Challenges and perspectives of liver tissue engineering: From cell therapy to bioprinting
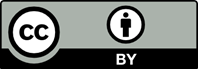
Liver tissue engineering offers a remarkable tool for toxicological screening and an approach to disease modeling, and provides an alternative solution to liver transplantation in treating end-stage liver diseases. As the liver is a highly specialized multicellular organ, distinctive features such as diversity of cell types, extracellular matrix composition, and three-dimensional architecture must be taken into consideration in recapitulating its physiology. Here, we review the current developments in liver tissue engineering approaches, focusing on their application to liver disease modeling and treatment, with special emphasis on bioprinting for high-throughput analysis. We also discuss the challenges encountered and the likely future developments in the area.
- Pimpin L, Cortez-Pinto H, Negro F, et al. Burden of liver disease in Europe: epidemiology and analysis of risk factors to identify prevention policies. J Hepatol. 2018;69(3):718-735. doi: 10.1016/j.jhep.2018.05.011
- Karlsen TH, Sheron N, Zelber-Sagi S, et al. The EASL– Lancet Liver Commission: protecting the next generation of Europeans against liver disease complications and premature mortality. Lancet. 2022;399(10319):61-116. doi: 10.1016/S0140-6736(21)01701-3
- Kaur S, Kidambi S, Ortega-Ribera M, et al. In vitro models for the study of liver biology and diseases: advances and limitations. CMGH. 2023;15(3):559-571. doi: 10.1016/j.jcmgh.2022.11.008
- Godoy P, Hewitt NJ, Albrecht U, et al. Recent advances in 2D and 3D in vitro systems using primary hepatocytes, alternative hepatocyte sources and non-parenchymal liver cells and their use in investigating mechanisms of hepatotoxicity, cell signaling and ADME. Arch Toxicol. 2013;87(8):1315-1530. doi: 10.1007/s00204-013-1078-5
- da Silva Morais A, Vieira S, Zhao X, et al. Advanced biomaterials and processing methods for liver regeneration: state‐of‐the‐art and future trends. Adv Healthc Mater. 2020;9(5). doi: 10.1002/adhm.201901435
- Ming Z, Tang X, Liu J, Ruan B. Advancements in research on constructing physiological and pathological liver models and their applications utilizing bioprinting technology. Molecules. 2023;28(9):3683. doi: 10.3390/molecules28093683
- Michalopoulos GK, DeFrances MC. Liver regeneration. Science. 1997;276(5309):60-66. doi: 10.1126/science.276.5309.60
- Kholodenko IV, Yarygin KN. Cellular mechanisms of liver regeneration and cell-based therapies of liver diseases. Biomed Res Int. 2017;2017:1-17. doi: 10.1155/2017/8910821
- Chawla S, Das A. Preclinical-to-clinical innovations in stem cell therapies for liver regeneration. Curr Res Transl Med. 2023;71(1):103365. doi: 10.1016/j.retram.2022.103365
- Asrani SK, Devarbhavi H, Eaton J, Kamath PS. Burden of liver diseases in the world. J Hepatol. 2019;70(1):151-171. doi: 10.1016/j.jhep.2018.09.014
- Burton R, Henn C, Lavoie D, et al. A rapid evidence review of the effectiveness and cost-effectiveness of alcohol control policies: an English perspective. Lancet. 2017;389(10078):1558-1580. doi: 10.1016/S0140-6736(16)32420-5
- Hart CL, Morrison DS, Batty GD, Mitchell RJ, Smith GD. Effect of body mass index and alcohol consumption onliver disease: analysis of data from two prospective cohort studies. BMJ. 2010;340(7747):634. doi: 10.1136/bmj.c1240
- Bataller R, Cabezas J, Aller R, et al. Enfermedad hepática por alcohol. Guías de práctica clínica. Documento de consenso auspiciado por la AEEH. Gastroenterol Hepatol. 2019;42(10):657-676. doi: 10.1016/j.gastrohep.2019.09.006
- Bhatia SN, Underhill GH, Zaret KS, Fox IJ. Cell and tissue engineering for liver disease. Sci Transl Med. 2014;6(245). doi: 10.1126/scitranslmed.3005975
- Pelechá M, Villanueva-Bádenas E, Timor-López E, Donato MT, Tolosa L. Cell models and omics techniques for the study of nonalcoholic fatty liver disease: focusing on stem cell-derived cell models. Antioxidants. 2021;11(1):86. doi: 10.3390/antiox11010086
- Ströbel S, Kostadinova R, Fiaschetti-Egli K, et al. A 3D primary human cell-based in vitro model of non-alcoholic steatohepatitis for efficacy testing of clinical drug candidates. Sci Rep. 2021;11(1):22765. doi: 10.1038/s41598-021-01951-7
- Tsuchida T, Friedman SL. Mechanisms of hepatic stellate cell activation. Nat Rev Gastroenterol Hepatol. 2017;14(7):397-411. doi: 10.1038/nrgastro.2017.38
- EASL. EASL-EASD-EASO Clinical Practice Guidelines for the management of non-alcoholic fatty liver disease. Obes Facts. 2016;9(2):65-90. doi: 10.1159/000443344
- Diehl AM, Day C. Cause, pathogenesis, and treatment of nonalcoholic steatohepatitis. N Engl J Med. 2017;377(21):2063-2072. doi: 10.1056/NEJMra1503519
- Arzumanyan A, Reis HMGPV, Feitelson MA. Pathogenic mechanisms in HBV- and HCV-associated hepatocellular carcinoma. Nat Rev Cancer. 2013;13(2):123-135. doi: 10.1038/nrc3449
- Ray G. Management of liver diseases: current perspectives. World J Gastroenterol. 2022;28(40):5818-5826. doi: 10.3748/wjg.v28.i40.5818
- Dhanasekaran R, Bandoh S, Roberts LR. Molecular pathogenesis of hepatocellular carcinoma and impact of therapeutic advances. F1000Res. 2016;5:879. doi: 10.12688/f1000research.6946.1
- Ruff SM, Manne A, Cloyd JM, Dillhoff M, Ejaz A, Pawlik TM. Current landscape of immune checkpoint inhibitor therapy for hepatocellular carcinoma. Curr Oncol. 2023;30(6): 5863-5875. doi: 10.3390/curroncol30060439
- Kaplowitz N. Idiosyncratic drug hepatotoxicity. Nat Rev Drug Discov. 2005;4(6):489-499. doi: 10.1038/nrd1750
- Björnsson ES, Gunnarsson BI, Gröndal G, et al. Risk of drug-induced liver injury from tumor necrosis factor antagonists. Clin Gastroenterol Hepatol. 2015;13(3):602-608. doi: 10.1016/j.cgh.2014.07.062
- EASL. EASL Clinical Practice Guidelines: drug-induced liver injury. J Hepatol. 2019;70(6):1222-1261. doi: 10.1016/j.jhep.2019.02.014
- Anand SK, Caputo M, Xia Y, et al. Inhibition of MAP4K4 signaling initiates metabolic reprogramming to protect hepatocytes from lipotoxic damage. J Lipid Res. 2022;63(7):100238. doi: 10.1016/j.jlr.2022.100238
- Pareja E, Gómez-Lechón MJ, Tolosa L. Induced pluripotent stem cells for the treatment of liver diseases: challenges and perspectives from a clinical viewpoint. Ann Transl Med. 2020;8(8):566-566. doi: 10.21037/atm.2020.02.164
- Adam R, Karam V, Delvart V, et al. Evolution of indications and results of liver transplantation in Europe. A report from the European Liver Transplant Registry (ELTR). J Hepatol. 2012;57(3):675-688. doi: 10.1016/j.jhep.2012.04.015
- Dutkowski P, Linecker M, DeOliveira ML, Müllhaupt B, Clavien PA. Challenges to liver transplantation and strategies to improve outcomes. Gastroenterology. 2015;148(2):307-323. doi: 10.1053/j.gastro.2014.08.045
- Bizzaro, Russo, Burra. New perspectives in liver transplantation: from regeneration to bioengineering. Bioengineering. 2019;6(3):81. doi: 10.3390/bioengineering6030081
- Smets F, Najimi M, Sokal EM. Cell transplantation in the treatment of liver diseases. Pediatr Transplant. 2008;12(1):6-13. doi: 10.1111/j.1399-3046.2007.00788.x
- Lysy PA, Najimi M, Stéphenne X, Bourgois A, Smets F, Sokal EM. Liver cell transplantation for Crigler-Najjar syndrome type I: update and perspectives. World J Gastroenterol. 2008;14(22):3464. doi: 10.3748/wjg.14.3464
- van de Kerkhove MP, Hoekstra R, Chamuleau RAFM, van Gulik TM. Clinical application of bioartificial liver support systems. Ann Surg. 2004;240(2):216-230. doi: 10.1097/01.sla.0000132986.75257.19
- Gebhardt R, Matz-Soja M. Liver zonation: novel aspects of its regulation and its impact on homeostasis. World J Gastroenterol. 2014;20(26):8491. doi: 10.3748/wjg.v20.i26.8491
- Panday R, Monckton CP, Khetani SR. The role of liver zonation in physiology, regeneration, and disease. Semin Liver Dis. 2022;42(01):001-016. doi: 10.1055/s-0041-1742279
- Trefts E, Gannon M, Wasserman DH. The liver. Curr Biol. 2017;27(21):R1147-R1151. doi: 10.1016/j.cub.2017.09.019
- Schulze RJ, Schott MB, Casey CA, Tuma PL, McNiven MA. The cell biology of the hepatocyte: a membrane trafficking machine. J Cell Biol. 2019;218(7):2096-2112. doi: 10.1083/jcb.201903090
- Ramachandran P, Matchett KP, Dobie R, Wilson-Kanamori JR, Henderson NC. Single-cell technologies in hepatology: new insights into liver biology and disease pathogenesis. Nat Rev Gastroenterol Hepatol. 2020;17(8):457-472. doi: 10.1038/s41575-020-0304-x
- Aizarani N, Saviano A, Sagar, et al. A human liver cell atlas reveals heterogeneity and epithelial progenitors. Nature. 2019;572(7768):199-204. doi: 10.1038/s41586-019-1373-2
- Dianat N, Dubois-Pot-Schneider H, Steichen C, et al. Generation of functional cholangiocyte-like cells from human pluripotent stem cells and HepaRG cells. Hepatology. 2014;60(2):700-714. doi: 10.1002/hep.27165
- Salas-Silva S, Simoni-Nieves A, Chávez-Rodríguez L, Gutiérrez-Ruiz MC, Bucio L, Quiroz LEG. Mechanism of cholangiocellular damage and repair during cholestasis. Ann Hepatol. 2021;26:100530. doi: 10.1016/j.aohep.2021.100530
- Dixon LJ, Barnes M, Tang H, Pritchard MT, Nagy LE. Kupffer cells in the liver. Compr Physiol. 2013;3(2):785-797. doi: 10.1002/cphy.c120026
- Han J, Ulevitch RJ. Limiting inflammatory responses during activation of innate immunity. Nat Immunol. 2005;6(12):1198-1205. doi: 10.1038/ni1274
- Sørensen KK, Simon‐Santamaria J, McCuskey RS, Smedsrød B. Liver sinusoidal endothelial cells. Compr Physiol. 2015;5:1751-1774. doi: 10.1002/cphy.c140078
- Jenne CN, Kubes P. Immune surveillance by the liver. Nat Immunol. 2013;14(10):996-1006. doi: 10.1038/ni.2691
- Ding B Sen, Cao Z, Lis R, et al. Divergent angiocrine signals from vascular niche balance liver regeneration and fibrosis. Nature. 2014;505(7481):97-102. doi: 10.1038/nature12681
- Halpern KB, Shenhav R, Massalha H, et al. Paired-cell sequencing enables spatial gene expression mapping of liver endothelial cells. Nat Biotechnol. 2018;36(10):962. doi: 10.1038/nbt.4231
- Senoo H. Structure and function of hepatic stellate cells. Med Electron Microsc. 2004;37(1):3-15. doi: 10.1007/s00795-003-0230-3
- Sanz-García C, Fernández-Iglesias A, Gracia-Sancho J, Arráez- Aybar LA, Nevzorova YA, Cubero FJ. The space of Disse: the liver hub in health and disease. Livers. 2021;1(1):3-26. doi: 10.3390/livers1010002
- Puche JE, Saiman Y, Friedman SL. Hepatic stellate cells and liver fibrosis. Compr Physiol. 2013;3:1473-1492. doi: 10.1002/cphy.c120035
- Frantz C, Stewart KM, Weaver VM. The extracellular matrix at a glance. J Cell Sci. 2010;123(24):4195-4200. doi: 10.1242/jcs.023820
- Baiocchini A, Montaldo C, Conigliaro A, et al. Extracellular matrix molecular remodeling in human liver fibrosis evolution. PLoS One. 2016;11(3). doi: 10.1371/journal.pone.0151736
- Bedossa P, Paradis V. Liver extracellular matrix in health and disease. J Pathol. 2003;200(4):504-515. doi: 10.1002/path.1397
- Allu I, Sahi AK, Koppadi M, Gundu S, Sionkowska A. Decellularization techniques for tissue engineering: towards replicating native extracellular matrix architecture in liver regeneration. J Funct Biomater. 2023;14(10):518. doi: 10.3390/jfb14100518
- Ford AJ, Rajagopalan P. Extracellular matrix remodeling in 3D: implications in tissue homeostasis and disease progression. Wiley Interdiscip Rev Nanomed Nanobiotechnol. 2018;10(4). doi: 10.1002/wnan.1503
- Mejias M, Gallego J, Naranjo-Suarez S, et al. CPEB4 increases expression of PFKFB3 to induce glycolysis and activate mouse and human hepatic stellate cells, promoting liver fibrosis. Gastroenterology. 2020;159(1):273-288. doi: 10.1053/j.gastro.2020.03.008
- Arriazu E, Ruiz de Galarreta M, Cubero FJ, et al. Extracellular matrix and liver disease. Antioxid Redox Signal. 2014;21(7):1078-1097. doi: 10.1089/ars.2013.5697
- Leroy V, Monier F, Bottari S, et al. Circulating matrix metalloproteinases 1, 2, 9 and their inhibitors TIMP-1 and TIMP-2 as serum markers of liver fibrosis in patients with chronic hepatitis C: comparison with PIIINP and hyaluronic acid. Am J Gastroenterol. 2004;99(2):271-279. doi: 10.1111/j.1572-0241.2004.04055.x
- Ramachandran P, Dobie R, Wilson-Kanamori JR, et al. Resolving the fibrotic niche of human liver cirrhosis at single-cell level. Nature. 2019;575(7783):512-518. doi: 10.1038/s41586-019-1631-3
- Yuan J, Li X, Yu S. Cancer organoid co-culture model system: novel approach to guide precision medicine. Front Immunol. 2023;13. doi: 10.3389/fimmu.2022.1061388
- Soldatow VY, LeCluyse EL, Griffith LG, Rusyn I. In vitro models for liver toxicity testing. Toxicol Res. 2013;2(1):23-39. doi: 10.1039/C2TX20051A
- Gomez-Lechon M, Donato M, Lahoz A, Castell J. Cell lines: a tool for in vitro drug metabolism studies. Curr Drug Metab. 2008;9(1):1-11. doi: 10.2174/138920008783331086
- Hewitt NJ, Gómez Lechón MJ, Houston JB, et al. Primary hepatocytes: current understanding of the regulation of metabolic enzymes and transporter proteins, and pharmaceutical practice for the use of hepatocytes in metabolism, enzyme induction, transporter, clearance, and hepatotoxicity studies. Drug Metab Rev. 2007;39(1):159-234. doi: 10.1080/03602530601093489
- O´Brien PJ, Chan K, Silber PM. Human and animal hepatocytes in vitro with extrapolation in vivo. Chem Biol Interact. 2004;150(1):97-114. doi: 10.1016/j.cbi.2004.09.003
- O´Brien PJ, Irwin W, Diaz D, et al. High concordance of drug-induced human hepatotoxicity with in vitro cytotoxicity measured in a novel cell-based model using high content screening. Arch Toxicol. 2006;80(9):580-604. doi: 10.1007/s00204-006-0091-3
- Tolosa L, Pareja-Ibars E, Donato MT, et al. Neonatal livers: a source for the isolation of good-performing hepatocytes for cell transplantation. Cell Transplant. 2014;23(10):1229-1242. doi: 10.3727/096368913X669743
- Gomez-Lechon M, Donato M, Castell J, Jover R. Human hepatocytes in primary culture: the choice to investigate drug metabolism in man. Curr Drug Metab. 2004;5(5):443-462. doi: 10.2174/1389200043335414
- Chowdhary V, Teng K yu, Thakral S, et al. miRNA-122 protects mice and human hepatocytes from acetaminophen toxicity by regulating cytochrome P450 family 1 subfamily A member 2 and family 2 subfamily e member 1 expression. Am J Pathol. 2017;187(12):2758-2774. doi: 10.1016/j.ajpath.2017.08.026
- Fox IJ, Chowdhury JR. Hepatocyte transplantation. Am J Transplant. 2004;4:7-13. doi: 10.1111/j.1600-6135.2004.0340.x
- Hansel MC, Davila JC, Vosough M, et al. The use of induced pluripotent stem cells for the study and treatment of liver diseases. Curr Protoc Toxicol. 2016;67(1). doi: 10.1002/0471140856.tx1413s67
- Iansante V, Mitry RR, Filippi C, Fitzpatrick E, Dhawan A. Human hepatocyte transplantation for liver disease: current status and future perspectives. Pediatr Res. 2018;83(1- 2):232-240. doi: 10.1038/pr.2017.284
- Gómez-Lechón MJ, Tolosa L, Conde I, Donato MT. Competency of different cell models to predict human hepatotoxic drugs. Expert Opin Drug Metab Toxicol. 2014;10(11):1553-1568. doi: 10.1517/17425255.2014.967680
- Donato MT, Tolosa L, Gómez-Lechón MJ. Culture and functional characterization of human hepatoma HepG2 cells. Methods Mol Biol. 2015;1250:77-93. doi: 10.1007/978-1-4939-2074-7_5
- Forbes SJ, Gupta S, Dhawan A. Cell therapy for liver disease: from liver transplantation to cell factory. J Hepatol. 2015;62(1):S157-S169. doi: 10.1016/j.jhep.2015.02.040
- Donato MT, Tolosa L. Stem-cell derived hepatocyte-like cells for the assessment of drug-induced liver injury. Differentiation. 2019;106(January):15-22. doi: 10.1016/j.diff.2019.02.004
- Hannan NRF, Segeritz CP, Touboul T, Vallier L. Production of hepatocyte-like cells from human pluripotent stem cells. Nat Protoc. 2013;8(2):430-437. doi: 10.1038/nprot.2012.153
- Takayama K, Morisaki Y, Kuno S, et al. Prediction of interindividual differences in hepatic functions and drug sensitivity by using human iPS-derived hepatocytes. Proc Natl Acad Sci. 2014;111(47): 16772-16777. doi: 10.1073/pnas.1413481111
- Bell CC, Dankers ACA, Lauschke VM, et al. Comparison of hepatic 2D sandwich cultures and 3d spheroids for long-term toxicity applications: a multicenter study. Toxicol Sci. 2018;162(2):655-666. doi: 10.1093/toxsci/kfx289
- Lee JY, Han HJ, Lee SJ, et al. Use of 3D human liver organoids to predict drug-induced phospholipidosis. Int J Mol Sci. 2020;21(8):2982. doi: 10.3390/ijms21082982
- Berger M, Neth O, Ilmer M, et al. Hepatoblastoma cells express truncated neurokinin-1 receptor and can be growth inhibited by aprepitant in vitro and in vivo. J Hepatol. 2014;60(5):985-994. doi: 10.1016/j.jhep.2013.12.024
- Davidson MD, Pickrell J, Khetani SR. Physiologically inspired culture medium prolongs the lifetime and insulin sensitivity of human hepatocytes in micropatterned co-cultures. Toxicology. 2021;449. doi: 10.1016/j.tox.2020.152662
- Takebe T, Sekine K, Enomura M, et al. Vascularized and functional human liver from an iPSC-derived organ bud transplant. Nature. 2013;499(7459):481-484. doi: 10.1038/nature12271
- Peters JT, Wechsler ME, Peppas NA. Advanced biomedical hydrogels: molecular architecture and its impact on medical applications. Regen Biomater. 2021;8(6). doi: 10.1093/rb/rbab060
- Annabi N, Tamayol A, Uquillas JA, et al. 25th anniversary article: rational design and applications of hydrogels inregenerative medicine. Adv Mater. 2014;26(1):85-124. doi: 10.1002/adma.201303233
- Jiang J, Tan Y, Liu A, et al. Tissue engineered artificial liver model based on viscoelastic hyaluronan-collagen hydrogel and the effect of EGCG intervention on ALD. Colloids Surf B Biointerfaces. 2021;206. doi: 10.1016/j.colsurfb.2021.111980
- Tong X, Zhao F, Ren Y, Zhang Y, Cui Y, Wang Q. Injectable hydrogels based on glycyrrhizin, alginate, and calcium for three‐dimensional cell culture in liver tissue engineering. J Biomed Mater Res A. 2018;106(12):3292-3302. doi: 10.1002/jbm.a.36528
- Lai JY. Biocompatibility of chemically cross-linked gelatin hydrogels for ophthalmic use. J Mater Sci Mater Med. 2010;21(6):1899-1911. doi: 10.1007/s10856-010-4035-3
- Tan H, Marra KG. Injectable, biodegradable hydrogels for tissue engineering applications. Materials. 2010;3(3): 1746-1767. doi: 10.3390/ma3031746
- Rodriguez-Fernandez J, Garcia-Legler E, Villanueva- Badenas E, et al. Primary human hepatocytes-laden scaffolds for the treatment of acute liver failure. Biomater Adv. 2023;153:213576. doi: 10.1016/j.bioadv.2023.213576
- Ramiah P, du Toit LC, Choonara YE, Kondiah PPD, Pillay V. Hydrogel-based bioinks for 3D bioprinting in tissue regeneration. Front Mater. 2020;7. doi: 10.3389/fmats.2020.00076
- Raghuwanshi VS, Garnier G. Characterisation of hydrogels: linking the nano to the microscale. Adv Colloid Interface Sci. 2019;274:102044. doi: 10.1016/j.cis.2019.102044
- Richbourg NR, Peppas NA. The swollen polymer network hypothesis: quantitative models of hydrogel swelling, stiffness, and solute transport. Prog Polym Sci. 2020;105:101243. doi: 10.1016/j.progpolymsci.2020.101243
- Willemse J, van Tienderen G, van Hengel E, et al. Hydrogels derived from decellularized liver tissue support the growth and differentiation of cholangiocyte organoids. Biomaterials. 2022;284:121473. doi: 10.1016/j.biomaterials.2022.121473
- Ye S, Boeter JWB, Penning LC, Spee B, Schneeberger K. Hydrogels for liver tissue engineering. Bioengineering. 2019;6(3):59. doi: 10.3390/bioengineering6030059
- Desimone MF, Hélary C, Rietveld IB, et al. Silica-collagen bionanocomposites as three-dimensional scaffolds for fibroblast immobilization. Acta Biomater. 2010;6(10): 3998-4004. doi: 10.1016/j.actbio.2010.05.014
- Lee JW, Choi YJ, Yong WJ, et al. Development of a 3D cell printed construct considering angiogenesis for liver tissue engineering. Biofabrication. 2016;8(1):015007. doi: 10.1088/1758-5090/8/1/015007
- Cui J, Wang H, Shi Q, Sun T, Huang Q, Fukuda T. Multicellular co-culture in three-dimensional gelatin methacryloyl hydrogels for liver tissue engineering. Molecules. 2019;24(9). doi: 10.3390/molecules24091762
- An S, Choi S, Min S, Cho SW. Hyaluronic acid-based biomimetic hydrogels for tissue engineering and medical applications. Biotechnol Bioprocess Eng. 2021;26(4):503-516. doi: 10.1007/s12257-020-0343-8
- Christoffersson J, Aronsson C, Jury M, Selegård R, Aili D, Mandenius CF. Fabrication of modular hyaluronan- PEG hydrogels to support 3D cultures of hepatocytes in a perfused liver-on-a-chip device. Biofabrication. 2019;11(1). doi: 10.1088/1758-5090/aaf657
- Kim M, Lee JY, Jones CN, Revzin A, Tae G. Heparin-based hydrogel as a matrix for encapsulation and cultivation of primary hepatocytes. Biomaterials. 2010;31(13):3596-3603. doi: 10.1016/j.biomaterials.2010.01.068
- Chen S, Liu A, Wu C, et al. Static–dynamic profited viscoelastic hydrogels for motor-clutch-regulated neurogenesis. ACS Appl Mater Interfaces. 2021;13(21):24463-24476. doi: 10.1021/acsami.1c03821
- Malinen MM, Kanninen LK, Corlu A, et al. Differentiation of liver progenitor cell line to functional organotypic cultures in 3D nanofibrillar cellulose and hyaluronan-gelatin hydrogels. Biomaterials. 2014;35(19):5110-5121. doi: 10.1016/j.biomaterials.2014.03.020
- Stevens KR, Miller JS, Blakely BL, Chen CS, Bhatia SN. Degradable hydrogels derived from PEG‐diacrylamide for hepatic tissue engineering. J Biomed Mater Res A. 2015;103(10):3331-3338. doi: 10.1002/jbm.a.35478
- Underhill GH, Chen AA, Albrecht DR, Bhatia SN. Assessment of hepatocellular function within PEG hydrogels. Biomaterials. 2007;28(2):256-270. doi: 10.1016/j.biomaterials.2006.08.043
- Lin TY, Ki CS, Lin CC. Manipulating hepatocellular carcinoma cell fate in orthogonally cross-linked hydrogels. Biomaterials. 2014;35(25):6898-6906. doi: 10.1016/j.biomaterials.2014.04.118
- Kim D, Kim M, Lee J, Jang J. Review on multicomponent hydrogel bioinks based on natural biomaterials for bioprinting 3D liver tissues. Front Bioeng Biotechnol. 2022;10. doi: 10.3389/fbioe.2022.764682
- Kim MK, Jeong W, Kang HW. Liver dECM–gelatin composite bioink for precise 3D printing of highly functional liver tissues. J Funct Biomater. 2023;14(8). doi: 10.3390/jfb14080417
- Liu H, Gong Y, Zhang K, et al. Recent advances in decellularized matrix-derived materials for bioink and 3D bioprinting. Gels. 2023;9(3). doi: 10.3390/gels9030195
- Monteiro MV, Henriques-Pereira M, Neves BM, Duarte ID, Gaspar VM, Mano JF. Photo-compartmentalized decellularized matrix-hyaluronan hybrid units for pancreatic tumor-stroma modeling. Adv Funct Mater. 2023;34(6):2305473. doi: 10.1002/adfm.202305473
- Agarwal T, Subramanian B, Maiti TK. Liver tissue engineering: challenges and opportunities. ACS Biomater Sci Eng. 2019;5(9):4167-4182. doi: 10.1021/acsbiomaterials.9b00745
- Huang D (Danielle), Gibeley SB, Xu C, et al. Engineering liver microtissues for disease modeling and regenerative medicine. Adv Funct Mater. 2020;30(44). doi: 10.1002/adfm.201909553
- Trujillo S, Dobre O, Dalby MJ. Salmeron-Sanchez M. Mechanotransduction and Growth Factor Signaling in Hydrogel-Based Microenvironments. Reis RL, eds. In Encyclopedia of Tissue Engineering and Regenerative Medicine. Oxford: Academic Press. 2019:87-101. doi: 10.1016/B978-0-12-801238-3.11141-9
- Cantini M, Donnelly H, Dalby MJ, Salmeron‐Sanchez M. The plot thickens: the emerging role of matrix viscosity in cell mechanotransduction. Adv Healthc Mater. 2020;9(8). doi: 10.1002/adhm.201901259
- Rizwan M, Ling C, Guo C, et al. Viscoelastic notch signaling hydrogel induces liver bile duct organoid growth and morphogenesis. Adv Healthc Mater. 2022;11(23). doi: 10.1002/adhm.202200880
- Bruns H, Kneser U, Holzhüter S, et al. Injectable liver: a novel approach using fibrin gel as a matrix for culture and intrahepatic transplantation of hepatocytes. Tissue Eng. 2005;11(11-12):1718-1726. doi: 10.1089/ten.2005.11.1718
- Le Guilcher C, Merlen G, Dellaquila A, et al. Engineered human liver based on pullulan-dextran hydrogel promotes mice survival after liver failure. Mater Today Bio. 2023;19:100554. doi: 10.1016/j.mtbio.2023.100554
- Meng D, Lei X, Li Y, Kong Y, Huang D, Zhang G. Three dimensional polyvinyl alcohol scaffolds modified with collagen for HepG2 cell culture. J Biomater Appl. 2020; 35(4-5):459-470. doi: 10.1177/0885328220933505
- He XL, Ge LL, Liu ZL, et al. Glycyrrhetinic acid-based thermoresponsive hydrogel as a synthetic extracellular matrix for hepatocyte culture and recovery. Ind Eng Chem Res. 2014;53(26):10618-10628. doi: 10.1021/ie404417u
- Ye S, Boeter JWB, Mihajlovic M, et al. A chemically defined hydrogel for human liver organoid culture. Adv Funct Mater. 2020;30(48). doi: 10.1002/adfm.202000893
- Ma L, Wu Y, Li Y, et al. Current advances on 3D‐bioprinted liver tissue models. Adv Healthc Mater. 2020;9(24). doi: 10.1002/adhm.202001517
- Naranjo-Alcazar R, Bendix S, Groth T, Gallego Ferrer G. Research progress in enzymatically cross-linked hydrogels as injectable systems for bioprinting and tissue engineering. Gels. 2023;9(3):230. doi: 10.3390/gels9030230
- Naghieh S, Chen X. Printability–a key issue in extrusion-based bioprinting. J Pharm Anal. 2021;11(5):564-579. doi: 10.1016/j.jpha.2021.02.001
- Li W, Liu Z, Tang F, et al. Application of 3D bioprinting in liver diseases. Micromachines. 2023;14(8). doi: 10.3390/mi14081648
- Wang Q, Liu J, Yin W, et al. Microscale tissue engineering of liver lobule models: advancements and applications. Front Bioeng Biotechnol. 2023;11. doi: 10.3389/fbioe.2023.1303053
- Xie M, Su J, Zhou S, Li J, Zhang K. Application of hydrogels as three-dimensional bioprinting ink for tissue engineering. Gels. 2023;9(2):88. doi: 10.3390/gels9020088
- Khati V, Ramachandraiah H, Pati F, Svahn HA, Gaudenzi G, Russom A. 3D bioprinting of multi-material decellularized liver matrix hydrogel at physiological temperatures. Biosensors. 2022;12(7):521. doi: 10.3390/bios12070521
- Unagolla JM, Jayasuriya AC. Hydrogel-based 3D bioprinting: a comprehensive review on cell-laden hydrogels, bioink formulations, and future perspectives. Appl Mater Today. 2020;18:100479. doi: 10.1016/j.apmt.2019.100479
- Gori M, Giannitelli SM, Torre M, et al. Biofabrication of hepatic constructs by 3D bioprinting of a cell-laden thermogel: an effective tool to assess drug-induced hepatotoxic response. Adv Healthc Mater. 2020; 9(21). doi: 10.1002/adhm.202001163
- Mazzocchi A, Devarasetty M, Huntwork R, Soker S, Skardal A. Optimization of collagen type I-hyaluronan hybrid bioink for 3D bioprinted liver microenvironments. Biofabrication. 2018;11(1):015003. doi: 10.1088/1758-5090/aae543
- He J, Wang J, Pang Y, et al. Bioprinting of a hepatic tissue model using humaninduced pluripotent stem cell-derived hepatocytes for drug-induced hepatotoxicity evaluation. Int J Bioprint. 2022;8(3):581. doi: 10.18063/ijb.v8i3.581
- Schmidt K, Berg J, Roehrs V, Kurreck J, Al-Zeer MA. 3D-bioprinted HepaRG cultures as a model for testing long term aflatoxin B1 toxicity in vitro. Toxicol Rep. 2020;7: 1578-1587. doi: 10.1016/j.toxrep.2020.11.003
- Bhise NS, Manoharan V, Massa S, et al. A liver-on-a-chip platform with bioprinted hepatic spheroids. Biofabrication. 2016;8(1). doi: 10.1088/1758-5090/8/1/014101
- Janani G, Priya S, Dey S, Mandal BB. Mimicking native liver lobule microarchitecture in vitro with parenchymal and non-parenchymal cells using 3D bioprinting for drug toxicity and drug screening applications. ACS Appl Mater Interfaces. 2022;14(8):10167-10186. doi: 10.1021/acsami.2c00312
- Xie F, Sun L, Pang Y, et al. Three-dimensional bio-printing of primary human hepatocellular carcinoma for personalized medicine. Biomaterials. 2021;265:120416. doi: 10.1016/j.biomaterials.2020.120416
- Ma X, Yu C, Wang P, et al. Rapid 3D bioprinting of decellularized extracellular matrix with regionally varied mechanical properties and biomimetic microarchitecture. Biomaterials. 2018;185:310-321. doi: 10.1016/j.biomaterials.2018.09.026
- Cuvellier M, Ezan F, Oliveira H, et al. 3D culture of HepaRG cells in GelMa and its application to bioprinting of a multicellular hepatic model. Biomaterials. 2021;269:120611. doi: 10.1016/j.biomaterials.2020.120611
- Kim D, Kim M, Lee J, Jang J. Review on multicomponent hydrogel bioinks based on natural biomaterials for bioprinting 3D liver tissues. Front Bioeng Biotechnol. 2022;10. doi: 10.3389/fbioe.2022.764682
- He J, Wang J, Pang Y, et al. Bioprinting of a hepatic tissue model using human-induced pluripotent stem cell-derived hepatocytes for drug-induced hepatotoxicity evaluation. Int J Bioprint. 2022;8(3):176-190. doi: 10.18063/ijb.v8i3.581
- Norona LM, Nguyen DG, Gerber DA, Presnell SC, LeCluyse EL. Editor’s highlight: modeling compound-induced fibrogenesis in vitro using three-dimensional bioprinted human liver tissues. Toxicol Sci. 2016;154(2):354-367. doi: 10.1093/toxsci/kfw169
- Norona LM, Nguyen DG, Gerber DA, Presnell SC, Mosedale M, Watkins PB. Bioprinted liver provides early insight into the role of Kupffer cells in TGF-β1 and methotrexate-induced fibrogenesis. PLoS One. 2019;14(1):e0208958. doi: 10.1371/journal.pone.0208958
- Maharjan S, Bonilla D, Sindurakar P, et al. 3D human nonalcoholic hepatic steatosis and fibrosis models. Biodes Manuf. 2021;4(2):157-170. doi: 10.1007/s42242-020-00121-4
- Mao S, He J, Zhao Y, et al. Bioprinting of patient-derived in vitro intrahepatic cholangiocarcinoma tumor model: establishment, evaluation and anti-cancer drug testing. Biofabrication. 2020;12(4). doi: 10.1088/1758-5090/aba0c3
- Kizawa H, