Bioprinting of wearable sensors, brain-machine interfaces, and exoskeleton robots
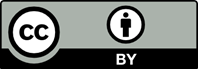
Bioprinting holds the promise of producing biocompatible structures capable of seamlessly integrating with human physiology, improving human health by enabling the precise fabrication of tissue models that closely mimic the architecture and functions of human skin, brain, and bone. Building on the advancements of bioprinting, there has been a corresponding increase in cross-disciplinary innovations in wearable technologies, brain-machine interfaces, and exoskeleton robotics. Given the progress of bioprinting in skin study, wearable electronics are expected to have improved biocompatibility and integration with the human body. For patient-specific neural tissues created using bioprinting, the potential to replicate neural activities through the synergy of bioprinting and brain-machine interfaces presents opportunities to enhance the performance of more advanced neuromorphic systems. Inspired by the advancements of bioprinting in producing patient-specific bone grafts and scaffolds, this technology could bridge the gap between mechanical systems and biomechanics, redefining the limits of skeleton robotics. This review explores the advancements of bioprinting in wearable sensors, brain-machine interfaces, and exoskeleton robots, and briefly addresses the existing and potential challenges in interdisciplinary research.

- Murphy SV, Atala A. 3D bioprinting of tissues and organs. Nat Biotechnol. 2014;32(8):773-785. doi: 10.1038/nbt.2958
- Budharaju H, Sundaramurthi D, Sethuraman S. Embedded 3D bioprinting-an emerging strategy to fabricate biomimetic and large vascularized tissue constructs. Bioact Mater. 2024;32:356-384. doi: 10.1016/j.bioactmat.2023.10.012
- Vijayavenkataraman S, Yan W C, Lu W F, et al. 3D bioprinting of tissues and organs for regenerative medicine. Adv Drug Deliv Rev. 2018;132:296-332. doi: 10.1016/j.addr.2018.07.004
- Dornhof J, Zieger V, Kieninger J, et al. Bioprinting-based automated deposition of single cancer cell spheroids into oxygen sensor microelectrode wells. Lab Chip. 2022;22(22):4369-4381. doi: 10.1039/D2LC00705C
- Liu H, Xing F, Yu P, et al. A review of biomacromolecule-based 3D bioprinting strategies for structure-function integrated repair of skin tissues. Int J Biol Macromol. 2024;268:131623. doi: 10.1016/j.ijbiomac.2024.131623
- Abbadessa A, Ronca A, Salerno A. Integrating bioprinting, cell therapies and drug delivery towards in vivo regeneration of cartilage, bone and osteochondral tissue. Drug Deliv Transl Res. 2024;14:858-894. doi: 10.1007/s13346-023-01437-1
- Lee S J, Esworthy T, Stake S, et al. Advances in 3D bioprinting for neural tissue engineering. Adv Biosyst. 2018;2(4):1700213. doi: 10.1002/adbi.201700213
- Gravina R, Fortino G. Wearable body sensor networks: state-of-the-art and research directions. IEEE Sens J. 2020;21(11):12511-12522. doi: 10.1109/JSEN.2020.3044447
- Vasilopoulou M, Mohd Yusoff AR, Chai Y, et al. Neuromorphic computing based on halide perovskites. Nat Electron. 2023;6(12):949-962. doi: 10.1038/s41928-023-01082-z
