3D-printed zinc/magnesium-doped hydroxyapatite-polycaprolactone composite scaffolds for angiogenesis and osteogenesis
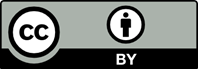
Critical-sized bone defect repair remains a major clinical challenge that requires scaffolds with angiogenesis and osteogenesis potential. Herein, we synthesized zinc (Zn)-doped and zinc/magnesium (Zn/Mg)-co-doped hydroxyapatite (HA) via the hydrothermal method and subsequently mixed them with polycaprolactone (PCL) as ink to fabricate composite scaffolds through 3D printing. We explored the potential of composite scaffolds in promoting angiogenesis and osteogenesis. In vitro experiments demonstrated that Zn/Mg-co-doped composite scaffolds can promote angiogenesis. In addition, Zn/Mg-co-doped scaffolds could promote osteogenesis and were superior to Zn-doped composite scaffolds. Furthermore, in vivo studies using a rat femoral defect model confirmed that the Zn/Mg-co-doped scaffolds repaired bone defects. Thus, the Zn/Mg-co-doped composite scaffolds developed in this study were effective in promoting angiogenesis and bone defect repairs, providing an excellent solution for the design and development of clinical materials.

- Li Y, Xu JK, Mi J, et al. Biodegradable magnesium combined with distraction osteogenesis synergistically stimulates bone tissue regeneration via CGRP-FAK-VEGF signaling axis. Biomaterials. 2021;275:120984. doi: 10.1016/j.biomaterials.2021.120984
- Wang Q, Xia QQ, Wu Y, et al. 3D-printed atsttrin-incorporated alginate/hydroxyapatite scaffold promotes bone defect regeneration with TNF/TNFR signaling involvement. Adv Healthc Mater. 2015;4:1701-1708. doi: 10.1002/adhm.201500211
- Hasani-Sadrabadi MM, Sarrion P, Pouraghaei S, et al. An engineered cell-laden adhesive hydrogel promotes craniofacial bone tissue regeneration in rats. Sci Transl Med. 2020;12:eaay6853. doi: 10.1126/scitranslmed.aay6853
- Possolli NM, Raupp-Pereira F, Montedo ORK, et al. LZS bioactive glass-ceramic scaffolds: colloidal processing, foam replication technique and mechanical properties to bone tissue engineering. Open Ceram. 2022;9:100219. doi: 10.1016/j.oceram.2022.100219
- Ren Y, Kong WQ, Liu YH, et al. Photocurable 3D-printed PMBG/TCP scaffold coordinated with PTH (1-34) bidirectionally regulates bone homeostasis to accelerate bone regeneration. Adv Healthc Mater. 2023;12:e202300292. doi: 10.1002/adhm.202300292
- Wu YG, Xing ZY, Zhao R, et al. Engineered cell-laden armor unit-mimicking bioceramic granules for bone regeneration. Adv Funct Mater. 2024;34:10331. doi: 10.1002/adfm.202310331
- Zhao R, Chen SY, Zhao WL, et al. A bioceramic scaffold composed of strontium-doped three-dimensional hydroxyapatite whiskers for enhanced bone regeneration in osteoporotic defects. Theranostics. 2020;10: 1572-1589. doi: 10.7150/thno.40103
- Xu JK, Hu PJ, Zhang XT, et al. Magnesium implantation or supplementation ameliorates bone disorder in CFTR-mutant mice through an ATF4-dependent Wnt/β-catenin signaling. Bioact Mater. 2022;8:95-108. doi: 10.1016/j.bioactmat.2021.06.034
- Li WT, Miao WQ, Liu YH, et al. Bioprinted constructs that mimic the ossification center microenvironment for targeted innervation in bone regeneration. Adv Funct Mater. 2022;32:2109871. doi: 10.1002/adfm.202109871
- Gu JN, Zhang QQ, Geng MR, et al. Construction of nanofibrous scaffolds with interconnected perfusable microchannel networks for engineering of vascularized bone tissue. Bioact Mater. 2021;6:3254-3268. doi: 10.1016/j.bioactmat.2021.02.033
- Dorozhkin SV. Multiphasic calcium orthophosphate (CaPO) bioceramics and their biomedical applications. Ceram Int. 2016;42:6529-6554. doi: 10.1016/j.ceramint.2016.01.062
- Thompson JB, Kindt JH, Drake B, et al. Bone indentation recovery time correlates with bond reforming time. Nature. 2001;414:773-776. doi: 10.1038/414773a
