.1007/s10719-016-9665-3
- Mencio CP, Hussein RK, Yu P, Geller HM. The role of chondroitin sulfate proteoglycans in nervous system development. J Histochem Cytochem. 2021;69:61-80. doi: 10.1369/0022155420959147
- Ida-Yonemochi H, Takeuchi K, Ohshima H. Role of chondroitin sulfate in the developmental and healing process of the dental pulp in mice. Cell Tissue Res. 2022;388:133-148. doi: 10.1007/s00441-022-03575-3
- Lin JZ, Duan MR, Lin N, Zhao WJ. The emerging role of the chondroitin sulfate proteoglycan family in neurodegenerative diseases. Rev Neurosci. 2021;32:737-750. doi: 10.1515/revneuro-2020-0146
- Yang S, Gigout S, Molinaro A, et al. Chondroitin 6-sulphate is required for neuroplasticity and memory in ageing. Mol Psychiatry. 2021;26:5658-5668. doi: 10.1038/s41380-021-01208-9
- Morla S. Glycosaminoglycans and glycosaminoglycan mimetics in cancer and inflammation. Int J Mol Sci. 2019;20(8):1963. doi: 10.3390/ijms20081963
- Grzanna MW, Au RY, Au AY, Rashmir AM, Frondoza CG. Avocado/soybean unsaponifiables, glucosamine and chondroitin sulfate combination inhibits proinflammatory cox-2 expression and prostaglandin e2 production in tendon-derived cells. J Med Food. 2020;23:139-146. doi: 10.1089/jmf.2019.0022
- Pudelko A, Wisowski G, Olczyk K, Kozma EM. The dual role of the glycosaminoglycan chondroitin-6-sulfate in the development, progression and metastasis of cancer. FEBS J. 2019;286:1815-1837. doi: 10.1111/febs.14748
- Ornell KJ, Lozada D, Phan NV, Coburn JM. Controlling methacryloyl substitution of chondroitin sulfate: injectable hydrogels with tunable long-term drug release profiles. J Mater Chem B. 2019;7:2151-2161. doi: 10.1039/c8tb03020k
- He Y, Sun M, Wang J, et al. Chondroitin sulfate microspheres anchored with drug-loaded liposomes play a dual antioxidant role in the treatment of osteoarthritis. Acta Biomater. 2022;151:512-527. doi: 10.1016/j.actbio.2022.07.052
- Liu C, Fan L, Xing J, et al. Inhibition of astrocytic differentiation of transplanted neural stem cells by chondroitin sulfate methacrylate hydrogels for the repair of injured spinal cord. Biomater Sci. 2019;7:1995-2008. doi: 10.1039/c8bm01363b
- Silva ED, Babo PS, Costa-Almeida R, et al. Multifunctional magnetic-responsive hydrogels to engineer tendon-to-bone interface. Nanomedicine. 2018;14:2375-2385. doi: 10.1016/j.nano.2017.06.002
- Le HV, Le Cerf D. Colloidal polyelectrolyte complexes from hyaluronic acid: preparation and biomedical applications. Small. 2022;18:e2204283. doi: 10.1002/smll.202204283.
- Xia C, Chen P, Mei S, et al. Photo-crosslinked HAMA hydrogel with cordycepin encapsulated chitosan microspheres for osteoarthritis treatment. Oncotarget. 2017;8: 2835-2849. doi: 10.18632/oncotarget.13748
- Mouser VH, Abbadessa A, Levato R, et al. Development of a thermosensitive HAMA-containing bio-ink for the fabrication of composite cartilage repair constructs. Biofabrication. 2017;9:015026. doi: 10.1088/1758-5090/aa6265
- Wei S, Wang Z, Liang X, et al. A composite hydrogel with antibacterial and promoted cell proliferation dual properties for healing of infected wounds. Am J Transl Res. 2023;15:4467-4486.
- Xu Z, Liu G, Liu P, et al. Hyaluronic acid-based glucose-responsive antioxidant hydrogel platform for enhanced diabetic wound repair. Acta Biomater. 2022;147:147-157. doi: 10.1016/j.actbio.2022.05.047
- Shen J, Chen A, Cai Z, et al. Exhausted local lactate accumulation via injectable nanozyme-functionalized hydrogel microsphere for inflammation relief and tissue regeneration. Bioact Mater. 2022;12:153-168. doi: 10.1016/j.bioactmat.2021.10.013
- Shen X, Li S, Zhao X, et al. Dual-crosslinked regenerative hydrogel for sutureless long-term repair of corneal defect. Bioact Mater. 2023;20:434-448. doi: 10.1016/j.bioactmat.2022.06.006
- Jung M, Han Y, Woo C, Ki CS. Pulmonary tissue-mimetic hydrogel niches for small cell lung cancer cell culture. J Mater Chem B. 2021;9:1858-1866. doi: 10.1039/d0tb02609c
- Schuurmans CCL, Abbadessa A, Bengtson MA, et al. Complex coacervation-based loading and tunable release of a cationic protein from monodisperse glycosaminoglycan microgels. Soft Matter. 2018;14:6327-6341. doi: 10.1039/c8sm00686e
- Wang L, Cao H, Jiang H, Fang Y, Jiang D. A novel 3D bio-printing “liver lobule” microtissue biosensor for the detection of AFB(1). Food Res Int. 2023;168:112778. doi: 10.1016/j.foodres.2023.112778
- Huang YC, Liu ZH, Kuo CY, Chen JP. Photo-crosslinked hyaluronic acid/carboxymethyl cellulose composite hydrogel as a dural substitute to prevent post-surgical adhesion. Int J Mol Sci. 2022;23:6177. doi: 10.3390/ijms23116177
- Yu Y, Xiao H, Tang G, et al. Biomimetic hydrogel derived from decellularized dermal matrix facilitates skin wounds healing. Mater Today Bio. 2023;21:100725. doi: 10.1016/j.mtbio.2023.100725
- Liu F, Wang X, Li Y, et al. Dendrimer-modified gelatin methacrylate hydrogels carrying adipose-derived stromal/ stem cells promote cartilage regeneration. Stem Cell Res Ther. 2022;13:26. doi: 10.1186/s13287-022-02705-6
- Lim KS, Levato R, Costa PF, et al. Bio-resin for high resolution lithography-based biofabrication of complex cell-laden constructs. Biofabrication. 2018;10:034101. doi: 10.1088/1758-5090/aac00c
- Simaan-Yameen H, Bar-Am O, Saar G, Seliktar D. Methacrylated fibrinogen hydrogels for 3D cell culture and delivery. Acta Biomater. 2023;164:94-110. doi: 10.1016/j.actbio.2023.03.046
- Powers JG, Higham C, Broussard K, Phillips TJ. Wound healing and treating wounds: chronic wound care and management. J Am Acad Dermatol. 2016;74:607-625; quiz625-606. doi: 10.1016/j.jaad.2015.08.070
- Wilkinson HN, Hardman MJ. Wound healing: cellular mechanisms and pathological outcomes. Open Biol. 2020;10:200223. doi: 10.1098/rsob.200223
- R Ibañez RI, do Amaral R, Reis RL, Marques AP, Murphy CM, O’Brien FJ. 3D-printed gelatin methacrylate scaffolds with controlled architecture and stiffness modulate the fibroblast phenotype towards dermal regeneration. Polymers (Basel). 2021;13(15):2510. doi: 10.3390/polym13152510
- Chen H, Ma X, Gao T, Zhao W, Xu T, Liu Z. Robot-assisted in situ bioprinting of gelatin methacrylate hydrogels with stem cells induces hair follicle-inclusive skin regeneration. Biomed Pharmacother. 2023;158:114140. doi: 10.1016/j.biopha.2022.114140
- Jin R, Cui Y, Chen H, et al. Three-dimensional bioprinting of a full-thickness functional skin model using acellular dermal matrix and gelatin methacrylamide bioink. Acta Biomater. 2021;131:248-261. doi: 10.1016/j.actbio.2021.07.012
- Zhang L, Yang G, Johnson BN, Jia X. Three-dimensional (3D) printed scaffold and material selection for bone repair. Acta Biomater. 2019;84:16-33. doi: 10.1016/j.actbio.2018.11.039
- Ji C, Qiu M, Ruan H, et al. Transcriptome analysis revealed the symbiosis niche of 3d scaffolds to accelerate bone defect healing. Adv Sci (Weinh). 2022;9:e2105194. doi: 10.1002/advs.202105194
- Beheshtizadeh N, Farzin A, Rezvantalab S, et al. 3D printing of complicated GelMA-coated Alginate/Tri-calcium silicate scaffold for accelerated bone regeneration. Int J Biol Macromol. 2023;229:636-653. doi: 10.1016/j.ijbiomac.2022.12.267
- Ma L, Wang X, Zhou Y, et al. Biomimetic Ti-6Al-4V alloy/gelatin methacrylate hybrid scaffold with enhanced osteogenic and angiogenic capabilities for large bone defect restoration. Bioact Mater. 2021;6:3437-3448. doi: 10.1016/j.bioactmat.2021.03.010
- Ghahri T, Salehi Z, Aghajanpour S, et al. Development of osteon-like scaffold-cell construct by quadruple coaxial extrusion-based 3D bioprinting of nanocomposite hydrogel. Biomater Adv. 2023;145:213254. doi: 10.1016/j.bioadv.2022.213254
- Alarcin E, Izbudak B, Yuce E, et al. Optimization of methacrylated gelatin /layered double hydroxides nanocomposite cell-laden hydrogel bioinks with high printability for 3D extrusion bioprinting. J Biomed Mater Res A. 2023;111:209-223. doi: 10.1002/jbm.a.37450
- Nie R, Sun Y, Lv H, et al. 3D printing of MXene composite hydrogel scaffolds for photothermal antibacterial activity and bone regeneration in infected bone defect models. Nanoscale. 2022;14:8112-8129. doi: 10.1039/d2nr02176e
- Wang L, Shen M, Hou Q, Wu Z, Xu J, Wang L. 3D printing of reduced glutathione grafted gelatine methacrylate hydrogel scaffold promotes diabetic bone regeneration by activating PI3K/Akt signaling pathway. Int J Biol Macromol. 2022;222:1175-1191. doi: 10.1016/j.ijbiomac.2022.09.236
- Dutta SD, Ganguly K, Hexiu J, Randhawa A, Moniruzzaman M, Lim KT. A 3D bioprinted nanoengineered hydrogel with photoactivated drug delivery for tumor apoptosis and simultaneous bone regeneration via macrophage immunomodulation. Macromol Biosci. 2023;23(9):e2300096. doi: 10.1002/mabi.202300096
- Liu G, Zhang B, Wan T, et al. A 3D-printed biphasic calcium phosphate scaffold loaded with platelet lysate/gelatin methacrylate to promote vascularization. J Mater Chem B. 2022;10:3138-3151. doi: 10.1039/d2tb00006g
- Xu Z, Qi X, Bao M, et al. Biomineralization inspired 3D printed bioactive glass nanocomposite scaffolds orchestrate diabetic bone regeneration by remodeling micromilieu. Bioact Mater. 2023;25:239-255. doi: 10.1016/j.bioactmat.2023.01.024
- Yang J, Fatima K, Zhou X, He C. Meticulously engineered three-dimensional-printed scaffold with microarchitecture and controlled peptide release for enhanced bone regeneration. Biomater Transl. 2024;5:69-83. doi: 10.12336/biomatertransl.2024.01.007
- Li S, Li Z, Yang J, Ha Y, Zhou X, He C. Inhibition of sympathetic activation by delivering calcium channel blockers from a 3D printed scaffold to promote bone defect repair. Adv Healthc Mater. 2022;11:e2200785. doi: 10.1002/adhm.202200785
- Zhang D, Fu Q, Fu H, Zeng J, Jia L, Chen M. 3D-bioprinted human lipoaspirate-derived cell-laden skin constructs for healing of full-thickness skin defects. Int J Bioprint. 2023;9:718. doi: 10.18063/ijb.718
- Yu K, Huangfu H, Qin Q, et al. Application of bone marrow-derived macrophages combined with bone mesenchymal stem cells in dual-channel three-dimensional bioprinting scaffolds for early immune regulation and osteogenic induction in rat calvarial defects. ACS Appl Mater Interfaces. 2022;14:47052-47065. doi: 10.1021/acsami.2c13557
- Yang Z, Cao F, Li H, et al. Microenvironmentally optimized 3D-printed TGFbeta-functionalized scaffolds facilitate endogenous cartilage regeneration in sheep. Acta Biomater. 2022;150:181-198. doi: 10.1016/j.actbio.2022.07.029
- Xie X, Wu S, Mou S, Guo N, Wang Z, Sun J. Microtissue-based bioink as a chondrocyte microshelter for DLP bioprinting. Adv Healthc Mater. 2022;11:e2201877. doi: 10.1002/adhm.202201877
- Galliger Z, Vogt CD, Helms HR, Panoskaltsis-Mortari A. Extracellular matrix microparticles improve GelMA bioink resolution for 3D bioprinting at ambient temperature. Macromol Mater Eng. 2022;307(10):2200196. doi: 10.1002/mame.202200196
- Zhu W, Cui H, Boualam B, et al. 3D bioprinting mesenchymal stem cell-laden construct with core-shell nanospheres for cartilage tissue engineering. Nanotechnology. 2018;29:185101. doi: 10.1088/1361-6528/aaafa1
- Grogan SP, Chung PH, Soman P, et al. Digital micromirror device projection printing system for meniscus tissue engineering. Acta Biomater. 2013;9:7218-7226. doi: 10.1016/j.actbio.2013.03.020
- Gong L, Li J, Zhang J, et al. An interleukin-4-loaded bi-layer 3D printed scaffold promotes osteochondral regeneration. Acta Biomater. 2020;117:246-260. doi: 10.1016/j.actbio.2020.09.039
- Gao F, Xu Z, Liang Q, et al. Osteochondral regeneration with 3d-printed biodegradable high-strength supramolecular polymer reinforced-gelatin hydrogel scaffolds. Adv Sci (Weinh). 2019;6:1900867. doi: 10.1002/advs.201900867
- Deng C, Yang J, He H, et al. 3D bio-printed biphasic scaffolds with dual modification of silk fibroin for the integrated repair of osteochondral defects. Biomater Sci. 2021;9:4891-4903. doi: 10.1039/d1bm00535a
- Chen P, Zheng L, Wang Y, et al. Desktop-stereolithography 3D printing of a radially oriented extracellular matrix/ mesenchymal stem cell exosome bioink for osteochondral defect regeneration. Theranostics. 2019;9:2439-2459. doi: 10.7150/thno.31017
- Jiang G, Li S, Yu K, et al. A 3D-printed PRP-GelMA hydrogel promotes osteochondral regeneration through M2 macrophage polarization in a rabbit model. Acta Biomater. 2021;128:150-162. doi: 10.1016/j.actbio.2021.04.010
- Irmak G, Gumusderelioglu M. Patients- and tissue-specific bio-inks with photoactivated PRP and methacrylated gelatin for the fabrication of osteochondral constructs, Mater Sci Eng C Mater Biol Appl. 2021;125:112092. doi: 10.1016/j.msec.2021.112092
- Zhang X, Yan Z, Guan G, et al. Polyethylene glycol diacrylate scaffold filled with cell-laden methacrylamide gelatin/ alginate hydrogels used for cartilage repair. J Biomater Appl. 2022;36:1019-1032. doi: 10.1177/08853282211044853
- Ding X, Gao J, Yu X, et al. 3D-printed porous scaffolds of hydrogels modified with TGF-beta1 binding peptides to promote in vivo cartilage regeneration and animal gait restoration. ACS Appl Mater Interfaces. 2022;14:15982-15995. doi: 10.1021/acsami.2c00761
- Gao J, Ding X, Yu X, et al. Cell-free bilayered porous scaffolds for osteochondral regeneration fabricated by continuous 3D-printing using nascent physical hydrogel as ink. Adv Healthc Mater. 2021;10:e2001404. doi: 10.1002/adhm.202001404
- Liu Y, Peng L, Li L, et al. 3D-bioprinted BMSC-laden biomimetic multiphasic scaffolds for efficient repair of osteochondral defects in an osteoarthritic rat model. Biomaterials. 2021;279:121216. doi: 10.1016/j.biomaterials.2021.121216
- Martyniak K, Lokshina A, Cruz MA, Karimzadeh M, Kemp R, Kean TJ. Biomaterial composition and stiffness as decisive properties of 3D bioprinted constructs for type II collagen stimulation. Acta Biomater. 2022;152:221-234. doi: 10.1016/j.actbio.2022.08.058
- Ege D, Hasirci V. Is 3D printing promising for osteochondral tissue regeneration? ACS Appl Bio Mater. 2023;6:1431-1444. doi: 10.1021/acsabm.3c00093
- Zhou X, Tenaglio S, Esworthy T, et al. Three-dimensional printing biologically inspired DNA-based gradient scaffolds for cartilage tissue regeneration. ACS Appl Mater Interfaces. 2020;12:33219-33228. doi: 10.1021/acsami.0c07918
- Guan J, Yuan FZ, Mao ZM, et al. Fabrication of 3D-printed interpenetrating hydrogel scaffolds for promoting chondrogenic differentiation. Polymers (Basel). 2021;13(13):2146. doi: 10.3390/polym13132146
- Xia H, Zhao D, Zhu H, et al. Lyophilized scaffolds fabricated from 3d-printed photocurable natural hydrogel for cartilage regeneration. ACS Appl Mater Interfaces. 2018;10:31704-31715. doi: 10.1021/acsami.8b10926
- Wang M, Zhao J, Luo Y, et al. 3D contour printing of anatomically mimetic cartilage grafts with microfiber-reinforced double-network bioink. Macromol Biosci. 2022;22:e2200179. doi: 10.1002/mabi.202200179
- Garcia-Lizarribar A, Fernandez-Garibay X, Velasco- Mallorqui F, Castano AG, Samitier J, Ramon-Azcon J. Composite biomaterials as long-lasting scaffolds for 3D bioprinting of highly aligned muscle tissue. Macromol Biosci. 2018;18:e1800167. doi: 10.1002/mabi.201800167.
- Ajiteru O, Choi KY, Lim TH, et al. A digital light processing 3D printed magnetic bioreactor system using silk magnetic bioink. Biofabrication. 2021;13(3). doi: 10.1088/1758-5090/abfaee
- Lee J, Lee H, Jin EJ, Ryu D, Kim GH. 3D bioprinting using a new photo-crosslinking method for muscle tissue restoration. NPJ Regen Med. 2023;8:18. doi: 10.1038/s41536-023-00292-5
- Boularaoui S, Shanti A, Lanotte M, et al. Nanocomposite conductive bioinks based on low-concentration gelma and mxene nanosheets/gold nanoparticles providing enhanced printability of functional skeletal muscle tissues. ACS Biomater Sci Eng. 2021;7:5810-5822. doi: 10.1021/acsbiomaterials.1c01193
- Koffler J, Zhu W, Qu X, et al. Biomimetic 3D-printed scaffolds for spinal cord injury repair. Nat Med. 2019;25:263-269. doi: 10.1038/s41591-018-0296-z
- Poongodi R, Chen YL, Yang TH, et al. Bio-scaffolds as cell or exosome carriers for nerve injury repair. Int J Mol Sci. 2021;22(24):13347. doi: 10.3390/ijms222413347
- Liu X, Song S, Chen Z, et al. Release of O-GlcNAc transferase inhibitor promotes neuronal differentiation of neural stem cells in 3D bioprinted supramolecular hydrogel scaffold for spinal cord injury repair, Acta Biomater. 2022;151:148-162. doi: 10.1016/j.actbio.2022.08.031
- Hamid OA, Eltaher HM, Sottile V, Yang J. 3D bioprinting of a stem cell-laden, multi-material tubular composite: an approach for spinal cord repair. Mater Sci Eng C Mater Biol Appl. 2021;120:111707. doi: 10.1016/j.msec.2020.111707
- Izadifar M, Chapman D, Babyn P, Chen X, Kelly ME. UV-assisted 3D bioprinting of nanoreinforced hybrid cardiac patch for myocardial tissue engineering. Tissue Eng Part C Methods. 2018;24:74-88. doi: 10.1089/ten.TEC.2017.0346
- Bejleri D, Streeter BW, Nachlas ALY, et al. A bioprinted cardiac patch composed of cardiac-specific extracellular matrix and progenitor cells for heart repair. Adv Healthc Mater. 2018;7:e1800672. doi: 10.1002/adhm.201800672
- Brazhkina O, Park JH, Park HJ, et al. Designing a 3D printing based auxetic cardiac patch with hiPSC-CMs for heart repair. J Cardiovasc Dev Dis. 2021;8(12):172. doi: 10.3390/jcdd8120172
- Lee S, Sani ES, Spencer AR, et al. Human-recombinant-elastin-based bioinks for 3D bioprinting of vascularized soft tissues. Adv Mater. 2020;32:e2003915. doi: 10.1002/adma.202003915
- Wang H, Guo Y, Hu Y, et al. Ultrasound-controlled nano oxygen carriers enhancing cell viability in 3D GelMA hydrogel for the treatment of myocardial infarction. Int J Biol Macromol. 2023;244:125139. doi: 10.1016/j.ijbiomac.2023.125139
- Mehrotra S, Singh RD, Bandyopadhyay A, Janani G, Dey S, Mandal BB. Engineering microsphere-loaded non-mulberry silk-based 3D bioprinted vascularized cardiac patches with oxygen-releasing and immunomodulatory potential. ACS Appl Mater Interfaces. 2021;13:50744-50759. doi: 10.1021/acsami.1c14118
- Ma N, Cheung DY, Butcher JT. Incorporating nanocrystalline cellulose into a multifunctional hydrogel for heart valve tissue engineering applications. J Biomed Mater Res A. 2022; 110:76-91. doi: 10.1002/jbm.a.37267
- Nachlas ALY, Li S, Streeter BW, et al. A multilayered valve leaflet promotes cell-laden collagen type I production and aortic valve hemodynamics. Biomaterials. 2020;240:119838. doi: 10.1016/j.biomaterials.2020.119838
- Mazzocchi A, Devarasetty M, Huntwork R, Soker S, Skardal A. Optimization of collagen type I-hyaluronan hybrid bioink for 3D bioprinted liver microenvironments. Biofabrication. 2018;11:015003. doi: 10.1088/1758-5090/aae543
- S. J, S. Velayudhan, A.K. Pr, Biocompatibility evaluation of antioxidant cocktail loaded gelatin methacrylamide as bioink for extrusion-based 3D bioprinting, Biomed Mater. 2023;18(4). doi: 10.1088/1748-605X/acd82f
- Mao Q, Wang Y, Li Y, et al. Fabrication of liver microtissue with liver decellularized extracellular matrix (dECM) bioink by digital light processing (DLP) bioprinting. Mater Sci Eng C Mater Biol Appl. 2020;109:110625. doi: 10.1016/j.msec.2020.110625
- Wu Y, Wenger A, Golzar H, Tang XS. 3D bioprinting of bicellular liver lobule-mimetic structures via microextrusion of cellulose nanocrystal-incorporated shear-thinning bioink. Sci Rep. 2020;10:20648. doi: 10.1038/s41598-020-77146-3
- Jang MJ, Bae SK, Jung YS, et al. Enhanced wound healing using a 3D printed VEGF-mimicking peptide incorporated hydrogel patch in a pig model. Biomed Mater. 2021;16(4). doi: 10.1088/1748-605X/abf1a8
- Lam T, Dehne T, Kruger JP, et al. Photopolymerizable gelatin and hyaluronic acid for stereolithographic 3D bioprinting of tissue-engineered cartilage. J Biomed Mater Res B Appl Biomater. 2019:107:2649-2657. doi: 10.1002/jbm.b.34354
- Yin P, Su W, Li T, et al. A modular hydrogel bioink containing microsphere-embedded chondrocytes for 3D-printed multiscale composite scaffolds for cartilage repair. iScience 2023;26:107349. doi: 10.1016/j.isci.2023.107349
- Sang S, Mao X, Cao Y, et al. 3D Bioprinting Using Synovium- Derived MSC-Laden Photo-Cross-Linked ECM Bioink for Cartilage Regeneration. ACS Appl Mater Interfaces. 2023. doi: 10.1021/acsami.2c19058
- Chen YW, Lin YH, Lin TL, et al. 3D-biofabricated chondrocyte-laden decellularized extracellular matrix-contained gelatin methacrylate auxetic scaffolds under cyclic tensile stimulation for cartilage regeneration, Biofabrication. 2023;15(4). doi: 10.1088/1758-5090/ace5e1
- Daly AC, Critchley SE, Rencsok EM, Kelly DJ. A comparison of different bioinks for 3D bioprinting of fibrocartilage and hyaline cartilage. Biofabrication. 2016;8:045002. doi: 10.1088/1758-5090/8/4/045002
- Yang Z, Zhao T, Gao C, et al. 3D-bioprinted difunctional scaffold for in situ cartilage regeneration based on aptamer-directed cell recruitment and growth factor-enhanced cell chondrogenesis. ACS Appl Mater Interfaces. 2021;13:23369-23383. doi: 10.1021/acsami.1c01844
- De Moor L, Fernandez S, Vercruysse C, et al. Hybrid bioprinting of chondrogenically induced human mesenchymal stem cell spheroids. Front Bioeng Biotechnol. 2020;8:484. doi: 10.3389/fbioe.2020.00484
- Wang B, Diaz-Payno PJ, Browe DC, et al. Affinity-bound growth factor within sulfated interpenetrating network bioinks for bioprinting cartilaginous tissues. Acta Biomater. 2021;128:130-142. doi: 10.1016/j.actbio.2021.04.016
- Kuo CY, Wilson E, Fuson A, et al. Repair of tympanic membrane perforations with customized bioprinted ear grafts using Chinchilla models. Tissue Eng Part A. 2018;24:527-535. doi: 10.1089/ten.TEA.2017.0246
- Hong H, Seo YB, Kim DY, et al. Digital light processing 3D printed silk fibroin hydrogel for cartilage tissue engineering. Biomaterials. 2020;232:119679. doi: 10.1016/j.biomaterials.2019.119679
- Bandyopadhyay A, Mandal BB, Bhardwaj N. 3D bioprinting of photo-crosslinkable silk methacrylate (SilMA)- polyethylene glycol diacrylate (PEGDA) bioink for cartilage tissue engineering. J Biomed Mater Res A. 2022;110:884-898. doi: 10.1002/jbm.a.37336
- Visscher DO, Lee H, van Zuijlen PPM, et al. A photo-crosslinkable cartilage-derived extracellular matrix bioink for auricular cartilage tissue engineering. Acta Biomater. 2021;121:193-203. doi: 10.1016/j.actbio.2020.11.029
- Cao Y, Cheng P, Sang S, et al. Mesenchymal stem cells loaded on 3D-printed gradient poly(epsilon-caprolactone)/ methacrylated alginate composite scaffolds for cartilage tissue engineering. Regen Biomater. 2021;8:rbab019. doi: 10.1093/rb/rbab019
- Abbadessa A, Blokzijl MM, Mouser VH, et al. A thermo-responsive and photo-polymerizable chondroitin sulfate-based hydrogel for 3D printing applications. Carbohydr Polym. 2016;149:163-174. doi: 10.1016/j.carbpol.2016.04.080
- Ma K, Zhao T, Yang L, et al. Application of robotic-assisted in situ 3D printing in cartilage regeneration with HAMA hydrogel: an in vivo study. J Adv Res. 2020;23:123-132. doi: 10.1016/j.jare.2020.01.010
- Kesti M, Muller M, Becher J, et al. A versatile bioink for three-dimensional printing of cellular scaffolds based on thermally and photo-triggered tandem gelation. Acta Biomater. 2015;11:162-172. doi: 10.1016/j.actbio.2014.09.033
- Zhang J, Tong D, Song H, et al. Osteoimmunity-regulating biomimetically hierarchical scaffold for augmented bone regeneration. Adv Mater. 2022;34:e2202044. doi: 10.1002/adma.202202044
- Gao J, Li M, Cheng J, et al. 3D-printed GelMA/PEGDA/ F127DA scaffolds for bone regeneration. J Funct Biomater. 2023;14:96. doi: 10.3390/jfb14020096
- Leu Alexa R, Cucuruz A, Ghitulica CD, et al. 3D printable composite biomaterials based on GelMA and hydroxyapatite powders doped with cerium ions for bone tissue regeneration. Int J Mol Sci. 2022;23:1841. doi: 10.3390/ijms23031841
- Li Z, Li S, Yang J, et al. 3D bioprinted gelatin/gellan gum-based scaffold with double-crosslinking network for vascularized bone regeneration. Carbohydr Polym. 2020;290:119469. doi: 10.1016/j.carbpol.2022.119469
- Liu G, Chen J, Wang X, Liu Y, Ma Y, Tu X. Functionalized 3D-printed ST2/gelatin methacryloyl/polcaprolactone scaffolds for enhancing bone regeneration with vascularization. Int J Mol Sci. 2022;23(15):8347. doi: 10.3390/ijms23158347
- Zhang X, Zhang H, Zhang Y, et al. 3D printed reduced graphene oxide-GelMA hybrid hydrogel scaffolds for potential neuralized bone regeneration. J Mater Chem B. 2023;11:1288-1301. doi: 10.1039/d2tb01979e
- Xu C, Chang Y, Xu Y, et al. Silicon-phosphorus-nanosheets-integrated 3D-printable hydrogel as a bioactive and biodegradable scaffold for vascularized bone regeneration. Adv Healthc Mater. 2022;11:e2101911. doi: 10.1002/adhm.202101911
- Jiang Y, Zhou D, Yang B. 3D bioprinted GelMA/GO composite induces osteoblastic differentiation. J Biomater Appl. 2022;37:527-537. doi: 10.1177/08853282221098235
- Ashwin B, Abinaya B, Prasith TP, et al. 3D-poly (lactic acid) scaffolds coated with gelatin and mucic acid for bone tissue engineering. Int J Biol Macromol. 2020;162:523-532. doi: 10.1016/j.ijbiomac.2020.06.157
- Tong L, Pu X, Liu Q, et al. Nanostructured 3D-printed hybrid scaffold accelerates bone regeneration by photointegrating nanohydroxyapatite. Adv Sci (Weinh). 2023;10:e2300038. doi: 10.1002/advs.202300038
- Pu X, Tong L, Wang X, et al. Bioinspired hydrogel anchoring 3DP GelMA/HAp scaffolds accelerates bone reconstruction. ACS Appl Mater Interfaces. 2022;14:20591-20602. doi: 10.1021/acsami.1c25015
- Leu Alexa R, Cucuruz A, Ghitulica CD, et al. 3D printed composite scaffolds of GelMA and hydroxyapatite nanopowders doped with Mg/Zn ions to evaluate the expression of genes and proteins of osteogenic markers. Nanomaterials (Basel). 2022;12(19):3420. doi: 10.3390/nano12193420
- Sun X, Yang J, Ma J, et al. Three-dimensional bioprinted BMSCs-laden highly adhesive artificial periosteum containing gelatin-dopamine and graphene oxide nanosheets promoting bone defect repair. Biofabrication. 2023;15(2). doi: 10.1088/1758-5090/acb73e
- Dong L, Bu Z, Xiong Y, et al. Facile extrusion 3D printing of gelatine methacrylate/Laponite nanocomposite hydrogel with high concentration nanoclay for bone tissue regeneration. Int J Biol Macromol. 2021;188:72-81. doi: 10.1016/j.ijbiomac.2021.07.199
- Yang X, Ma Y, Wang X, et al. A 3D-bioprinted functional module based on decellularized extracellular matrix bioink for periodontal regeneration. Adv Sci (Weinh). 2023;10:e2205041. doi: 10.1002/advs.202205041
- Ratheesh G, Vaquette C, Xiao Y. Patient-specific bone particles bioprinting for bone tissue engineering. Adv Healthc Mater. 2020;9(23):e2001323. doi: 10.1002/adhm.202001323
- Alcala-Orozco CR, Mutreja I, Cui X, Hooper GJ, Lim KS, Woodfield TBF. Hybrid biofabrication of 3D osteoconductive constructs comprising Mg-based nanocomposites and cell-laden bioinks for bone repair. Bone. 2022;154:116198. doi: 10.1016/j.bone.2021.116198
- Dutta SD, Ganguly K, Hexiu J, Randhawa A, Moniruzzaman M, Lim KT. A 3D bioprinted nanoengineered hydrogel with photoactivated drug delivery for tumor apoptosis and simultaneous bone regeneration via macrophage immunomodulation. Macromol Biosci. 2023;23:e2300096. doi: 10.1002/mabi.202300096
- Tavares MT, Gaspar VM, Monteiro MV, S Farinha JP, Baleizão C, Mano JF. GelMA/bioactive silica nanocomposite bioinks for stem cell osteogenic differentiation. Biofabrication. 2021;13(3). doi: 10.1088/1758-5090/abdc86
- Shahabipour F, Tavafoghi M, Aninwene GE, et al. Coaxial 3D bioprinting of tri-polymer scaffolds to improve the osteogenic and vasculogenic potential of cells in co-culture models. J Biomed Mater Res A. 2022;110:1077-1089. doi: 10.1002/jbm.a.37354
- Lee SH, Kang MS, Jeon S, et al. 3D bioprinting of human mesenchymal stem cells-laden hydrogels incorporating MXene for spontaneous osteodifferentiation. Heliyon. 2023;9:e14490. doi: 10.1016/j.heliyon.2023.e14490
- Ma Y, Ji Y, Zhong T, et al. Bioprinting-based PDLSC-ECM screening for in vivo repair of alveolar bone defect using cell-laden, injectable and photocrosslinkable hydrogels. ACS Biomater Sci Eng. 2017;3:3534-3545. doi: 10.1021/acsbiomaterials.7b00601
- Miao G, Liang L, Li W, et al. 3D bioprinting of a bioactive composite scaffold for cell delivery in periodontal tissue regeneration. Biomolecules. 2023;13(7):1062. doi: 10.3390/biom13071062
- Guo L, Chen H, Li Y, Zhou J, Chen J. Biocompatible scaffolds constructed by chondroitin sulfate microspheres conjugated 3D-printed frameworks for bone repair. Carbohydr Polym. 2023;299:120188. doi: 10.1016/j.carbpol.2022.120188
- Su JJ, Lin CH, Chen H, Lee SY, Lin YM. Biofabrication of cell-laden gelatin methacryloyl hydrogels with incorporation of silanized hydroxyapatite by visible light projection. Polymers (Basel). 2021;13(14):2354. doi: 10.3390/polym13142354
- Bucciarelli A, Petretta M, Grigolo B, et al. Methacrylated silk fibroin additive manufacturing of shape memory constructs with possible application in bone regeneration. Gels. 2022;8(12):833. doi: 10.3390/gels8120833
- Chang HK, Yang DH, Ha MY, et al. 3D printing of cell-laden visible light curable glycol chitosan bioink for bone tissue engineering. Carbohydr Polym. 2022;287:119328. doi: 10.1016/j.carbpol.2022.119328
- Ferroni L, D’Amora U, Leo S, et al. PEEK and hyaluronan-based 3d printed structures: promising combination to improve bone regeneration. Molecules. 2022;27(24):8749. doi: 10.3390/molecules27248749
- Kajave NS, Schmitt T, Patrawalla NY, Kishore V. Design-build-validate strategy to 3D print bioglass gradients for anterior cruciate ligament enthesis reconstruction. Tissue Eng Part C Methods. 2022;28:158-167. doi: 10.1089/ten.TEC.2022.0035
- Qiu C, Sun Y, Li J, et al. Therapeutic effect of biomimetic scaffold loaded with human amniotic epithelial cell-derived neural-like cells for spinal cord injury. Bioengineering (Basel). 2022;9(10):535. doi: 10.3390/bioengineering9100535
- Kim KS, Joo HJ, Choi SC, et al. Transplantation of 3D bio-printed cardiac mesh improves cardiac function and vessel formation via ANGPT1/Tie2 pathway in rats with acute myocardial infarction. Biofabrication. 2021;13(4). doi: 10.1088/1758-5090/ac1e78
- Hu Y, Jia Y, Wang H, et al. Low-intensity pulsed ultrasound promotes cell viability and inhibits apoptosis of H9C2 cardiomyocytes in 3D bioprinting scaffolds via PI3K-Akt and ERK1/2 pathways. J Biomater Appl. 2022; 37:402-414. doi: 10.1177/08853282221102669
- Ainsworth MJ, Chirico N, de Ruijter M, et al. Convergence of melt electrowriting and extrusion-based bioprinting for vascular patterning of a myocardial construct. Biofabrication. 2023;15(3). doi: 10.1088/1758-5090/ace07f
- Li H, Yin Y, Xiang Y, Liu H, Guo R. A novel 3D printing PCL/GelMA scaffold containing USPIO for MRI-guided bile duct repair. Biomed Mater. 2020;15:045004. doi: 10.1088/1748-605X/ab797a
- Cui J, Wang H, Shi Q, Sun T, Huang Q, Fukuda T. Multicellular co-culture in three-dimensional gelatin methacryloyl hydrogels for liver tissue engineering. Molecules. 2019;24(9):1762. doi: 10.3390/molecules24091762
- Cuvellier M, Rose S, Ezan F, et al. In vitrolong term differentiation and functionality of three-dimensional bioprinted primary human hepatocytes: application forin vivoengraftment. Biofabrication. 2022;14(3). doi: 10.1088/1758-5090/ac7825
- Li T, Hou J, Wang L, et al. Bioprinted anisotropic scaffolds with fast stress relaxation bioink for engineering 3D skeletal muscle and repairing volumetric muscle loss. Acta Biomater. 2023;156:21-36. doi: 10.1016/j.actbio.2022.08.037
- Hwangbo H, Lee H, Jin EJ, et al. Bio-printing of aligned GelMa-based cell-laden structure for muscle tissue regeneration. Bioact Mater. 2022;8:57-70. doi: 10.1016/j.bioactmat.2021.06.031
- Tavares-Negrete JA, Pedroza-Gonzalez SC, Frias-Sanchez AI, et al. Supplementation of GelMA with minimally processed tissue promotes the formation of densely packed skeletal-muscle-like tissues. ACS Biomater Sci Eng. 2023;9:3462-3475. doi: 10.1021/acsbiomaterials.2c01521
- Wang Y, Wang Q, Luo S, et al. 3D bioprinting of conductive hydrogel for enhanced myogenic differentiation. Regen Biomater. 2021;8:rbab035. doi: 10.1093/rb/rbab035
- Choi KY, Ajiteru O, Hong H, et al. A digital light processing 3D-printed artificial skin model and full-thickness wound models using silk fibroin bioink. Acta Biomater. 2023;164:159-174. doi: 10.1016/j.actbio.2023.04.034
- de Barros NR, Darabi MA, Ma X, et al. Enhanced maturation of 3D bioprinted skeletal muscle tissue constructs encapsulating soluble factor-releasing microparticles. Macromol Biosci. 2023;23(12):e2300276. doi: 10.1002/mabi.202300276
- Liu G, Guo Q, Liu C, et al. Cytomodulin-10 modified GelMA hydrogel with kartogenin for in-situ osteochondral regeneration. Acta Biomater. 2023;169:317-333. doi: 10.1016/j.actbio.2023.08.013
- Wang M, Li W, Luo Z, et al. A multifunctional micropore-forming bioink with enhanced anti-bacterial and anti-inflammatory properties. Biofabrication. 2022;14(2). doi: 10.1088/1758-5090/ac5936
- Wang C, Wang M, Xu T, et al. Engineering bioactive self-healing antibacterial exosomes hydrogel for promoting chronic diabetic wound healing and complete skin regeneration. Theranostics. 2019;9:65-76. doi: 10.7150/thno.29766
- Zhang FX, Liu P, Ding W, et al. Injectable mussel-inspired highly adhesive hydrogel with exosomes for endogenous cell recruitment and cartilage defect regeneration. Biomaterials. 2021;278:121169. doi: 10.1016/j.biomaterials.2021.121169
- Fan L, Liu C, Chen X, et al. Exosomes-loaded electroconductive hydrogel synergistically promotes tissue repair after spinal cord injury via immunoregulation and enhancement of myelinated axon growth. Adv Sci (Weinh). 2022;9:e2105586. doi: 10.1002/advs.202105586
- Khayambashi P, Iyer J, Pillai S, Upadhyay A, Zhang Y, Tran SD. Hydrogel encapsulation of mesenchymal stem cells and their derived exosomes for tissue engineering. Int J Mol Sci. 2021;22(2):684. doi: 10.3390/ijms22020684
- Born LJ, McLoughlin ST, Dutta D, et al. Sustained released of bioactive mesenchymal stromal cell-derived extracellular vesicles from 3D-printed gelatin methacrylate hydrogels. J Biomed Mater Res A. 2022;110:1190-1198. doi: 10.1002/jbm.a.37362
- Hu H, Zhang H, Bu Z, et al. Small extracellular vesicles released from bioglass/hydrogel scaffold promote vascularized bone regeneration by transferring miR-23a-3p. Int J Nanomedicine. 2022;17:6201-6220. doi: 10.2147/IJN.S389471
- Zhu B, Wang D, Pan H, et al. Three-in-one customized bioink for islet organoid: GelMA/ECM/PRP orchestrate pro-angiogenic and immunoregulatory function. Colloids Surf B Biointerfaces. 2023;221:113017. doi: 10.1016/j.colsurfb.2022.113017
- Cao W, Peng S, Yao Y, et al. A nanofibrous membrane loaded with doxycycline and printed with conductive hydrogel strips promotes diabetic wound healing in vivo. Acta Biomater. 2022;152:60-73. doi: 10.1016/j.actbio.2022.08.048
- Ferroni L, Gardin C, D’Amora U, et al. Exosomes of mesenchymal stem cells delivered from methacrylated hyaluronic acid patch improve the regenerative properties of endothelial and dermal cells. Biomater Adv. 2022;139:213000. doi: 10.1016/j.bioadv.2022.213000
- Liu H, Wang Y, Cui K, Guo Y, Zhang X, Qin J. Advances in hydrogels in organoids and organs-on-a-chip. Adv Mater. 2019;31:e1902042. doi: 10.1002/adma.201902042
- Cuvellier M, Ezan F, Oliveira H, et al. 3D culture of HepaRG cells in GelMa and its application to bioprinting of a multicellular hepatic model. Biomaterials. 2021;269:120611. doi: 10.1016/j.biomaterials.2020.120611
- Bhise NS, Manoharan V, Massa S, et al. A liver-on-a-chip platform with bioprinted hepatic spheroids. Biofabrication. 2016;8:014101. doi: 10.1088/1758-5090/8/1/014101
- Zhang YS, Davoudi F, Walch P, et al. Bioprinted thrombosis-on-a-chip. Lab Chip. 2016;16:4097-4105. doi: 10.1039/c6lc00380j
- Liu S, Wang Z, Chen X, et al. Multiscale anisotropic scaffold integrating 3D printing and electrospinning techniques as a heart-on-a-chip platform for evaluating drug-induced cardiotoxicity. Adv Healthc Mater. 2023;12(24):e2300719. doi: 10.1002/adhm.202300719
- van der Valk DC, van der Ven CFT, Blaser MC, et al. Engineering a 3D-bioprinted model of human heart valve disease using nanoindentation-based biomechanics. Nanomaterials (Basel). 2018;8(5):296. doi: 10.3390/nano8050296
- Sanchez-Salazar MG, Crespo-Lopez Oliver R, Ramos- Meizoso S, et al. 3D-printed tumor-on-chip for the culture of colorectal cancer microspheres: mass transport characterization and anti-cancer drug assays. Bioengineering (Basel). 2023;10(5):554. doi: 10.3390/bioengineering10050554
- Chen Z, Wang F, Zhang J, et al. Study on development of composite hydrogels with tunable structures and properties for tumor-on-a-chip research. Front Bioeng Biotechnol. 2020;8:611796. doi: 10.3389/fbioe.2020.611796
- Makarcyzk MJ, Li ZA, Yu I, et al. Creation of a knee joint-on-a-chip for modeling joint diseases and testing drugs. J Vis Exp. 2023;(191):10.3791/64186. doi: 10.3791/64186
- Vurat MT, Seker S, Lalegul-Ulker O, et al. Development of a multicellular 3D-bioprinted microtissue model of human periodontal ligament-alveolar bone biointerface: towards a pre-clinical model of periodontal diseases and personalized periodontal tissue engineering. Genes Dis. 2022;9:1008-1023. doi: 10.1016/j.gendis.2020.11.011
- Kim W, Lee H, Lee J, et al. Efficient myotube formation in 3D bioprinted tissue construct by biochemical and topographical cues. Biomaterials. 2020;230:119632. doi: 10.1016/j.biomaterials.2019.119632
- Ma X, Qu X, Zhu W, et al. Deterministically patterned biomimetic human iPSC-derived hepatic model via rapid 3D bioprinting. Proc Natl Acad Sci U S A. 2016;113:2206-2211. doi: 10.1073/pnas.1524510113
- Gu L, Jiang S, Xu X, et al. Size- and density-dependent acoustic differential bioassembly of spatially-defined heterocellular architecture. Biofabrication. 2022;15(1). doi: 10.1088/1758-5090/aca79c
- Wang D, Guo Y, Zhu J, et al. Hyaluronic acid methacrylate/ pancreatic extracellular matrix as a potential 3D printing bioink for constructing islet organoids. Acta Biomater. 2023;165:86-101. doi: 10.1016/j.actbio.2022.06.036
- Chen S, Tomov ML, Ning L, et al. Extrusion-based 3D bioprinting of adhesive tissue engineering scaffolds using hybrid functionalized hydrogel bioinks. Adv Biol (Weinh). 2023;7:e2300124. doi: 10.1002/adbi.202300124
- Li W, Wang M, Wang S, et al. An adhesive bioink toward biofabrication under wet conditions. Small. 2023;19(50):e2205078. doi: 10.1002/smll.202205078
- Wei K, Sun J, Gao Q, et al. 3D “honeycomb” cell/carbon nanofiber/gelatin methacryloyl (GelMA) modified screen-printed electrode for electrochemical assessment of the combined toxicity of deoxynivalenol family mycotoxins. Bioelectrochemistry. 2021;139:107743. doi: 10.1016/j.bioelechem.2021.107743
- Wan W, Li Z, Wang X, Tian F, Yang J. Surface-fabrication of fluorescent hydroxyapatite for cancer cell imaging and bio-printing applications. Biosensors (Basel). 2022;12(6):419. doi: 10.3390/bios12060419
- Liu S, Wang Z, Chen X, et al. Multiscale anisotropic scaffold integrating 3D printing and electrospinning techniques as a heart-on-a-chip platform for evaluating drug-induced cardiotoxicity. Adv Healthc Mater. 2023;12:e2300719. doi: 10.1002/adhm.202300719
- Liu J, Miller K, Ma X, et al. Direct 3D bioprinting of cardiac micro-tissues mimicking native myocardium. Biomaterials. 2020;256:120204. doi: 10.1016/j.biomaterials.2020.120204
- Wang J, Wu Y, Li G, et al. Engineering large-scale self-mineralizing bone organoids with bone matrix-inspired hydroxyapatite hybrid bioinks. Adv Mater. 2024;36:e2309875. doi: 10.1002/adma.202309875
- O’Donnell BT, Al-Ghadban S, Ives CJ, et al. Adipose tissue-derived stem cells retain their adipocyte differentiation potential in three-dimensional hydrogels and bioreactors (dagger). Biomolecules. 2020;10:1070. doi: 10.3390/biom10071070
- Nothdurfter D, Ploner C, Coraca-Huber DC, et al. 3D bioprinted, vascularized neuroblastoma tumor environment in fluidic chip devices for precision medicine drug testing. Biofabrication. 2022;14(3). doi: 10.1088/1758-5090/ac5fb7.
- Bhattacharyya A, Ham HW, Sonh J, et al. 3D bioprinting of complex tissue scaffolds with in situ homogeneously mixed alginate-chitosan-kaolin bioink using advanced portable biopen. Carbohydr Polym. 2023;317:121046. doi: 10.1016/j.carbpol.2023.121046