- Spezialetti M, Placidi G, Rossi S. Emotion recognition for human-robot interaction: recent advances and future perspectives. Front Robot AI. 2020;7:532279. doi: 10.3389/frobt.2020.532279
- Ates HC, Nguyen PQ, Gonzalez-Macia L, et al. End-to-end design of wearable sensors. Nat Rev Mater. 2022; 7(11):887-907. doi: 10.1038/s41578-022-00460-x
- Liu F, Deswal S, Christou A, et al. Neuro-inspired electronic skin for robots. Sci Robot. 2022;7(67):eabl7344. doi: 10.1126/scirobotics.abl7344
- Li D, Yao K, Gao Z, et al. Recent progress of skin-integrated electronics for intelligent sensing. Light: Advanced Manufacturing. 2021;2(1):39-58. doi: 10.37188/lam.2021.004
- Shanechi MM. Brain–machine interfaces from motor to mood. Nat Neurosci. 2019;22(10):1554-1564. doi: 10.1038/s41593-019-0488-y
- Ziai Y, Zargarian SS, Rinoldi C, et al. Conducting polymer‐based nanostructured materials for brain–machine interfaces. Wiley Interdiscip Rev Nanomed Nanobiotechnol. 2023;15(5):e1895. doi: 10.1002/wnan.1895
- Portillo-Lara R, Goding JA, Green RA. Adaptive biomimicry: design of neural interfaces with enhanced biointegration. Curr Opin Biotechnol. 2021;72:62-68. doi: 10.1016/j.copbio.2021.10.004
- Shi D, Zhang W, Zhang W, et al. A review on lower limb rehabilitation exoskeleton robots. Chin J Mech Eng. 2019;32(1):1-11. doi: 10.1186/s10033-019-0389-8
- Selvam A, Aggarwal T, Mukherjee M, et al. Humans and robots: friends of the future? a bird’s eye view of biomanufacturing industry 5.0. Biotechnol Adv. 2023;68:108237. doi: 10.1016/j.biotechadv.2023.108237
- da Silva JLGF, Gonçalves SMB, da Silva HHP, et al. Three-dimensional printed exoskeletons and orthoses for the upper limb – a systematic review. Prosthet Orthot Int. 2022;10:1097. doi: 10.1097/PXR.0000000000000318
- Popov A, Malferrari S, Kalaskar DM. 3D bioprinting for musculoskeletal applications. J 3D Print Med. 2017;1(3): 191-211. doi: 10.2217/3dp-2017-0004
- Zhang YS, Haghiashtiani G, Hübscher T, et al. 3D extrusion bioprinting. Nat Rev Methods Primers. 2021;1(1):75. doi: 10.1038/s43586-021-00073-8
- Li X, Liu B, Pei B, et al. Inkjet bioprinting of biomaterials. Chem Rev. 2020;120(9):10793-10833. doi: 10.1021/acs.chemrev.0c00008
- Yang J, Chen Z, Gao C, et al. A mechanical-assisted post-bioprinting strategy for challenging bone defects repair. Nat Commun. 2024;15(1):3565. doi: 10.1038/s41467-024-48023-8
- Di Buduo CA, Lunghi M, Kuzmenko V, et al. Bioprinting soft 3D models of hematopoiesis using natural silk fibroin-based bioink efficiently supports platelet differentiation. Adv Sci. 2024;11:2308276. doi: 10.1002/advs.202308276
- Gillispie G, Prim P, Copus J, et al. Assessment methodologies for extrusion-based bioink printability. Biofabrication. 2020;12(2):022003. doi: 10.1088/1758-5090/ab6f0d
- Schwab A, Levato R, D’Este M, et al. Printability and shape fidelity of bioinks in 3D bioprinting. Chem Rev. 2020;120(19):11028-11055. doi: 10.1021/acs.chemrev.0c00084
- Cooke ME, Rosenzweig DH. The rheology of direct and suspended extrusion bioprinting. APL Bioengineering. 2021;5(1):011502. doi: 10.1063/5.0031475
- Gudapati H, Dey M, Ozbolat I. A comprehensive review on droplet-based bioprinting: past, present and future. Biomaterials. 2016;102:20-42. doi: 10.1016/j.biomaterials.2016.06.012
- Shah PP, Shah HB, Maniar KK, et al. Extrusion-based 3D bioprinting of alginate-based tissue constructs. Procedia CIRP. 2020;95:143-148. doi: 10.1016/j.procir.2020.06.007
- Kačarević ŽP, Rider PM, Alkildani S, et al. An introduction to 3D bioprinting: possibilities, challenges and future aspects. Materials. 2018;11(11):2199. doi: 10.3390/ma11112199
- Levato R, Dudaryeva O, Garciamendez-Mijares CE, et al. Light-based vat-polymerization bioprinting. Nat Rev Methods Primers. 2023;3(1):47. doi: 10.1038/s43586-023-00231-0
- Chang J, Sun X. Laser-induced forward transfer based laser bioprinting in biomedical applications. Front Bioeng Biotechnol. 2023;11:1255782. doi: 10.3389/fbioe.2023.1255782
- Wu Y, Su H, Li M, et al. Digital light processing‐based multi‐material bioprinting: processes, applications, and perspectives. J Biomed Mater Res A. 2023;111(4):527-542. doi: 10.1002/jbm.a.37473
- Afting C, Mainik P, Vazquez-Martel C, et al. Minimal-invasive 3D laser printing of microimplants in organismo. Adv Sci. 2024:2401110. doi: 10.1002/advs.202401110
- Zennifer A, Manivannan S, Sethuraman S, et al. 3D bioprinting and photocrosslinking: emerging strategies & future perspectives. Biomater Adv. 2022;134:112576. doi: 10.1016/j.msec.2021.112576
- Kam D, Rulf O, Reisinger A, et al. 3D printing by stereolithography using thermal initiators. Nat Commun. 2024;15(1):2285. doi: 10.1038/s41467-024-46532-0
- Thangadurai M, Ajith A, Budharaju H, et al. Advances in electrospinning and 3D bioprinting strategies to enhance functional regeneration of skeletal muscle tissue, Biomater Adv. 2022;142:213135. doi: 10.1016/j.bioadv.2022.213135
- Van de Walle A, Perez J E, Wilhelm C. Magnetic bioprinting of stem cell-based tissues. Bioprinting, 2023;30:e00265. doi: 10.1016/j.bprint.2023.e00265
- Rasouli R, Villegas KM, Tabrizian M. Acoustofluidics– changing paradigm in tissue engineering, therapeutics development, and biosensing. Lab Chip. 2023;23(5): 1300-1338. doi: 10.1039/D2LC00439A
- Raees S, Ullah F, Javed F, et al. Classification, processing, and applications of bioink and 3D bioprinting: a detailed review. Int J Biol Macromol. 2023;232:123476. doi: 10.1016/j.ijbiomac.2023.123476
- Wang H, Yu H, Zhou X, et al. An overview of extracellular matrix-based bioinks for 3D bioprinting. Front Bioeng Biotechnol. 2022;10:905438. doi: 10.3389/fbioe.2022.905438
- Ong CS, Yesantharao P, Huang CY, et al. 3D bioprinting using stem cells. Pediatr Res. 2018;83(1):223-231. doi: 10.1038/pr.2017.252
- Zhang H, Wang Y, Zheng Z, et al. Strategies for improving the 3D printability of decellularized extracellular matrix bioink. Theranostics. 2023;13(8):2562. doi: 10.7150/thno.81785
- Tang M, Rich JN, Chen S. Biomaterials and 3D bioprinting strategies to model glioblastoma and the blood-brain barrier. Adv. Mater. 2021;33(5):2004776. doi: 10.1002/adma.202004776
- Hull SM, Brunel LG, Heilshorn SC. 3D bioprinting of cell‐laden hydrogels for improved biological functionality. Adv. Mater. 2022;34(2):2103691. doi: 10.1002/adma.202103691
- Mancha Sánchez E, Gómez-Blanco JC, López Nieto E, et al. Hydrogels for bioprinting: a systematic review of hydrogels synthesis, bioprinting parameters, and bioprinted structures behavior. Front Bioeng Biotechnol. 2020;8:776. doi: 10.3389/fbioe.2020.00776
- Liu F, Wang X. Synthetic polymers for organ 3D printing. Polymers. 2020;12(8):1765. doi: 10.3390/polym12081765
- Muthukrishnan L. Imminent antimicrobial bioink deploying cellulose, alginate, EPS and synthetic polymers for 3D bioprinting of tissue constructs. Carbohydr Polym. 2021;260:117774. doi: 10.1016/j.carbpol.2021.117774
- Cai Y, Chang SY, Gan SW, et al. Nanocomposite bioinks for 3D bioprinting. Acta Biomater. 2022;151:45-69. doi: 10.1016/j.actbio.2022.08.014
- Loukelis K, Helal ZA, Mikos AG, et al. Nanocomposite bioprinting for tissue engineering applications. Gels. 2023;9(2):103. doi: 10.3390/gels9020103
- Dong H, Lin J, Tao Y, et al. AI-enhanced biomedical micro/nanorobots in microfluidics. Lab Chip. 2024;24: 1419-1440. doi: 10.1039/D3LC00909B
- Heid S, Boccaccini AR. Advancing bioinks for 3D bioprinting using reactive fillers: a review. Acta Biomater. 2020; 113:1-22. doi: 10.1016/j.actbio.2020.06.040
- Kolan KCR, Semon JA, Bindbeutel AT, et al. Bioprinting with bioactive glass loaded polylactic acid composite and human adipose stem cells. Bioprinting. 2020;18:e00075. doi: 10.1016/j.bprint.2020.e00075
- Sordi MB, Cruz A, Fredel MC, et al. Three-dimensional bioactive hydrogel-based scaffolds for bone regeneration in implant dentistry. Mater Sci Eng C Mater Biol Appl. 2021;124:112055. doi: 10.1016/j.msec.2021.112055
- Murphy SV, De Coppi P, Atala A. Opportunities and challenges of translational 3D bioprinting. Nat Biomed Eng. 2020;4(4):370-380. doi: 10.1038/s41551-019-0471-7
- Xu H, Zhang Y, Zhang Y, et al. 3D bioprinting advanced biomaterials for craniofacial and dental tissue engineering – a review. Materials & Design. 2024;241:112886. doi: 10.1016/j.matdes.2024.112886
- Khati, V, Ramachandraiah, H, et al. 3D Bioprinting of multi-material decellularized liver matrix hydrogel at physiological temperatures. Biosensors. 2022;12(7):521. doi: 10.3390/bios12070521
- Zhang Y, Enhejirigala, Yao B, et al. Using bioprinting and spheroid culture to create a skin model with sweat glands and hair follicles. Burns Trauma. 2021;9:tkab013. doi: 10.1093/burnst/tkab013
- O’Shea DG, Hodgkinson T, Curtin CM, et al. An injectable and 3D printable pro-chondrogenic hyaluronic acid and collagen type II composite hydrogel for the repair of articular cartilage defects. Biofabrication. 2023;16(1):015007. doi: 10.1088/1758-5090/ad047a
- Cruz EM, Machado LS, Zamproni LN, et al. A gelatin methacrylate-based hydrogel as a potential bioink for 3D bioprinting and neuronal differentiation. Pharmaceutics. 2023;15(2):627. doi: 10.3390/pharmaceutics15020627
- Xiong R, Zhang Z, Chai W, et al. Freeform drop-on-demand laser printing of 3D alginate and cellular constructs. Biofabrication. 2015;7:045011. doi: 10.1088/1758-5090/7/4/045011
- Xie M, Gao Q, Zhao H, et al. Electro‐assisted bioprinting of low‐concentration GelMA microdroplets. Small. 2019;15:1804216. doi: 10.1002/smll.201804216
- Puistola P, Miettinen S, Skottman H, et al. Novel strategy for multi-material 3D bioprinting of human stem cell based corneal stroma with heterogenous design. Mater Today Bio.2024;24:100924. doi: 10.1016/j.mtbio.2023.100924
- Mahdavi SS, Abdekhodaie MJ, Kumar H, et al. Stereolithography 3D bioprinting method for fabrication of human corneal stroma equivalent. Ann Biomed Eng. 2020;48:1955-1970. doi: 10.1007/s10439-020-02537-6
- Boularaoui S, Shanti A, Lanotte M, et al. Nanocomposite conductive bioinks based on low-concentration GelMA and MXene nanosheets/gold nanoparticles providing enhanced printability of functional skeletal muscle tissues. ACS Biomater Sci Eng. 2021;7(12):5810-5822. doi: 10.1021/acsbiomaterials.1c01193
- Lackner F, Šurina P, Fink J, et al. 4‐axis 3D‐printed tubular biomaterials imitating the anisotropic nanofiber orientation of porcine aortae. Adv Healthc Mater. 2024;13(2):2302348. doi: 10.1002/adhm.202302348
- Mousavi A, Hedayatnia A, van Vliet PP, et al. Development of photocrosslinkable bioinks with improved electromechanical properties for 3D bioprinting of cardiac BioRings. Applied Materials Today. 2024;36:102035. doi: 10.1016/j.apmt.2023.102035
- Wang Y, Yuan X, Yao B, et al. Tailoring bioinks of extrusion-based bioprinting for cutaneous wound healing. Bioact Mater. 2022;17:178-194. doi: 10.1016/j.bioactmat.2022.01.024
- Ozbek II, Saybasili H, Ulgen KO. Applications of 3D bioprinting technology to brain cells and brain tumor models: special emphasis to glioblastoma. ACS Biomater Sci Eng. 2024;10(5):2616-2635. doi: 10.1021/acsbiomaterials.3c01569
- Daly AC, Prendergast ME, Hughes AJ, et al. Bioprinting for the biologist. Cell. 2021;184:18-32. doi: 10.1016/j.cell.2020.12.002
- Ramesh S, Deep A, Tamayol A, et al. Advancing 3D bioprinting through machine learning and artificial intelligence. Bioprinting. 2024:e00331. doi: 10.1016/j.bprint.2024.e00331
- Manz A, Graber N, Widmer HM. Miniaturized total chemical analysis systems: a novel concept for chemical sensing. Sens Actuators B Chem. 1990;1(1-6):244-248. doi: 10.1016/0925-4005(90)80209-I
- Sun H, Jia Y, Dong H, et al. Combining additive manufacturing with microfluidics: an emerging method for developing novel organs-on-chips. Curr Opin Chem Eng. 2020;28:1-9. doi: 10.1016/j.coche.2019.10.006
- Yu S, Jing Y, Fan Y, et al. Ultrahigh efficient emulsification with drag-reducing liquid gating interfacial behavior. Proc Natl Acad Sci USA. 2022;119(29):e2206462119. doi: 10.1073/pnas.2206462119
- Cai B, Kilian D, Ramos Mejia D, et al. Diffusion‐based 3D bioprinting strategies. Adv Sci. 2024;11(8):2306470. doi: 10.1002/advs.202306470
- Sun H, Xiong L, Huang Y, et al. AI-aided on-chip nucleic acid assay for smart diagnosis of infectious disease. Fundam Res. 2022;2(3):476-486. doi: 10.1016/j.fmre.2021.12.005
- Sujigarasharma K, Sharulatha S, Lawanya Shri M, et al. Optimizing 3D bioprinting using advanced deep learning techniques a comparative study of CNN, RNN, and GAN. Comput Intell Bioprint. 2024;8: 157-173. doi: 10.1002/9781394204878.ch8
- Ramesh S, Deep A, Tamayol A, et al. Advancing 3D bioprinting through machine learning and artificial intelligence. Bioprinting. 2024;38:e00331. doi: 10.1016/j.bprint.2024.e00331
- Sun H, Xie W, Mo J, et al. Deep learning with microfluidics for on-chip droplet generation, control, and analysis. Front Bioeng Biotechnol. 2023;11:1208648. doi: 10.3389/fbioe.2023.1208648
- Liu C, Wang L, Lu W, et al. Computer vision-aided bioprinting for bone research. Bone Res. 2022;10(1):21. doi: 10.1038/s41413-022-00192-2
- Thai MT, Phan PT, Tran HA, et al. Advanced soft robotic system for in situ 3D bioprinting and endoscopic surgery. Adv Sci. 2023;10(12):2205656. doi: 10.1002/advs.202205656
- Wang Z, Xiao C, Roy M, et al. Bioinspired skin towards next-generation rehabilitation medicine. Front Bioeng Biotechnol. 2023;11:1196174. doi: 10.3389/fbioe.2023.1196174
- Motter Catarino C, Cigaran Schuck D, Dechiario L, et al. Incorporation of hair follicles in 3D bioprinted models of human skin. Sci Adv. 2023;9(41):eadg0297. doi: 10.1126/sciadv.adg0297
- Zhang B, Li J, Zhou J, et al. A three-dimensional liquid diode for soft, integrated permeable electronics. Nature. 2024;628:84-92. doi: 10.1038/s41586-024-07161-1
- Jorgensen AM, Gorkun A, Mahajan N, et al. Multicellular bioprinted skin facilitates human-like skin architecture in vivo. Sci Transl Med. 2023;15(716):eadf7547. doi: 10.1126/scitranslmed.adf7547
- Chortos A, Liu J, Bao Z. Pursuing prosthetic electronic skin. Nat Mater. 2016;15(9):937-950. doi: 10.1038/nmat4671
- Dong T, Hu J, Dong Y, et al. Advanced biomedical and electronic dual-function skin patch created through microfluidic-regulated 3D bioprinting. Bioact Mater. 2024;40:261-274. doi: 10.1016/j.bioactmat.2024.06.015
- Yang JC, Mun J, Kwon SY, et al. Electronic skin: recent progress and future prospects for skin‐attachable devices for health monitoring, robotics, and prosthetics. Adv. Mater. 2019;31:1904765. doi: 10.1002/adma.20190476
- Apelgren P, Amoroso M, Säljö K, et al. Long‐term in vivo integrity and safety of 3D‐bioprinted cartilaginous constructs. J Biomed Mater Res B Appl Biomater. 2021;109:126-136. doi: 10.1002/jbm.b.34687
- Agarwala S, Lee JM, Ng WL, et al. A novel 3D bioprinted flexible and biocompatible hydrogel bioelectronic platform. Biosens Bioelectron. 2018;102:365-371. doi: 10.1016/j.bios.2017.11.039
- Krishnadoss V, Kanjilal B, Hesketh A, et al. In situ 3D printing of implantable energy storage devices. Chem Eng J. 2021;409:128213. doi: 10.1016/j.cej.2020.128213
- Lei IM, Jiang C, Lei CL, et al. 3D printed biomimetic cochleae and machine learning co-modelling provides clinical informatics for cochlear implant patients. Nat Commun. 2021;12:6260. doi: 10.1038/s41467-021-26491-6
- Xu Y, Rothe R, Voigt D, et al. Convergent synthesis of diversified reversible network leads to liquid metal-containing conductive hydrogel adhesives. Nat Commun. 2021;12(1):2407. doi: 10.1038/s41467-021-22675-2
- Chatterjee B, Mohseni P, Sen S. Bioelectronic sensor nodes for the internet of bodies. Annu Rev Biomed Eng. 2023;25:101-129. doi: 10.1146/annurev-bioeng-110220-112448
- Kim Y, Alimperti S, Choi P, et al. An inkjet printed flexible electrocorticography (ECoG) microelectrode array on a thin parylene-C film. Sensors. 2022;22(3):1277. doi: 10.3390/s22031277
- Xie M, Shi Y, Zhang C, et al. In situ 3D bioprinting with bioconcrete bioink. Nat Commun. 2022;13(1):3597. doi: 10.1038/s41467-022-30997-y
- Sondell M, Lundborg G, Kanje M. Vascular endothelial growth factor has neurotrophic activity and stimulates axonal outgrowth, enhancing cell survival and Schwann cell proliferation in the peripheral nervous system. J Neurosci. 1999;19:5731-5740. doi: 10.1523/JNEUROSCI.19-14-05731.1999
- Carpanini SM, Torvell M, Morgan BP. Therapeutic inhibition of the complement system in diseases of the central nervous system. Front Immunol. 2019;10:362. doi: 10.3389/fimmu.2019.00362
- Hu Y, Wu Y, Gou Z, et al. 3D-engineering of cellularized conduits for peripheral nerve regeneration. Sci Rep. 2016;6(1):32184. doi: 10.1038/srep32184
- Hsu RS, Li SJ, Fang JH, et al. Wireless charging-mediated angiogenesis and nerve repair by adaptable microporous hydrogels from conductive building blocks. Nat Commun. 2022;13(1):5172. doi: 10.1038/s41467-022-32912-x
- Fang Y, Wang C, Liu Z, et al. 3D printed conductive multiscale nerve guidance conduit with hierarchical fibers for peripheral nerve regeneration. Adv Sci. 2023;10(12):2205744. doi: 10.1002/advs.202205744
- Courtine G, Micera S, DiGiovanna J, et al. Brain-machine interface: closer to therapeutic reality?. Lancet. 2013;381(9866):515-517. doi: 10.1016/S0140-6736(12)62164-3
- Zhang Q, Hu S, Talay R, et al. A prototype closed-loop brain–machine interface for the study and treatment of pain. Nat Biomed Eng. 2023;7(4):533-545. doi: 10.1038/s41551-021-00736-7
- Kantawala B, Hamitoglu AE, Nohra L, et al. Microengineered neuronal networks: enhancing brain-machine interfaces. Ann Med Surg. 2024;86(6):3535-3542. doi: 10.1097/MS9.0000000000002130
- Ghafoor U, Kim S, Hong KS. Selectivity and longevity of peripheral-nerve and machine interfaces: a review. Front Neurorobot. 2017;11:59. doi: 10.3389/fnbot.2017.00059
- Sun G, Zeng F, McCartin M, et al. Closed-loop stimulation using a multiregion brain-machine interface has analgesic effects in rodents. Sci Transl Med. 2022;14(651):eabm5868. doi: 10.1126/scitranslmed.abm5868
- Adewole DO, Struzyna LA, Burrell JC, et al. Development of optically controlled “living electrodes” with long-projecting axon tracts for a synaptic brain-machine interface. Sci Adv. 2021;7(4):eaay5347. doi: 10.1126/sciadv.aay5347
- Afanasenkau D, Kalinina D, Lyakhovetskii V, et al. Rapid prototyping of soft bioelectronic implants for use as neuromuscular interfaces. Nat Biomed Eng. 2020;4(10): 1010-1022. doi: 10.1038/s41551-020-00615-7
- Liu Y, Liu J, Chen S, et al. Soft and elastic hydrogel-based microelectronics for localized low-voltage neuromodulation. Nat Biomed Eng. 2019;3(1):58-68. doi: 10.1038/s41551-018-0335-6
- Roth JG, Brunel LG, Huang MS, et al. Spatially controlled construction of assembloids using bioprinting. Nat Commun. 2023;14(1):4346. doi: 10.1038/s41467-023-40006-5
- Ahmed T. Bio-inspired artificial synapses: neuromorphic computing chip engineering with soft biomaterials, memories-materials, devices. Circuits Syst. 2023;6:100088. doi: 10.1016/j.memori.2023.100088
- Cheng Z, Ríos C, Pernice WHP, et al. On-chip photonic synapse. Sci Adv. 2017;3(9):e1700160. doi: 10.1126/sciadv.1700160
- Fu T, Liu X, Fu S, et al. Self-sustained green neuromorphic interfaces. Nat Commun. 2021;12(1):3351. doi: 10.1038/s41467-021-23744-2
- Abdollahiyan P, Oroojalian F, Mokhtarzadeh A, et al. Hydrogel‐based 3D bioprinting for bone and cartilage tissue engineering. Biotechnol J. 2020;15(12):2000095. doi: 10.1002/biot.202000095
- Li T, Ma Z, Zhang Y, et al. Regeneration of humeral head using a 3D bioprinted anisotropic scaffold with dual modulation of endochondral ossification. Adv Sci. 2023;10(12): 2205059. doi: 10.1002/advs.202205059
- Liu Q, Dong X, Qi H, et al. 3D printable strong and tough composite organo-hydrogels inspired by natural hierarchical composite design principle. Nat Commun. 2024;1 5(1):3237. doi: 10.1038/s41467-024-47597-7
- Du L, Wu J, Han Y, et al. Immunomodulatory multicellular scaffolds for tendon-to-bone regeneration. Sci Adv. 2024;10(10):eadk6610. doi: 10.1126/sciadv.adk6610
- Jo HJ, Kang MS, Heo HJ, et al. Skeletal muscle regeneration with 3D bioprinted hyaluronate/gelatin hydrogels incorporating MXene nanoparticles. Int J Biol Macromol. 2024;265:130696. doi: 10.1016/j.ijbiomac.2024.130696
- Huan Y, Zhou D, Wu X, et al. 3D bioprinted autologous bone particle scaffolds for cranioplasty promote bone regeneration with both implanted and native BMSCs. Biofabrication. 2023;15(2):025016. doi: 10.1088/1758-5090/acbe21
- He H, Yuan Y, Wu Y, et al. Exoskeleton partial‐coated stem cells for infarcted myocardium restoring. Adv. Mater. 2023;35(52):2307169. doi: 10.1002/adma.202307169
- Zhao T, Zhou J, Wu W, et al. Antibacterial conductive polyacrylamide/quaternary ammonium chitosan hydrogel for electromagnetic interference shielding and strain sensing. Int J Biol Macromol. 2024;265:130795. doi: 10.1016/j.ijbiomac.2024.130795
- Wu J, Zhu Z, Pei X. 3D-printed biomimetic hydrogel for repairing tissue damage in motor systems. Chin J Tissue Eng Res. 2024;28:4703. doi: 10.12307/2024.535
- Xue Q, Ma L, Hu H, et al. 3D bioprinting as a prospective therapeutic strategy for corneal limbal epithelial stem cell deficiency. Int J Bioprint. 2023;9(3):710. doi: 10.18063/ijb.710
- Panagou S, Neumann WP, Fruggiero F. A scoping review of human robot interaction research towards Industry 5.0 human-centric workplaces. Int J Product Res. 2024;62(3): 974-990. doi: 10.1080/00207543.2023.2172473
- Dong H, Hu B, Zhang W, et al. Robotic-assisted automated in situ bioprinting. Int J Bioprint. 2023;9(1):629. doi: 10.18063/ijb.v9i1.629
- Guix M, Mestre R, Patiño T, et al. Biohybrid soft robots with self-stimulating skeletons. Sci Robot. 2021;6(53):eabe7577. doi: 10.1126/scirobotics.abe7577
- Mestre R, Patiño T, Sánchez S. Biohybrid robotics: from the nanoscale to the macroscale. Wiley Interdiscip Rev Nanomed Nanobiotechnol. 2021;13(5):e1703. doi: 10.1002/wnan.1703
- Jin D, Zhang L. Embodied intelligence weaves a better future. Nat Machine Intell. 2020;2(11):663-664. doi: 10.1038/s42256-020-00250-6
- Smirnova L, Caffo BS, Gracias DH, et al. Organoid intelligence (OI): the new frontier in biocomputing and intelligence-in-a-dish. Front Sci. 2023; 1:1017235. doi: 10.3389/fsci.2023.1017235
- Lee W, Xu C, Fu H, et al. 3D bioprinting highly elastic PEG‐PCL‐DA hydrogel for soft tissue fabrication and biomechanical stimulation. Adv Funct Mater. 2024;34:2313942. doi: 10.1002/adfm.202313942
- Kronenfeld JM, Rother L, Saccone MA, et al. Roll-to-roll, high-resolution 3D printing of shape-specific particles. Nature. 2024;627(8003):306-312. doi: 10.1038/s41586-024-07061-4