Miniature modelling while aiming for transplantation– Current challenges and future perspectives of lung bioprinting
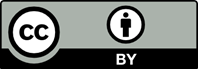
The development of three-dimensional (3D) lung organs or tissues using gravitational methods and bioprinting technologies shows great promise for producing lung tissue for research, pharmaceutical, and clinical applications. The advancement of innovative technologies can improve our understanding of lung diseases and, if necessary, enable the production of replacement lungs for transplantation. The development of functional organs-on-a-chip and disease-specific lung tissues could provide a deep understanding of the molecular mechanisms underlying lung diseases and aid the identification of drug targets. This knowledge has the potential to enhance our understanding of lung tissue regeneration processes and could lead to the development of more effective treatments for human lung diseases. So much so that lung transplants in most disease-induced cases would not be necessary, as the appropriate medications could induce regeneration of the damaged organ. This review highlights the importance of using a variety of materials, preparation methods, and sizes of lung tissues in 3D bioprinting technologies to understand lung function better, test for drug selection during therapy and eventually produce transplantable organs, if necessary. The review also emphasizes the need for improvements in legislation and guidelines for researchers aiming to achieve quality-assured biomanufacturing.