- Lim KT, Patel DK, Dutta SD, et al. Human teeth-derived bioceramics for improved bone regeneration. Nanomaterials Basel. 2020;10:2396. doi: 10.3390/nano10122396
- Zhang H, Huang HF, Hao GR, et al. 3D printing hydrogel scaffolds with nanohydroxyapatite gradient to effectively repair osteochondral defects in rats. Adv Funct Mater. 2021;31:2006697. doi: 10.1002/adfm.202006697
- Huang H, Yang AC, Li JS, et al. Preparation of multigradient hydroxyapatite scaffolds and evaluation of their osteoinduction properties. Regen Biomater. 2022;9:001. doi: 10.1093/rb/rbac001
- Supova M. Substituted hydroxyapatites for biomedical applications: a review. Ceram Int. 2015;41:9203-9231. doi: 10.1016/j.ceramint.2015.03.316
- Lakhkar NJ, Lee IH, Kim HW, et al. Bone formation controlled by biologically relevant inorganic ions: role and controlled delivery from phosphate-based glasses. Adv Drug Deliver Rev. 2013;65:405-420. doi: 10.1016/j.addr.2012.05.015
- Li SJ, Zhang LY, Liu CY, et al. Spontaneous immuno-modulation and regulation of angiogenesis and osteogenesis by Sr/Cu-borosilicate glass (BSG) bone cement to repair critical bone defects. Bioact Mater. 2023;23:101-117. doi: 10.1016/j.bioactmat.2022.10.021
- D’Mello S, Elangovan S, Hong L, et al. Incorporation of copper into chitosan scaffolds promotes bone regeneration in rat calvarial defects. J Biomed Mater Res B. 2015;103: 1044-1049. doi: 10.1002/jbm.b.33290
- Wang Y, Wang XY, Pang YY, et al. Ion-engineered microcryogels via osteogenesis-angiogenesis coupling and inflammation reversing augment vascularized bone regeneration. Adv Funct Mater. 2024;34(34):2400745. doi: 10.1002/adfm.202400745
- Ghorbani FM, Kaffashi B, Shokrollahi P, et al. PCL/ chitosan/Zn-doped nHA electrospun nanocomposite scaffold promotes adipose derived stem cells adhesion and proliferation. Carbohyd Polym. 2015;118:133-142. doi: 10.1016/j.carbpol.2014.10.071
- Kulanthaivel S, Mishra U, Agarwal T, et al. Improving the osteogenic and angiogenic properties of synthetic hydroxyapatite by dual doping of bivalent cobalt and magnesium ion. Ceram Int. 2015;41:11323-11333. doi: 10.1016/j.ceramint.2015.05.090
- Xiao DQ, Yang F, Zhao Q, et al. Fabrication of a Cu/Zn co-incorporated calcium phosphate scaffold-derived GDF-5 sustained release system with enhanced angiogenesis and osteogenesis properties. RSC Adv. 2018;8:29526-29534. doi: 10.1039/c8ra05441j
- Elrayah A, Zhi W, Feng S, et al. Preparation of micro/nano-structure copper-substituted hydroxyapatite scaffolds with improved angiogenesis capacity for bone regeneration. Materials. 2018;11:1516. doi: 10.3390/ma11091516
- Shoaib M, Bahadur A, Iqbal S, et al. Magnesium doped mesoporous bioactive glass nanoparticles: a promising material for apatite formation and mitomycin c delivery to the MG-63 cancer cells. J Alloy Compd. 2021;866:159013. doi: 10.1016/j.jallcom.2021.159013
- Castiglioni S, Cazzaniga A, Albisetti W, et al. Magnesium and osteoporosis: current state of knowledge and future research directions. Nutrients. 2013;5:3022-3033. doi: 10.3390/nu5083022
- Rude RK, Singer FR, and Gruber HE. Skeletal and hormonal effects of magnesium deficiency. J Am Coll Nutr. 2009;28:131-141. doi: 10.1080/07315724.2009.10719764
- Shrestha S, Lee SY, Shrestha D, et al. Micro/nanometer-sized porous structure of zinc phosphate incorporated Ti (HPO4) hydrate bioceramic induces osteogenic gene expression and enhances osteoporotic bone regeneration. Chem Eng J. 2022;450:138360. doi: 10.1016/j.cej.2022.138360
- Yin S, Lin SH, Xu JY, et al. Dominoes with interlocking consequences triggered by zinc: involvement of microelement-stimulated MSC-derived exosomes in senile osteogenesis and osteoclast dialogue. J Nanobiotechnol. 2023;21:346. doi: 10.1186/s12951-023-02085-w
- Shahed CA, Ahmad F, Günister E, et al. Antibacterial mechanism with consequent cytotoxicity of different reinforcements in biodegradable magnesium and zinc alloys: a review. J Magnes Alloy. 2023;11:3038-3058. doi: 10.1016/j.jma.2023.08.018
- Matsunaga K. First-principles study of substitutional magnesium and zinc in hydroxyapatite and octacalcium phosphate. J Chem Phys. 2008;128:245101. doi: 10.1063/1.2940337
- Naing MW, Chua CK, Leong KF, et al. Fabrication of customised scaffolds using computer-aided design and rapid prototyping techniques. Rapid Prototyping J. 2005;11(4):249-259. doi: 10.1108/13552540510612938
- Chua CK, Leong KF, Cheah CM, et al. Development of a tissue engineering scaffold structure library for rapid prototyping. part 1: investigation and classification. Int J Adv Manuf Technol. 2003;21:291-301. doi: 10.1007/s001700300034
- Chua CK, Leong KF, Cheah CM, et al. Development of a tissue engineering scaffold structure library for rapid prototyping. Part 2: Parametric library and assembly program. Int J Adv Manuf Technol. 2003;21:302-312. doi: 10.1007/s001700300035
- Dong C, Wei H, Zhang XN, et al. 3D printed hydrogel/ wesselsite-PCL composite scaffold with structural change from core/shell fibers to microchannels for enhanced bone regeneration. Compos B: Eng. 2022;246:110264. doi: 10.1016/j.compositesb.2022.110264
- Xiao DQ, Tan Z, Fu YK, et al. Hydrothermal synthesis of hollow hydroxyapatite microspheres with nano-structured surface assisted by inositol hexakisphosphate. Ceram Int. 2014;40:10183-10188. doi: 10.1016/j.ceramint.2014.02.057
- Huang H, Qiang L, Fan MJ, et al. 3D-printed tri-element-doped hydroxyapatite/ polycaprolactone composite scaffolds with antibacterial potential for osteosarcoma therapy and bone regeneration. Bioact Mater. 2024;31:18-37. doi: 10.1016/j.bioactmat.2023.07.004
- Kim H, Mondal S, Bharathiraja S, et al. Optimized Zn-doped hydroxyapatite/doxorubicin bioceramics system for efficient drug delivery and tissue engineering application. Ceram Int. 2018;44:6062-6071. doi: 10.1016/j.ceramint.2017.12.235
- Fleet ME, Liu X. Coupled substitution of type A and B carbonate in sodium-bearing apatite. Biomaterials. 2007;28:916-926. doi: 10.1016/j.biomaterials.2006.11.003
- Karunakaran G, Kumar GS, Cho EB, et al. Microwave-assisted hydrothermal synthesis of mesoporous carbonated hydroxyapatite with tunable nanoscale characteristics for biomedical applications. Ceram Int. 2019;45:970-977. doi: 10.1016/j.ceramint.2018.09.273
- Kundu K, Afshar A, Katti DR, et al. Composite nanoclay-hydroxyapatite-polymer fiber scaffolds for bone tissue engineering manufactured using pressurized gyration. Compos Sci Technol. 2021;202:108598. doi: 10.1016/j.compscitech.2020.108598
- Karageorgiou V, Kaplan D. Porosity of 3D biomaterial scaffolds and osteogenesis. Biomaterials. 2005;26:5474-5491. doi: 10.1016/j.biomaterials.2005.02.002
- Zhao FL, Gao A, Liao Q, et al. Balancing the anti-bacterial and pro-osteogenic properties of Ti-based implants by partial conversion of ZNO nanorods into hybrid zinc phosphate nanostructures. Adv Funct Mater. 2024;34:2311812. doi: 10.1002/adfm.202311812
- Li Y, Xiong W, Zhang CC, et al. Enhanced osseointegration and antibacterial action of zinc-loaded titania-nanotube-coated titanium substrates: and studies. J Biomed Mater Res A. 2014;102:3939-3950. doi: 10.1002/jbm.a.35060
- Rasmussen JW, Martinez E, Louka P, et al. Zinc oxide nanoparticles for selective destruction of tumor cells and potential for drug delivery applications. Expert Opin Drug Del. 2010;7:1063-1077. doi: 10.1517/17425247.2010.502560
- Xu L, Willumeit-Römer R, Luthringer-Feyerabend BJC. Effect of magnesium-degradation products and hypoxia on the angiogenesis of human umbilical vein endothelial cells. Acta Biomater. 2019;98:269-283. doi: 10.1016/j.actbio.2019.02.018
- Hung CC, Chaya A, Liu K, et al. The role of magnesium ions in bone regeneration involves the canonical Wnt signaling pathway. Acta Biomater. 2019;98:246-255. doi: 10.1016/j.actbio.2019.06.001