The application prospects of 4D printing tissue engineering materials in oral bone regeneration
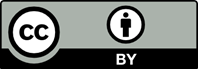
Researchers have developed smart shape-memory materials that adapt their structure or function to external stimuli. The demand for dynamic oral bone tissue repair is driven by continuous changes in bone and surrounding tissues during the repair process, such as tooth growth, movement, reconstruction of oral soft tissues, and skeletal differences in alveolar and craniofacial bones. These changes challenge the mechanical stability of bone implants and the precision of printing. Consequently, 4D printing technology introduces “time,” allowing pre-programmed changes in material shape or functionality, which enables scaffolds to respond to complex oral environments intelligently, achieving dynamic repair of bone and surrounding tissues. Despite its theoretical benefits in oral bone tissue engineering, the study and use of 4D printing technology is still in its infancy. This review explores the recent advances in 4D printing in dentistry, discussing skeletal structure, etiology of bone defects, and bone repair mechanisms. It also provides an overview of the materials, cells, and growth factors used in 4D printing bone tissue engineering. Thus, by reviewing existing studies, this review provides valuable insights for the future development of 4D printing technology in oral bone tissue engineering.

- Florencio-Silva R, Sasso GRDS, Sasso-Cerri E, Simões MJ, Cerri PS. Biology of bone tissue: structure, function, and factors that influence bone cells. BioMed Res Int. 2015;2015:1-17. doi: 10.1155/2015/421746
- Deng L, Yan Y. [Research status and progress of biomaterials for bone repair and reconstruction]. Zhongguo Xiu Fu Chong Jian Wai Ke Za Zhi Zhongguo Xiufu Chongjian Waike Zazhi Chin J Reparative Reconstr Surg. 2018;32(7):815-820. doi: 10.7507/1002-1892.201806028
- Freeman FE, Pitacco P, Van Dommelen LHA, et al. 3D bioprinting spatiotemporally defined patterns of growth factors to tightly control tissue regeneration. Sci Adv. 2020;6(33):eabb5093. doi: 10.1126/sciadv.abb5093
- Collins MN, Ren G, Young K, Pina S, Reis RL, Oliveira JM. Scaffold fabrication technologies and structure/function properties in bone tissue engineering. Adv Funct Mater. 2021;31(21):2010609. doi: 10.1002/adfm.202010609
- Moroni L, Burdick JA, Highley C, et al. Biofabrication strategies for 3D in vitro models and regenerative medicine. Nat Rev Mater. 2018;3(5):21-37. doi: 10.1038/s41578-018-0006-y
- Guo Y, Chi X, Wang Y, et al. Mitochondria transfer enhances proliferation, migration, and osteogenic differentiation of bone marrow mesenchymal stem cell and promotes bone defect healing. Stem Cell Res Ther. 2020;11(1):245. doi: 10.1186/s13287-020-01704-9
- Kuang X, Roach DJ, Wu J, et al. Advances in 4D printing: materials and applications. Adv Funct Mater. 2019;29(2):1805290. doi: 10.1002/adfm.201805290
- Wu JJ, Huang LM, Zhao Q, Xie T. 4D printing: history and recent progress. Chin J Polym Sci. 2018;36(5):563-575. doi: 10.1007/s10118-018-2089-8
- Haleem A, Javaid M, Vaishya R. 4D printing and its applications in orthopaedics. J Clin Orthop Trauma. 2018;9(3):275-276. doi: 10.1016/j.jcot.2018.08.016
- Gao B, Yang Q, Zhao X, Jin G, Ma Y, Xu F. 4D bioprinting for biomedical applications. Trends Biotechnol. 2016;34(9):746-756. doi: 10.1016/j.tibtech.2016.03.004
- Zolfagharian A, Kaynak A, Bodaghi M, Kouzani AZ, Gharaie S, Nahavandi S. Control-based 4D printing: adaptive 4D-printed systems. Appl Sci. 2020;10(9):3020. doi: 10.3390/app10093020
- Ge Q, Dunn CK, Qi HJ, Dunn ML. Active origami by 4D printing. Smart Mater Struct. 2014;23(9):094007. doi: 10.1088/0964-1726/23/9/094007
- Miao S, Zhu W, Castro NJ, et al. 4D printing smart biomedical scaffolds with novel soybean oil epoxidized acrylate. Sci Rep. 2016;6(1):27226. doi: 10.1038/srep27226
- Bodaghi M, Damanpack AR, Liao WH. Triple shape memory polymers by 4D printing. Smart Mater Struct. 2018;27(6):065010. doi: 10.1088/1361-665X/aabc2a
- Zhang F, Wang L, Zheng Z, Liu Y, Leng J. Magnetic programming of 4D printed shape memory composite structures. Compos Part Appl Sci Manuf. 2019;125:105571. doi: 10.1016/j.compositesa.2019.105571
- Nakielski P, Rybak D, Jezierska-Woźniak K, et al. Minimally invasive intradiscal delivery of BM-MSCs via fibrous microscaffold carriers. ACS Appl Mater Interfaces. 2023;15(50):58103-58118. doi: 10.1021/acsami.3c11710
- Lin C, Liu L, Liu Y, Leng J. 4D printing of bioinspired absorbable left atrial appendage occluders: a proof-of-concept study. ACS Appl Mater Interfaces. 2021;13(11):12668-12678. doi: 10.1021/acsami.0c17192
- Kitana W, Apsite I, Hazur J, Boccaccini AR, Ionov L. 4D biofabrication of T-shaped vascular bifurcation. Adv Mater Technol. 2023;8(1):2200429. doi: 10.1002/admt.202200429
- del Barrio J, Sánchez-Somolinos C. Light to shape the future: from photolithography to 4D printing. Adv Opt Mater. 2019;7(16):1900598. doi: 10.1002/adom.201900598
- Xie H, Yang KK, Wang YZ. Photo-cross-linking: a powerful and versatile strategy to develop shape-memory polymers. Prog Polym Sci. 2019;95:32-64. doi: 10.1016/j.progpolymsci.2019.05.001
- Wan X, Luo L, Liu Y, Leng J. Direct ink writing based 4D printing of materials and their applications. Adv Sci. 2020;7(16):2001000. doi: 10.1002/advs.202001000
- Castro NJ, Meinert C, Levett P, Hutmacher DW. Current developments in multifunctional smart materials for 3D/4D bioprinting. Curr Opin Biomed Eng. 2017;2:67-75. doi: 10.1016/j.cobme.2017.04.002
- Li X, Shang J, Wang Z. Intelligent materials: a review of applications in 4D printing. Assem Autom. 2017;37(2):170-185. doi: 10.1108/AA-11-2015-093
- Shin DG, Kim TH, Kim DE. Review of 4D printing materials and their properties. Int J Precis Eng Manuf-Green Technol. 2017;4(3):349-357. doi: 10.1007/s40684-017-0040-z
- Ashammakhi N, Ahadian S, Zengjie F, et al. Advances and future perspectives in 4D bioprinting. Biotechnol J. 2018;13(12):e1800148. doi: 10.1002/biot.201800148
- Liu T, Liu L, Zeng C, Liu Y, Leng J. 4D printed anisotropic structures with tailored mechanical behaviors and shape memory effects. Compos Sci Technol. 2020;186:107935. doi: 10.1016/j.compscitech.2019.107935
- Weerasinghe DK, Hodge JM, Pasco JA, Samarasinghe RM, Azimi Manavi B, Williams LJ. Antipsychotic-induced bone loss: the role of dopamine, serotonin and adrenergic receptor signalling. Front Cell Dev Biol. 2023;11:1184550. doi: 10.3389/fcell.2023.1184550
- Kenkre J, Bassett J. The bone remodelling cycle. Ann Clin Biochem. 2018;55(3):308-327. doi: 10.1177/0004563218759371
- Langdahl B, Ferrari S, Dempster DW. Bone modeling and remodeling: potential as therapeutic targets for the treatment of osteoporosis. Ther Adv Musculoskelet Dis. 2016;8(6):225-235. doi: 10.1177/1759720X16670154
- Shaheen MY, Basudan AM, de Vries RB, van den Beucken JJ, Jansen JA, Alghamdi H. S. Bone regeneration using antiosteoporotic drugs in adjunction with bone grafting: a meta-analysis. Tissue Eng Part B Rev. 2019;25(6): 500-509. doi: 10.1089/ten.teb.2019.0132
- Gosset A, Pouillès, JM, Trémollieres F. Menopausal hormone therapy for the management of osteoporosis. Best Pract Res Clin Endocrinol Metab. 2021;35(6):101551. doi: 10.1016/j.beem.2021.101551
- Fillingham Y, Jacobs J. Bone grafts and their substitutes. Bone Jt J. 2016;98-B(1_Supple_A):6-9. doi: 10.1302/0301-620X.98B.36350
- Qasim M, Chae DS, Lee NY. Advancements and frontiers in nano-based 3D and 4D scaffolds for bone and cartilage tissue engineering. Int J Nanomedicine. 2019;14:4333-4351. doi: 10.2147/IJN.S209431
- Haleem A, Javaid M, Vaishya R. 5D printing and its expected applications in orthopaedics. J Clin Orthop Trauma. 2019;10(4):809-810. doi: 10.1016/j.jcot.2018.11.014
- Vijayavenkataraman S, Yan WC, Lu WF, Wang CH, Fuh JYH. 3D bioprinting of tissues and organs for regenerative medicine. Adv Drug Deliv Rev. 2018;132:296-332. doi: 10.1016/j.addr.2018.07.004
- Wang W, Yeung KWK. Bone grafts and biomaterials substitutes for bone defect repair: a review. Bioact Mater. 2017;2(4):224-247. doi: 10.1016/j.bioactmat.2017.05.007
- Henkel J, Woodruff MA, Epari DR, et al. Bone regeneration based on tissue engineering conceptions – a 21st century perspective. Bone Res. 2013;1(1):216-248. doi: 10.4248/BR201303002
- Guo Y, Lv Z, Huo Y, et al. A biodegradable functional water-responsive shape memory polymer for biomedical applications. J Mater Chem B. 2019;7(1):123-132. doi: 10.1039/C8TB02462F
- Christy PN, Basha SK, Kumari VS, et al. Biopolymeric nanocomposite scaffolds for bone tissue engineering applications – a review. J Drug Deliv Sci Technol. 2020;55:101452. doi: 10.1016/j.jddst.2019.101452
- Lin X, Patil S, Gao YG, Qian A. The bone extracellular matrix in bone formation and regeneration. Front Pharmacol. 2020;11:757. doi: 10.3389/fphar.2020.00757
- Fraile-Martínez O, García-Montero C, Coca A, et al. Applications of polymeric composites in bone tissue engineering and jawbone regeneration. Polymers. 2021;13(19):3429. doi: 10.3390/polym13193429
- Gopal S, Multhaupt HAB, Couchman JR. Calcium in cell-extracellular matrix interactions. In: Islam MS, ed. Calcium Signaling. Cham: Springer International Publishing;2020:1079-1102. doi: 10.1007/978-3-030-12457-1_43
- Ciosek Ż, Kot K, Kosik-Bogacka D, Łanocha-Arendarczyk N, Rotter I. The effects of calcium, magnesium, phosphorus, fluoride, and lead on bone tissue. Biomolecules. 2021;11(4):506. doi: 10.3390/biom11040506
- Fakhry M, Hamade E, Badran B, Buchet R, Magne D. Molecular mechanisms of mesenchymal stem cell differentiation towards osteoblasts. World J Stem Cells. 2013;5(4):136-148. doi: 10.4252/wjsc.v5.i4.136
- Gu Q, Yang H, Shi Q. Macrophages and bone inflammation. J Orthop Transl. 2017;10:86-93. doi: 10.1016/j.jot.2017.05.002
- Wang JS, Mazur CM, Wein MN. Sclerostin and osteocalcin: candidate bone-produced hormones. Front Endocrinol. 2021;12:584147. doi: 10.3389/fendo.2021.584147
- Amarasekara DS, Kim S, Rho J. Regulation of osteoblast differentiation by cytokine networks. Int J Mol Sci. 2021;22(6):2851. doi: 10.3390/ijms22062851
- Hadjidakis DJ, Androulakis II. Bone remodeling. Ann NY Acad Sci. 2006;1092(1):385-396. doi: 10.1196/annals.1365.035
- Fuller K, Lawrence KM, Ross JL, et al. Cathepsin K inhibitors prevent matrix-derived growth factor degradation by human osteoclasts. Bone. 2008;42(1):200-211. doi: 10.1016/j.bone.2007.09.044
- Schemitsch EH. Size matters: defining critical in bone defect size!. J Orthop Trauma. 2017;31:S20. doi: 10.1097/BOT.0000000000000978
- Rowe P, Koller A, Sharma S. Physiology, bone remodeling. In: StatPearls. Treasure Island, FL:StatPearls Publishing;2024.
- You D, Chen G, Liu C, et al. 4D printing of multi-responsive membrane for accelerated in vivo bone healing via remote regulation of stem cell fate. Adv Funct Mater. 2021;31(40):2103920. doi: 10.1002/adfm.202103920
- Fang Z, Song H, Zhang Y, et al. Modular 4D printing via interfacial welding of digital light-controllable dynamic covalent polymer networks. Matter. 2020;2(5):1187-1197. doi: 10.1016/j.matt.2020.01.014
- Özcan M, Hotza D, Fredel MC, Cruz A, Volpato CAM. Materials and manufacturing techniques for polymeric and ceramic scaffolds used in implant dentistry. J Compos Sci. 2021;5(3):78. doi: 10.3390/jcs5030078
- Leist SK, Zhou J. Current status of 4D printing technology and the potential of light-reactive smart materials as 4D printable materials. Virtual Phys Prototyp. 2016;11(4):249-262. doi: 10.1080/17452759.2016.1198630
- Sordi MB, Cruz A, Fredel MC, Magini R, Sharpe PT. Three-dimensional bioactive hydrogel-based scaffolds for bone regeneration in implant dentistry. Mater Sci Eng C. 2021;124:112055. doi: 10.1016/j.msec.2021.112055
- Lim HK, Hong SJ, Byeon SJ, et al. 3D-printed ceramic bone scaffolds with variable pore architectures. Int J Mol Sci. 2020;21(18):6942. doi: 10.3390/ijms21186942
- Huang J, Xia S, Li Z, Wu X, Ren J. Applications of four-dimensional printing in emerging directions: review and prospects. J Mater Sci Technol. 2021;91:105-120. doi: 10.1016/j.jmst.2021.02.040
- Mallakpour S, Tabesh F, Hussain CM. 3D and 4D printing: from innovation to evolution. Adv Colloid Interface Sci. 2021;294:102482. doi: 10.1016/j.cis.2021.102482
- Saska S, Pilatti L, Blay A, Shibli JA. Bioresorbable polymers: advanced materials and 4D printing for tissue engineering. Polymers. 2021;13(4):563. doi: 10.3390/polym13040563
- Li YC, Zhang YS, Akpek A, Shin SR, Khademhosseini A. 4D bioprinting: the next-generation technology for biofabrication enabled by stimuli-responsive materials. Biofabrication. 2016;9(1):012001. doi: 10.1088/1758-5090/9/1/012001
- Mandon CA, Blum LJ, Marquette CA. 3D–4D printed objects: new bioactive material opportunities. Micromachines. 2017;8(4):102. doi: 10.3390/mi8040102
- Zhang Z, Demir KG, Gu GX. Developments in 4D-printing: a review on current smart materials, technologies, and applications. Int J Smart Nano Mater. 2019;10(3): 205-224. doi: 10.1080/19475411.2019.1591541
- Chu H, Yang W, Sun L, et al. 4D printing: a review on recent progresses. Micromachines. 2020;11(9):796. doi: 10.3390/mi11090796
- Melly SK, Liu L, Liu Y, Leng J. On 4D printing as a revolutionary fabrication technique for smart structures. Smart Mater Struct. 2020;29(8):083001. doi: 10.1088/1361-665X/ab9989
- Zhou W, Qiao Z, Nazarzadeh Zare E, et al. 4D-printed dynamic materials in biomedical applications: chemistry, challenges, and their future perspectives in the clinical sector. J Med Chem. 2020;63(15):8003-8024. doi: 10.1021/acs.jmedchem.9b02115
- Javaid M, Haleem A. Significant advancements of 4D printing in the field of orthopaedics. J Clin Orthop Trauma. 2020;11:S485-S490. doi: 10.1016/j.jcot.2020.04.021
- Momeni F, Mehdi Hassani MNS, Liu X, Ni J. A review of 4D printing. Mater Des. 2017;122:42-79. doi: 10.1016/j.matdes.2017.02.068
- Hoa SV, Cai X. Twisted composite structures made by 4D printing method. Compos Struct. 2020;238:111883. doi: 10.1016/j.compstruct.2020.111883
- Jeong HY, An SC, Lim Y, Jeong MJ, Kim N, Jun YC. 3D and 4D printing of multistable structures. Appl Sci. 2020;10(20):7254. doi: 10.3390/app10207254
- Bodaghi M, Damanpack AR, Liao WH. Self-expanding/ shrinking structures by 4D printing. Smart Mater Struct. 2016;25(10):105034. doi: 10.1088/0964-1726/25/10/105034
- Bhanushali H, Amrutkar S, Mestry S, Mhaske ST. Shape memory polymer nanocomposite: a review on structure-property relationship. Polym Bull. 2022;79(6):3437-3493. doi: 10.1007/s00289-021-03686-x
- Alshahrani HA. Review of 4D printing materials and reinforced composites: behaviors, applications and challenges. J Sci Adv Mater Devices. 2021;6(2):167-185. doi: 10.1016/j.jsamd.2021.03.006
- Sobacchi C, Erreni M, Strina D, Palagano E, Villa A, Menale C. 3D bone biomimetic scaffolds for basic and translational studies with mesenchymal stem cells. Int J Mol Sci. 2018;19(10):3150. doi: 10.3390/ijms19103150
- Park J, Park SY, Lee D, Song YS. Shape memory polymer composites embedded with hybrid ceramic microparticles. Smart Mater Struct. 2020;29(5):055037. doi: 10.1088/1361-665X/ab5e53
- Subash A, Kandasubramanian B. 4D Printing of shape memory polymers. Eur Polym J. 2020;134:109771. doi: 10.1016/j.eurpolymj.2020.109771
- Hendrikson WJ, Rouwkema J, Clementi F, Blitterswijk CA van, Farè S, Moroni L. Towards 4D printed scaffolds for tissue engineering: exploiting 3D shape memory polymers to deliver time-controlled stimulus on cultured cells. Biofabrication. 2017;9(3):031001. doi: 10.1088/1758-5090/aa8114
- Stroganov V, Pant J, Stoychev G, et al. 4D biofabrication: 3D cell patterning using shape-changing films. Adv Funct Mater. 2018;28(11):1706248. doi: 10.1002/adfm.201706248
- Erndt-Marino JD, Munoz-Pinto DJ, Samavedi S, et al. Evaluation of the osteoinductive capacity of polydopamine-coated poly(ε-caprolactone) diacrylate shape memory foams. ACS Biomater Sci Eng. 2015;1(12):1220-1230. doi: 10.1021/acsbiomaterials.5b00445
- Buffington SL, Paul JE, Ali MM, Macios MM, Mather PT, Henderson JH. Enzymatically triggered shape memory polymers. Acta Biomater. 2019;84:88-97. doi: 10.1016/j.actbio.2018.11.031
- Rajeshkumar G, Arvindh Seshadri S, Devnani GL, et al. Environment friendly, renewable and sustainable poly lactic acid (PLA) based natural fiber reinforced composites – a comprehensive review. J Clean Prod. 2021;310:127483. doi: 10.1016/j.jclepro.2021.127483
- Langford T, Mohammed A, Essa K, Elshaer A, Hassanin H. 4D printing of origami structures for minimally invasive surgeries using functional scaffold. Appl Sci. 2021;11(1):332. doi: 10.3390/app11010332
- Li Y, Zhang F, Liu Y, Leng J. 4D printed shape memory polymers and their structures for biomedical applications. Sci China Technol Sci. 2020;63(4):545-560. doi: 10.1007/s11431-019-1494-0
- Shuai L, Jun Z, Jianjun C, Ming Y, Xuepeng L, Zhiguo J. Biodegradable body temperature-responsive shape memory polyurethanes with self-healing behavior. Polym Eng Sci. 2019;59(s2):E310-E316. doi: 10.1002/pen.25061
- Yang W, Guan D, Liu J, Luo Y, Wang Y. Synthesis and characterization of biodegradable linear shape memory polyurethanes with high mechanical performance by incorporating novel long chain diisocyanates. New J Chem. 2020;44(8):3493-3503. doi: 10.1039/C9NJ06017K
- Zhang Y, Hu J, Xie R, et al. A programmable, fast-fixing, osteo-regenerative, biomechanically robust bone screw. Acta Biomater. 2020;103:293-305. doi: 10.1016/j.actbio.2019.12.017
- Zhang C, Cai D, Liao P, et al. 4D printing of shape-memory polymeric scaffolds for adaptive biomedical implantation. Acta Biomater. 2021;122:101-110. doi: 10.1016/j.actbio.2020.12.042
- Le Fer G, Becker ML. 4D printing of resorbable complex shape-memory poly(propylene fumarate) star scaffolds. ACS Appl Mater Interfaces. 2020;12(20):22444-22452. doi: 10.1021/acsami.0c01444
- Cemali G, Okutan B, Şafak EC, Köse GT. In vitro investigation of poly(propylene fumarate) cured with phosphonic acid based monomers as scaffolds for bone tissue engineering. J Polym Res. 2023;30(9):347. doi: 10.1007/s10965-023-03713-7
- Joshi S, Rawat K, Karunakaran C, et al. 4D printing of materials for the future: opportunities and challenges. Appl Mater Today. 2020;18:100490. doi: 10.1016/j.apmt.2019.100490
- Ahmed K, Shiblee MNI, Khosla A, Nagahara L, Thundat T, Furukawa H. Review – recent progresses in 4D printing of gel materials. J Electrochem Soc. 2020;167(3):037563. doi: 10.1149/1945-7111/ab6e60
- Kirillova A, Maxson R, Stoychev G, Gomillion CT, Ionov L. 4D biofabrication using shape-morphing hydrogels. Adv Mater. 2017;29(46):1703443. doi: 10.1002/adma.201703443
- Torbati AH, Mather PT. A hydrogel-forming liquid crystalline elastomer exhibiting soft shape memory. J Polym Sci Part B Polym Phys. 2016;54(1):38-52. doi: 10.1002/polb.23892
- Zhang Y, Wang Q, Yi S, et al. 4D printing of magnetoactive soft materials for on-demand magnetic actuation transformation. ACS Appl Mater Interfaces. 2021;13(3):4174-4184. doi: 10.1021/acsami.0c19280
- Ying Y, Li B, Liu C, Xiong Z, Bai W, Ma P. Shape-memory ECM-mimicking heparin-modified nanofibrous gelatin scaffold for enhanced bone regeneration in sinus augmentation. ACS Biomater Sci Eng. 2022;8(1):218-231. doi: 10.1021/acsbiomaterials.1c01365
- Diba M, Koons GL, Bedell ML, Mikos AG. 3D printed colloidal biomaterials based on photo-reactive gelatin nanoparticles. Biomaterials. 2021;274:120871. doi: 10.1016/j.biomaterials.2021.120871
- Yuan Z, Yuan X, Zhao Y, et al. Injectable GelMA cryogel microspheres for modularized cell delivery and potential vascularized bone regeneration. Small. 2021;17(11): 2006596. doi: 10.1002/smll.202006596
- Jiang LB, Su DH, Liu P, Ma YQ, Shao ZZ, Dong J. Shape-memory collagen scaffold for enhanced cartilage regeneration: native collagen versus denatured collagen. Osteoarthritis Cartilage. 2018;26(10):1389-1399. doi: 10.1016/j.joca.2018.06.004
- Luo Y, Lin X, Chen B, Wei X. Cell-laden four-dimensional bioprinting using near-infrared-triggered shape-morphing alginate/polydopamine bioinks. Biofabrication. 2019;11(4):045019. doi: 10.1088/1758-5090/ab39c5
- Hamann I, Gebhardt F, Eisenhut M, et al. Investigation into the hybrid production of a superelastic shape memory alloy with additively manufactured structures for medical implants. Materials. 2021;14(11):3098. doi: 10.3390/ma14113098
- Liu J, Lin Y, Bian D, et al. In vitro and in vivo studies of mg- 30sc alloys with different phase structure for potential usage within bone. Acta Biomater. 2019;98:50-66. doi: 10.1016/j.actbio.2019.03.009
- Werner M, Hammer N, Rotsch C, Berthold I, Leimert M. Experimental validation of adaptive pedicle screws – a novel implant concept using shape memory alloys. Med Biol Eng Comput. 2020;58(1):55-65. doi: 10.1007/s11517-019-02059-x
- Hou Z, Liu Z, Zhu X, et al. Contactless treatment for scoliosis by electromagnetically controlled shape-memory alloy rods: a preliminary study in rabbits. Eur Spine J. 2020;29(5):1147-1158. doi: 10.1007/s00586-019-06207-7
- Zhang Y, Li J, Soleimani M, et al. Biodegradable elastic sponge from nanofibrous biphasic calcium phosphate ceramic as an advanced material for regenerative medicine. Adv Funct Mater. 2021;31(40):2102911. doi: 10.1002/adfm.202102911
- Holman H, Kavarana MN, Rajab TK. Smart materials in cardiovascular implants: shape memory alloys and shape memory polymers. Artif Organs. 2021;45(5):454-463. doi: 10.1111/aor.13851
- Tamay DG, Dursun Usal T, Alagoz AS, Yucel D, Hasirci N, Hasirci V. 3D and 4D printing of polymers for tissue engineering applications. Front Bioeng Biotechnol. 2019;7:164. doi: 10.3389/fbioe.2019.00164
- Dessì M, Borzacchiello A, Mohamed THA, Abdel-Fattah WI, Ambrosio L. Novel biomimetic thermosensitive β-tricalcium phosphate/chitosan-based hydrogels for bone tissue engineering. J Biomed Mater Res A. 2013;101(10):2984-2993. doi: 10.1002/jbm.a.34592
- Wei H, Cheng SX, Zhang XZ, Zhuo RX. Thermo-sensitive polymeric micelles based on poly(N-isopropylacrylamide) as drug carriers. Prog Polym Sci. 2009;34(9):893-910. doi: 10.1016/j.progpolymsci.2009.05.002
- Ozturk N, Girotti A, Kose GT, Rodríguez-Cabello JC, Hasirci V. Dynamic cell culturing and its application to micropatterned, elastin-like protein-modified poly(N-isopropylacrylamide) scaffolds. Biomaterials. 2009;30(29):5417-5426. doi: 10.1016/j.biomaterials.2009.06.044
- Wang R, Wang X, Mu X, et al. Reducing thermal damage to adjacent normal tissue with dual thermo-responsive polymer via thermo-induced phase transition for precise photothermal theranosis. Acta Biomater. 2022;148:142-151. doi: 10.1016/j.actbio.2022.06.007
- Zu S, Zhang Z, Liu Q, et al. 4D printing of core-shell hydrogel capsules for smart controlled drug release. Bio-Des Manuf. 2022;5(2):294-304. doi: 10.1007/s42242-021-00175-y
- Adedoyin AA, Ekenseair AK. Biomedical applications of magneto-responsive scaffolds. Nano Res. 2018;11(10): 5049-5064. doi: 10.1007/s12274-018-2198-2
- Lucarini S, Hossain M, Garcia-Gonzalez D. Recent advances in hard-magnetic soft composites: synthesis, characterisation, computational modelling, and applications. Compos Struct. 2022;279:114800. doi: 10.1016/j.compstruct.2021.114800
- Zhang J, Zhao S, Zhu M, et al. 3D-printed magnetic Fe3O4/ MBG/PCL composite scaffolds with multifunctionality of bone regeneration, local anticancer drug delivery and hyperthermia. J Mater Chem B. 2014;2(43): 7583-7595. doi: 10.1039/C4TB01063A
- Meng J, Xiao B, Zhang Y, et al. Super-paramagnetic responsive nanofibrous scaffolds under static magnetic field enhance osteogenesis for bone repair in vivo. Sci Rep. 2013;3(1):2655. doi: 10.1038/srep02655
- Betsch M, Cristian C, Lin YY, et al. Incorporating 4D into bioprinting: real-time magnetically directed collagen fiber alignment for generating complex multilayered tissues. Adv Healthc Mater. 2018;7(21):1800894. doi: 10.1002/adhm.201800894
- Fuhrer R, Hofmann S, Hild N, et al. Pressureless mechanical induction of stem cell differentiation is dose and frequency dependent. Plos One. 2013;8(11):e81362. doi: 10.1371/journal.pone.0081362
- Zhang Q, Liu J, Yuan K, Zhang Z, Zhang X, Fang X. A multi-controlled drug delivery system based on magnetic mesoporous Fe3O4 nanopaticles and a phase change material for cancer thermo-chemotherapy. Nanotechnology. 2017;28(40):405101. doi: 10.1088/1361-6528/aa883f
- Kim C, Kim H, Park H, Lee KY. Controlling the porous structure of alginate ferrogel for anticancer drug delivery under magnetic stimulation. Carbohydr Polym. 2019;223:115045. doi: 10.1016/j.carbpol.2019.115045
- Alam Ansari MA, Dash M, Camci-Unal G, et al. Engineered stimuli-responsive smart grafts for bone regeneration. Curr Opin Biomed Eng. 2023;28:100493. doi: 10.1016/j.cobme.2023.100493
- Pourmasoumi P, Moghaddam A, Nemati Mahand S, et al. A review on the recent progress, opportunities, and challenges of 4D printing and bioprinting in regenerative medicine. J Biomater Sci Polym Ed. 2023;34(1):108-146. doi: 10.1080/09205063.2022.2110480
- Yang GH, Kim W, Kim J, Kim G. A skeleton muscle model using GelMA-based cell-aligned bioink processed with an electric-field assisted 3D/4D bioprinting. Theranostics. 2021;11(1):48-63. doi: 10.7150/thno.50794
- Lu H, Liu Y, Gou J, Leng J, Du S. Electrical properties and shape-memory behavior of self-assembled carbon nanofiber nanopaper incorporated with shape-memory polymer. Smart Mater Struct. 2010;19(7):075021. doi: 10.1088/0964-1726/19/7/075021
- Huang Y, Zhang L, Ji Y, et al. A non-invasive smart scaffold for bone repair and monitoring. Bioact Mater. 2023;19:499-510. doi: 10.1016/j.bioactmat.2022.04.034
- Municoy S, Álvarez Echazú MI, Antezana PE, et al. Stimuli-responsive materials for tissue engineering and drug delivery. Int J Mol Sci. 2020;21(13):4724. doi: 10.3390/ijms21134724
- Bedell ML, Navara AM, Du Y, Zhang S, Mikos AG. Polymeric systems for bioprinting. Chem Rev. 2020;120(19):10744-10792. doi: 10.1021/acs.chemrev.9b00834
- Ding A, Lee SJ, Ayyagari S, Tang R, Huynh CT, Alsberg E. 4D biofabrication via instantly generated graded hydrogel scaffolds. Bioact Mater. 2022;7:324-332. doi: 10.1016/j.bioactmat.2021.05.021
- Bagheri A, Arandiyan H, Boyer C, Lim M. Lanthanide-doped upconversion nanoparticles: emerging intelligent light-activated drug delivery systems. Adv Sci. 2016;3(7):1500437. doi: 10.1002/advs.201500437
- Zhang Y, Li C, Zhang W, et al. 3D-printed NIR-responsive shape memory polyurethane/magnesium scaffolds with tight-contact for robust bone regeneration. Bioact Mater. 2022;16:218-231. doi: 10.1016/j.bioactmat.2021.12.032
- Dong Q, Wan Z, Li Q, et al. 3D-printed near-infrared-light-responsive on-demand drug-delivery scaffold for bone regeneration. 2023. doi: 10.21203/rs.3.rs-2702534/v1
- Sydney Gladman A, Matsumoto EA, Nuzzo RG, Mahadevan L, Lewis JA. Biomimetic 4D printing. Nat Mater. 2016;15(4):413-418. doi: 10.1038/nmat4544
- Morouço, P, Azimi B, Milazzo M, et al. Four-dimensional (bio-)printing: a review on stimuli-responsive mechanisms and their biomedical suitability. Appl Sci. 2020;10(24):9143. doi: 10.3390/app10249143
- Liu Y, Li Y, Yang G, Zheng X, Zhou S. Multi-stimulus-responsive shape-memory polymer nanocomposite network cross-linked by cellulose nanocrystals. ACS Appl Mater Interfaces. 2015;7(7):4118-4126. doi: 10.1021/am5081056
- Zhou X, Guo L, Shi D, Duan S, Li J. Biocompatible chitosan nanobubbles for ultrasound-mediated targeted delivery of doxorubicin. Nanoscale Res Lett. 2019;14(1):24. doi: 10.1186/s11671-019-2853-x
- Cao Y, Chen Y, Yu T, et al. Drug release from phase-changeable nanodroplets triggered by low-intensity focused ultrasound. Theranostics. 2018;8(5):1327-1339. doi: 10.7150/thno.21492
- Yang P, Li D, Jin S, et al. Stimuli-responsive biodegradable poly(methacrylic acid) based nanocapsules for ultrasound traced and triggered drug delivery system. Biomaterials. 2014;35(6):2079-2088. doi: 10.1016/j.biomaterials.2013.11.057
- Li B, Zhang C, Peng F, Wang W, Vogt BD, Tan KT. 4D printed shape memory metamaterial for vibration bandgap switching and active elastic-wave guiding. J Mater Chem C. 2021;9(4):1164-1173. doi: 10.1039/D0TC04999A
- Kocak G, Tuncer C, Bütün V. pH-responsive polymers. Polym Chem. 2017;8(1):144-176. doi: 10.1039/C6PY01872F
- Xu Y, Han J, Chai Y, Yuan S, Lin H, Zhang X. Development of porous chitosan/tripolyphosphate scaffolds with tunable uncross-linking primary amine content for bone tissue engineering. Mater Sci Eng C. 2018;85:182-190. doi: 10.1016/j.msec.2017.12.032
- Qu J, Zhao X, Ma PX, Guo B. pH-responsive self-healing injectable hydrogel based on N-carboxyethyl chitosan for hepatocellular carcinoma therapy. Acta Biomater. 2017;58:168-180. doi: 10.1016/j.actbio.2017.06.001
- Mohy Eldin MS, Kamoun EA, Sofan MA, Elbayomi SM. L-arginine grafted alginate hydrogel beads: a novel pH-sensitive system for specific protein delivery. Arab J Chem. 2015;8(3):355-365. doi: 10.1016/j.arabjc.2014.01.007
- Bai T, Han Y, Zhang P, Wang W, Liu W. Zinc ion-triggered two-way macro-/microscopic shape changing and memory effects in high strength hydrogels with pre-programmed unilateral patterned surfaces. Soft Matter. 2012;8(25):6846-6852. doi: 10.1039/C2SM07364A
- Nan W, Wang W, Gao H, Liu W. Fabrication of a shape memory hydrogel based on imidazole – zinc ion coordination for potential cell-encapsulating tubular scaffold application. Soft Matter. 2013;9(1):132-137. doi: 10.1039/C2SM26918J
- Lai J, Ye X, Liu J, et al. 4D printing of highly printable and shape morphing hydrogels composed of alginate and methylcellulose. Mater Des. 2021;205:109699. doi: 10.1016/j.matdes.2021.109699
- Wells CM, Harris M, Choi L, Murali VP, Guerra FD, Jennings JA. Stimuli-responsive drug release from smart polymers. J Funct Biomater. 2019;10(3):34. doi: 10.3390/jfb10030034
- Kim J, Park Y, Tae G, et al. Synthesis and characterization of matrix metalloprotease sensitive-low molecular weight hyaluronic acid based hydrogels. J Mater Sci Mater Med. 2008;19(11):3311-3318. doi: 10.1007/s10856-008-3469-3
- Zhang C, Pan D, Li J, et al. Enzyme-responsive peptide dendrimer-gemcitabine conjugate as a controlled-release drug delivery vehicle with enhanced antitumor efficacy. Acta Biomater. 2017;55:153-162. doi: 10.1016/j.actbio.2017.02.047
- Wang B, Liu H, Sun L, et al. Construction of high drug loading and enzymatic degradable multilayer films for self-defense drug release and long-term biofilm inhibition. Biomacromolecules. 2018;19(1):85-93. doi: 10.1021/acs.biomac.7b01268
- Davaran S, Ghamkhari A, Alizadeh E, Massoumi B, Jaymand M. Novel dual stimuli-responsive ABC triblock copolymer: RAFT synthesis, “Schizophrenic” micellization, and its performance as an anticancer drug delivery nanosystem. J Colloid Interface Sci. 2017;488:282-293. doi: 10.1016/j.jcis.2016.11.002
- Li Z, Fan Z, Xu Y, et al. pH-sensitive and thermosensitive hydrogels as stem-cell carriers for cardiac therapy. ACS Appl Mater Interfaces. 2016;8(17):10752-10760. doi: 10.1021/acsami.6b01374
- Xu X, Huang Z, Huang Z, et al. Injectable, NIR/pH-responsive nanocomposite hydrogel as long-acting implant for chemophotothermal synergistic cancer therapy. ACS Appl Mater Interfaces. 2017;9(24):20361-20375. doi: 10.1021/acsami.7b02307
- IO de Solorzano, T Alejo, M Abad, et al. Cleavable and thermo-responsive hybrid nanoparticles for on-demand drug delivery. J Colloid Interface Sci. 2019;533: 171-181. doi: 10.1016/j.jcis.2018.08.069
- Bozuyuk U, Yasa O, Yasa IC, Ceylan H, Kizilel S, Sitti M. Light-triggered drug release from 3D-printed magnetic chitosan microswimmers. ACS Nano. 2018;12(9): 9617-9625. doi: 10.1021/acsnano.8b05997
- Collado-González M, García-Bernal D, Oñate-Sánchez RE, et al. Cytotoxicity and bioactivity of various pulpotomy materials on stem cells from human exfoliated primary teeth. Int Endod J. 2017;50(S2):e19-e30. doi: 10.1111/iej.12751
- Leberfinger AN, Dinda S, Wu Y, et al. Bioprinting functional tissues. Acta Biomater. 2019;95:32-49. doi: 10.1016/j.actbio.2019.01.009
- Unagolla JM, Jayasuriya AC. Hydrogel-based 3D bioprinting: a comprehensive review on cell-laden hydrogels, bioink formulations, and future perspectives. Appl Mater Today. 2020;18:100479. doi: 10.1016/j.apmt.2019.100479
- Haleem A, Javaid M, Singh RP, Suman R. Significant roles of 4D printing using smart materials in the field of manufacturing. Adv Ind Eng Polym Res. 2021;4(4):301-311. doi: 10.1016/j.aiepr.2021.05.001
- Li J, Wu C, Chu P, Gelinsky M. 3D printing of hydrogels: rational design strategies and emerging biomedical applications. Mater Sci Eng R Rep. 2020;140:100543. doi: 10.1016/j.mser.2020.100543
- Ng WL, An J, Chua CK. Process, material, and regulatory considerations for 3D printed medical devices and tissue constructs. Engineering. 2024;36:146-166. doi: 10.1016/j.eng.2024.01.028
- Basak S. Is 4D printing at the forefront of transformations in tissue engineering and beyond? Biomed Mater Devices. 2024;2(2):587-600. doi: 10.1007/s44174-024-00161-9
- Ghosh S, Chaudhuri S, Roy P, Lahiri D. 4D printing in biomedical engineering: a state-of-the-art review of technologies, biomaterials, and application. Regen Eng Transl Med. 2023;9(3):339-365. doi: 10.1007/s40883-022-00288-5
- Miao S, Zhu W, Castro NJ, Leng J, Zhang LG. Four-dimensional printing hierarchy scaffolds with highly biocompatible smart polymers for tissue engineering applications. Tissue Eng Part C Methods. 2016;22(10):952-963. doi: 10.1089/ten.tec.2015.0542
- Zhao T, Yu R, Li X, et al. 4D printing of shape memory polyurethane via stereolithography. Eur Polym J. 2018;101:120-126. doi: 10.1016/j.eurpolymj.2018.02.021
- González-Henríquez CM, Sarabia-Vallejos MA, Rodriguez- Hernandez J. Polymers for additive manufacturing and 4D-printing: materials, methodologies, and biomedical applications. Prog Polym Sci. 2019;94:57-116. doi: 10.1016/j.progpolymsci.2019.03.001
- Ali MH, Abilgaziyev A, Adair D. 4D printing: a critical review of current developments, and future prospects. Int J Adv Manuf Technol. 2019;105(1):701-717. doi: 10.1007/s00170-019-04258-0
- Senatov FS, Niaza KV, Zadorozhnyy MY, Maksimkin AV, Kaloshkin SD, Estrin YZ. Mechanical properties and shape memory effect of 3D-printed PLA-based porous scaffolds. J Mech Behav Biomed Mater. 2016;57:139-148. doi: 10.1016/j.jmbbm.2015.11.036
- Murphy SV, Atala A. 3D bioprinting of tissues and organs. Nat Biotechnol. 2014;32(8):773-785. doi: 10.1038/nbt.2958
- Mu Q, Wang L, Dunn CK, et al. Digital light processing 3D printing of conductive complex structures. Addit Manuf. 2017;18:74-83. doi: 10.1016/j.addma.2017.08.011
- Gou M, Qu X, Zhu W, et al. Bio-inspired detoxification using 3D-printed hydrogel nanocomposites. Nat Commun. 2014;5(1):3774. doi: 10.1038/ncomms4774
- DuRaine GD, Brown WE, Hu JC, Athanasiou KA. Emergence of scaffold-free approaches for tissue engineering musculoskeletal cartilages. Ann Biomed Eng. 2015;43(3):543-554. doi: 10.1007/s10439-014-1161-y
- Han Y, Li X, Zhang Y, Han Y, Chang F, Ding J. Mesenchymal stem cells for regenerative medicine. Cells. 2019;8(8):886. doi: 10.3390/cells8080886
- Zhai Q, Dong Z, Wang W, Li B, Jin Y. Dental stem cell and dental tissue regeneration. Front Med. 2019;13(2):152-159. doi: 10.1007/s11684-018-0628-x
- Hernández-Monjaraz B, Santiago-Osorio E, Monroy- García A, Ledesma-Martínez E, Mendoza-Núñez VM. Mesenchymal stem cells of dental origin for inducing tissue regeneration in periodontitis: a mini-review. Int J Mol Sci. 2018;19(4):944. doi: 10.3390/ijms19040944
- Ono N, Kronenberg HM. Bone repair and stem cells. Curr Opin Genet Dev. 2016;40:103-107. doi: 10.1016/j.gde.2016.06.012
- Shen C, Yang C, Xu S, Zhao H. Comparison of osteogenic differentiation capacity in mesenchymal stem cells derived from human amniotic membrane (AM), umbilical cord (UC), chorionic membrane (CM), and decidua (DC). Cell Biosci. 2019;9(1):17. doi: 10.1186/s13578-019-0281-3
- Friedenstein AJ, Chailakhyan RK, Gerasimov UV. Bone marrow osteogenic stem cells: in vitro cultivation and transplantation in diffusion chambers. Cell Prolif. 1987;20(3):263-272. doi: 10.1111/j.1365-2184.1987.tb01309.x
- Moncal KK, Tigli Aydın RS, Godzik KP, et al. Controlled co-delivery of pPDGF-B and pBMP-2 from intraoperatively bioprinted bone constructs improves the repair of calvarial defects in rats. Biomaterials. 2022;281:121333. doi: 10.1016/j.biomaterials.2021.121333
- Zhang Z, Yang X, Cao X, Qin A, Zhao J. Current applications of adipose-derived mesenchymal stem cells in bone repair and regeneration: a review of cell experiments, animal models, and clinical trials. Front Bioeng Biotechnol. 2022;10:942128. doi: 10.3389/fbioe.2022.942128
- Xu T, Yu X, Yang Q, Liu X, Fang J, Dai X. Autologous micro-fragmented adipose tissue as stem cell-based natural scaffold for cartilage defect repair. Cell Transplant. 2019;28(12):1709-1720. doi: 10.1177/0963689719880527
- Wang Z, Han L, Sun T, Wang W, Li X, Wu B. Osteogenic and angiogenic lineage differentiated adipose-derived stem cells for bone regeneration of calvarial defects in rabbits. J Biomed Mater Res A. 2021;109(4):538-550. doi: 10.1002/jbm.a.37036
- Conejero JA, Lee JA, Parrett BM, et al. Repair of palatal bone defects using osteogenically differentiated fat-derived stem cells. Plast Reconstr Surg. 2006; 117(3):857. doi: 10.1097/01.prs.0000204566.13979.c1
- Roberts SJ, van Gastel N, Carmeliet G, Luyten FP. Uncovering the periosteum for skeletal regeneration: the stem cell that lies beneath. Bone. 2015;70:10-18. doi: 10.1016/j.bone.2014.08.007
- Bahney CS, Zondervan RL, Allison P, et al. Cellular biology of fracture healing. J Orthop Res. 2019;37(1):35-50. doi: 10.1002/jor.24170
- Radtke CL, Nino-Fong R, Gonzalez BPE, Stryhn H, McDuffee LA. Characterization and osteogenic potential of equine muscle tissue- and periosteal tissue-derived mesenchymal stem cells in comparison with bone marrow-and adipose tissue-derived mesenchymal stem cells. Am J Vet Res. 2013;74(5):790-800. doi: 10.2460/ajvr.74.5.790
- Roberts SJ, Geris L, Kerckhofs G, Desmet E, Schrooten J, Luyten FP. The combined bone forming capacity of human periosteal derived cells and calcium phosphates. Biomaterials. 2011;32(19):4393-4405. doi: 10.1016/j.biomaterials.2011.02.047
- Nuti N, Corallo C, Chan BMF, Ferrari M, Gerami-Naini B. Multipotent differentiation of human dental pulp stem cells: a literature review. Stem Cell Rev Rep. 2016;12(5): 511-523. doi: 10.1007/s12015-016-9661-9
- Nada OA, El Backly RM. Stem cells from the apical papilla (SCAP) as a tool for endogenous tissue regeneration. Front Bioeng Biotechnol. 2018;6:103. doi: 10.3389/fbioe.2018.00103
- Huang GTJ, Garcia-Godoy F. 13 – stem cells and dental tissue reconstruction. In: Spencer P, Misra A, eds. Material-Tissue Interfacial Phenomena. Sawston, Cambridge: Woodhead Publishing; 2017:325-353. doi: 10.1016/B978-0-08-100330-5.00013-3
- Kang J, Fan W, Deng Q, He H, Huang F. Stem cells from the apical papilla: a promising source for stem cell-based therapy. BioMed Res Int. 2019;2019:e6104738. doi: 10.1155/2019/6104738
- Kérourédan O, Bourget JM, Rémy M, et al. Micropatterning of endothelial cells to create a capillary-like network with defined architecture by laser-assisted bioprinting. J Mater Sci Mater Med. 2019;30(2):28. doi: 10.1007/s10856-019-6230-1
- Gao H, Li B, Zhao L, Jin Y. Influence of nanotopography on periodontal ligament stem cell functions and cell sheet based periodontal regeneration. Int J Nanomedicine. 2015;10:4009-4027. doi: 10.2147/IJN.S83357
- Salar Amoli M, EzEldeen M, Jacobs R, Bloemen V. Materials for dentoalveolar bioprinting: current state of the art. Biomedicines. 2022;10(1):71. doi: 10.3390/biomedicines10010071
- Zhou T, Pan J, Wu P, et al. Dental follicle cells: roles in development and beyond. Stem Cells Int. 2019;2019:e9159605. doi: 10.1155/2019/9159605
- Dave JR, Chandekar SS, Behera S, et al. Human gingival mesenchymal stem cells retain their growth and immunomodulatory characteristics independent of donor age. Sci Adv. 2022;8(25):eabm6504. doi: 10.1126/sciadv.abm6504
- Zhang Q, Nguyen PD, Shi S, Burrell JC, Cullen DK, Le AD. 3D bio-printed scaffold-free nerve constructs with human gingiva-derived mesenchymal stem cells promote rat facial nerve regeneration. Sci Rep. 2018;8(1):6634. doi: 10.1038/s41598-018-24888-w
- Pore-forming bioinks to enable spatio-temporally defined gene delivery in bioprinted tissues. J Control Release. 2019;301:13-27. doi: 10.1016/j.jconrel.2019.03.006
- Pizzicannella J, Diomede F, Gugliandolo A, et al. 3D printing PLA/gingival stem cells/EVs upregulate miR-2861 and -210 during osteoangiogenesis commitment. Int J Mol Sci. 2019;20(13):3256. doi: 10.3390/ijms20133256
- Attalla R, Puersten E, Jain N, Selvaganapathy PR. 3D bioprinting of heterogeneous bi- and tri-layered hollow channels within gel scaffolds using scalable multi-axial microfluidic extrusion nozzle. Biofabrication. 2018;11(1):015012. doi: 10.1088/1758-5090/aaf7c7
- Liu X, Gaihre B, George MN, et al. 3D bioprinting of oligo(poly[ethylene glycol] fumarate) for bone and nerve tissue engineering. J Biomed Mater Res A. 2021;109(1):6-17. doi: 10.1002/jbm.a.37002
- Pierdomenico L, Bonsi L, Calvitti M, et al. Multipotent mesenchymal stem cells with immunosuppressive activity can be easily isolated from dental pulp. Transplantation. 2005;80(6):836. doi: 10.1097/01.tp.0000173794.72151.88
- La Noce M, Paino F, Spina A, et al. Dental pulp stem cells: state of the art and suggestions for a true translation of research into therapy. J Dent. 2014;42(7):761-768. doi: 10.1016/j.jdent.2014.02.018
- Jin R, Song G, Chai J, Gou X, Yuan G, Chen Z. Effects of concentrated growth factor on proliferation, migration, and differentiation of human dental pulp stem cells in vitro. J Tissue Eng. 2018;9:2041731418817505. doi: 10.1177/2041731418817505
- Park JH, Gillispie GJ, Copus JS, et al. The effect of BMP-mimetic peptide tethering bioinks on the differentiation of dental pulp stem cells (DPSCs) in 3D bioprinted dental constructs. Biofabrication. 2020;12(3):035029. doi: 10.1088/1758-5090/ab9492
- Karbanová J, Soukup T, Suchánek J, Pytlík R, Corbeil D, Mokrý, J. Characterization of dental pulp stem cells from impacted third molars cultured in low serum-containing medium. Cells Tissues Organs. 2010;193(6):344-365. doi: 10.1159/000321160
- Comparative analysis of in vitro osteo/odontogenic differentiation potential of human dental pulp stem cells (DPSCs) and stem cells from the apical papilla (SCAP). Arch Oral Biol. 2011;56(7):709-721. doi: 10.1016/j.archoralbio.2010.12.008
- Thattaruparambil Raveendran N, Vaquette C, Meinert C, Samuel Ipe D, Ivanovski S. Optimization of 3D bioprinting of periodontal ligament cells. Dent Mater. 2019;35(12):1683-1694. doi: 10.1016/j.dental.2019.08.114
- Rombouts C, Giraud T, Jeanneau C, About I. Pulp vascularization during tooth development, regeneration, and therapy. J Dent Res. 2017;96(2):137-144. doi: 10.1177/0022034516671688
- Miura M, Gronthos S, Zhao M, et al. SHED: stem cells from human exfoliated deciduous teeth. Proc Natl Acad Sci. 2003;100(10):5807-5812. doi: 10.1073/pnas.0937635100
- Yao S, Pan F, Prpic V, Wise GE. Differentiation of stem cells in the dental follicle. J Dent Res. 2008;87(8):767-771. doi: 10.1177/154405910808700801
- Handa K, Saito M, Tsunoda A, et al. Progenitor cells from dental follicle are able to form cementum matrix in vivo. Connect Tissue Res. 2002;43(2-3):406-408. doi: 10.1080/03008200290001023
- Abdal-Wahab M, Abdel Ghaffar KA, Ezzatt OM, Hassan AAA, El Ansary MMS, Gamal AY. Regenerative potential of cultured gingival fibroblasts in treatment of periodontal intrabony defects (randomized clinical and biochemical trial). J Periodontal Res. 2020;55(3):441-452. doi: 10.1111/jre.12728
- Yasui T, Mabuchi Y, Toriumi H, et al. Purified human dental pulp stem cells promote osteogenic regeneration. J Dent Res. 2016;95(2):206-214. doi: 10.1177/0022034515610748
- d’Aquino R, Papaccio G, Laino G, Graziano A. Dental pulp stem cells: a promising tool for bone regeneration. Stem Cell Rev. 2008;4(1):21-26. doi: 10.1007/s12015-008-9013-5
- Sonoyama W, Liu Y, Yamaza T, et al. Characterization of the apical papilla and its residing stem cells from human immature permanent teeth: a pilot study. J Endod. 2008;34(2):166-171. doi: 10.1016/j.joen.2007.11.021
- Cheng H, Huang Y, Yue H, Fan Y. Electrical stimulation promotes stem cell neural differentiation in tissue engineering. Stem Cells Int. 2021;2021:e6697574. doi: 10.1155/2021/6697574
- Iwasa SN, Babona-Pilipos R, Morshead CM. Environmental factors that influence stem cell migration: an “Electric Field.” Stem Cells Int. 2017;2017:e4276927. doi: 10.1155/2017/4276927
- Kusuyama J, Bandow K, Shamoto M, Kakimoto K, Ohnishi T, Matsuguchi T. Low intensity pulsed ultrasound (LIPUS) influences the multilineage differentiation of mesenchymal stem and progenitor cell lines through ROCK-Cot/ Tpl2-MEK-ERK signaling pathway*. J Biol Chem. 2014;289(15):10330-10344. doi: 10.1074/jbc.M113.546382
- Lew WZ, Huang YC, Huang KY, Lin CT, Tsai MT, Huang HM. Static magnetic fields enhance dental pulp stem cell proliferation by activating the p38 mitogen-activated protein kinase pathway as its putative mechanism. J Tissue Eng Regen Med. 2018;12(1):19-29. doi: 10.1002/term.2333
- Galli C, Pedrazzi G, Mattioli-Belmonte M, Guizzardi S. The use of pulsed electromagnetic fields to promote bone responses to biomaterials in vitro and in vivo. Int J Biomater. 2018;2018:e8935750. doi: 10.1155/2018/8935750
- Hwang NS, Varghese S, Elisseeff J. Controlled differentiation of stem cells. Adv Drug Deliv Rev. 2008;60(2):199-214. doi: 10.1016/j.addr.2007.08.036
- Orciani M, Fini M, Di Primio R, Mattioli-Belmonte M. Biofabrication and bone tissue regeneration: cell source, approaches, and challenges. Front Bioeng Biotechnol. 2017;5:17. doi: 10.3389/fbioe.2017.00017
- Wang Z, Wang Z, Lu WW, Zhen W, Yang D, Peng S. Novel biomaterial strategies for controlled growth factor delivery for biomedical applications. NPG Asia Mater. 2017;9(10):e435. doi: 10.1038/am.2017.171
- Mahmoudi Z, Sedighi M, Jafari A, et al. In situ 3D bioprinting: a promising technique in advanced biofabrication strategies. Bioprinting. 2023;31:e00260. doi: 10.1016/j.bprint.2023.e00260
- Kim J, Choi HS, Kim YM, Song SC. Thermo-responsive nanocomposite bioink with growth-factor holding and its application to bone regeneration. Small. 2023;19(9):2203464. doi: 10.1002/smll.202203464
- Nyberg E, Holmes C, Witham T, Grayson WL. Growth factor-eluting technologies for bone tissue engineering. Drug Deliv Transl Res. 2016;6(2):184-194. doi: 10.1007/s13346-015-0233-3
- De Witte TM, Wagner AM, Fratila-Apachitei LE, Zadpoor AA, Peppas NA. Immobilization of nanocarriers within a porous chitosan scaffold for the sustained delivery of growth factors in bone tissue engineering applications. J Biomed Mater Res A. 2020;108(5):1122-1135. doi: 10.1002/jbm.a.36887
- Cheng M, Qin G. Chapter 11 – progenitor cell mobilization and recruitment: SDF-1, CXCR4, Α4-Integrin, and c-Kit. In: Tang Y, ed. Progress in Molecular Biology and Translational Science, Genetics of Stem Cells, Part A. Cambridge, Massachusetts: Academic Press; 2012;111:243-264. doi: 10.1016/B978-0-12-398459-3.00011-3
- Lauer A, Wolf P, Mehler D, et al. Biofabrication of SDF-1 functionalized 3D-printed cell-free scaffolds for bone tissue regeneration. Int J Mol Sci. 2020;21(6):2175. doi: 10.3390/ijms21062175
- Tan J, Zhang M, Hai Z, et al. Sustained release of two bioactive factors from supramolecular hydrogel promotes periodontal bone regeneration. ACS Nano. 2019;13(5):5616-5622. doi: 10.1021/acsnano.9b00788
- Lopez CD, Diaz-Siso JR, Witek L, et al. Three dimensionally printed bioactive ceramic scaffold osseoconduction across critical-sized mandibular defects. J Surg Res. 2018;223:115-122. doi: 10.1016/j.jss.2017.10.027
- Dhavalikar P, Robinson A, Lan Z, et al. Review of integrin-targeting biomaterials in tissue engineering. Adv Healthc Mater. 2020;9(23):2000795. doi: 10.1002/adhm.202000795
- Alvarez P, Hee CK, Solchaga L, et al. Growth factors and craniofacial surgery. J Craniofac Surg. 2012;23(1):20. doi: 10.1097/SCS.0b013e318240c6a8
- Wang MM, Flores RL, Witek L, et al. Dipyridamole-loaded 3D-printed bioceramic scaffolds stimulate pediatric bone regeneration in vivo without disruption of craniofacial growth through facial maturity. Sci Rep. 2019;9(1):18439. doi: 10.1038/s41598-019-54726-6
- Maliha SG, Lopez CD, Coelho PG, et al. Bone tissue engineering in the growing calvaria using dipyridamole-coated, three-dimensionally-printed bioceramic scaffolds: construct optimization and effects on cranial suture patency. Plast Reconstr Surg. 2020;145(2):337e. doi: 10.1097/PRS.0000000000006483
- Nagayasu-Tanaka T, Anzai J, Takedachi M, Kitamura M, Harada T, Murakami S. Effects of combined application of fibroblast growth factor (FGF)-2 and carbonate apatite for tissue regeneration in a beagle dog model of one-wall periodontal defect. Regen Ther. 2023;23:84-93. doi: 10.1016/j.reth.2023.04.002
- Qu M, Jiang X, Zhou X, et al. Stimuli-responsive delivery of growth factors for tissue engineering. Adv Healthc Mater. 2020;9(7):1901714. doi: 10.1002/adhm.201901714
- Amler AK, Thomas A, Tüzüner S, et al. 3D bioprinting of tissue-specific osteoblasts and endothelial cells to model the human jawbone. Sci Rep. 2021;11(1):4876. doi: 10.1038/s41598-021-84483-4
- Kim D, Lee H, Lee GH, Hoang TH, Kim HR, Kim GH. Fabrication of bone-derived decellularized extracellular matrix/ceramic-based biocomposites and their osteo/ odontogenic differentiation ability for dentin regeneration. Bioeng Transl Med. 2022;7(3):e10317. doi: 10.1002/btm2.10317
- Lee UL, Yun S, Cao HL, et al. Bioprinting on 3D printed titanium scaffolds for periodontal ligament regeneration. Cells. 2021;10(6):1337. doi: 10.3390/cells10061337
- Lin YT, Hsu TT, Liu YW, Kao CT, Huang TH. Bidirectional differentiation of human-derived stem cells induced by biomimetic calcium silicate-reinforced gelatin methacrylate bioink for odontogenic regeneration. Biomedicines. 2021;9(8):929. doi: 10.3390/biomedicines9080929
- Kort-Mascort J, Bao G, Elkashty O, et al. Decellularized extracellular matrix composite hydrogel bioinks for the development of 3D bioprinted head and neck in vitro tumor models. ACS Biomater Sci Eng. 2021;7(11):5288-5300. doi: 10.1021/acsbiomaterials.1c00812
- Aguilar IN, Smith LJ, Olivos DJ, Chu TMG, Kacena MA, Wagner DR. Scaffold-free bioprinting of mesenchymal stem cells with the regenova printer: optimization of printing parameters. Bioprinting. 2019;15:e00048. doi: 10.1016/j.bprint.2019.e00048
- Aguilar IN, Olivos DJ, Brinker A, et al. Scaffold-free bioprinting of mesenchymal stem cells using the regenova printer: spheroid characterization and osteogenic differentiation. Bioprinting. 2019;15:e00050. doi: 10.1016/j.bprint.2019.e00050
- Hamza H. Dental 4D printing: an innovative approach. 2018;1(9):e17. doi: 10.30771/2018.4
- Javaid M, Haleem A, Singh RP, Rab S, Suman R, Kumar L. Significance of 4D printing for dentistry: materials, process, and potentials. J Oral Biol Craniofacial Res. 2022;12(3):388-395. doi: 10.1016/j.jobcr.2022.05.002
- Datta S, Rameshbabu AP, Bankoti K, et al. Decellularized bone matrix/oleoyl chitosan derived supramolecular injectable hydrogel promotes efficient bone integration. Mater Sci Eng C. 2021;119:111604. doi: 10.1016/j.msec.2020.111604
- Akbarinia S, Sadrnezhaad SK, Hosseini SA. Porous shape memory dental implant by reactive sintering of TiH2-Ni-urea mixture. Mater Sci Eng C. 2020;107:110213. doi: 10.1016/j.msec.2019.110213
- do Nascimento RO, Chirani N. 13 – shape-memory polymers for dental applications. In: Yahia L, ed. Shape Memory Polymers for Biomedical Applications. Woodhead Publishing Series in Biomaterials. Woodhead Publishing;2015:267-280. doi: 10.1016/B978-0-85709-698-2.00013-1
- Pfau MR, Beltran FO, Woodard LN, et al. Evaluation of a self-fitting, shape memory polymer scaffold in a rabbit calvarial defect model. Acta Biomater. 2021;136:233-242. doi: 10.1016/j.actbio.2021.09.041
- Uijlenbroek H, Liu Y, Wismeijer D. Soft tissue expansion: principles and inferred intraoral hydrogel tissue expanders. Dent Oral Craniofacial Res. 2016;1(6):178–185. doi: 10.15761/DOCR.1000140
- Malekmohammadi S, Sedghi Aminabad N, Sabzi A, et al. Smart and biomimetic 3D and 4D printed composite hydrogels: opportunities for different biomedical applications. Biomedicines. 2021;9(11):1537. doi: 10.3390/biomedicines9111537
- Naniz MA, Askari M, Zolfagharian A, Naniz MA, Bodaghi M. 4D printing: a cutting-edge platform for biomedical applications. Biomed Mater. 2022;17(6):062001. doi: 10.1088/1748-605X/ac8e42
- Hwangbo H, Lee H, Roh EJ, et al. Bone tissue engineering via application of a collagen/hydroxyapatite 4D-printed biomimetic scaffold for spinal fusion. Appl Phys Rev. 2021;8(2):021403. doi: 10.1063/5.0035601
- Liu S, Wang YN, Ma B, Shao J, Liu H, Ge S. Gingipain-responsive thermosensitive hydrogel loaded with SDF-1 facilitates in situ periodontal tissue regeneration. ACS Appl Mater Interfaces. 2021;13(31):36880-36893. doi: 10.1021/acsami.1c08855
- Wang B, Booij-Vrieling HE, Bronkhorst EM, et al. Antimicrobial and anti-inflammatory thermo-reversible hydrogel for periodontal delivery. Acta Biomater. 2020;116:259-267. doi: 10.1016/j.actbio.2020.09.018
- Wu D, Wang P, Wu Q, et al. Preparation and characterization of bomidin-loaded thermosensitive hydrogel for periodontal application. J Mater Res. 2022;37(18):3021-3032. doi: 10.1557/s43578-022-00706-y
- Ma S, Lu X, Yu X, et al. An injectable multifunctional thermo-sensitive chitosan-based hydrogel for periodontitis therapy. Biomater Adv. 2022;142:213158. doi: 10.1016/j.bioadv.2022.213158
- Irvine SA, Venkatraman SS. Bioprinting and differentiation of stem cells. Molecules. 2016;21(9):1188. doi: 10.3390/molecules21091188
- Son C, Choi MS, Park JC. Different responsiveness of alveolar bone and long bone to epithelial mesenchymal interaction related factor. JBMR Plus. 2020;4(8):e10382. doi: 10.1002/jbm4.10382
- Liu X, Zhao K, Gong T, et al. Delivery of growth factors using a smart porous nanocomposite scaffold to repair a mandibular bone defect. Biomacromolecules. 2014;15(3):1019-1030. doi: 10.1021/bm401911p
- Mohd N, Razali M, Ghazali MJ, Abu Kasim NH. Current advances of three-dimensional bioprinting application in dentistry: a scoping review. Materials. 2022;15(18):6398. doi: 10.3390/ma15186398
- Filipowska J, Tomaszewski KA, Niedźwiedzki Ł, Walocha JA, Niedźwiedzki T. The role of vasculature in bone development, regeneration and proper systemic functioning. Angiogenesis. 2017;20(3):291-302. doi: 10.1007/s10456-017-9541-1
- Qu H, Fu H, Han Z, Sun Y. Biomaterials for bone tissue engineering scaffolds: a review. RSC Adv. 2019;9(45):26252-26262. doi: 10.1039/C9RA05214C
- Burdis R, Kelly DJ. Biofabrication and bioprinting using cellular aggregates, microtissues and organoids for the engineering of musculoskeletal tissues. Acta Biomater. 2021;126:1-14. doi: 10.1016/j.actbio.2021.03.016
- Miao Y, Chen Y, Luo J, et al. Black phosphorus nanosheets-enabled DNA hydrogel integrating 3D-printed scaffold for promoting vascularized bone regeneration. Bioact Mater. 2023;21:97-109. doi: 10.1016/j.bioactmat.2022.08.005
- Zhao X, Xu Z, Xiao L, et al. Review on the vascularization of organoids and organoids-on-a-chip. Front Bioeng Biotechnol. 2021;9:637048. doi: 10.3389/fbioe.2021.637048
- Miri AK, Khalilpour A, Cecen B, Maharjan S, Shin SR, Khademhosseini A. Multiscale bioprinting of vascularized models. Biomaterials. 2019;198:204-216. doi: 10.1016/j.biomaterials.2018.08.006
- Dong Y, Wang S, Ke Y, et al. 4D printed hydrogels: fabrication, materials, and applications. Adv Mater Technol. 2020;5(6):2000034. doi: 10.1002/admt.202000034
- Champeau M, Heinze DA, Viana TN, de Souza ER, Chinellato AC, Titotto S. 4D printing of hydrogels: a review. Adv Funct Mater. 2020;30(31):1910606. doi: 10.1002/adfm.201910606
- Yang X, Ma Y, Wang X, et al. A 3D-bioprinted functional module based on dec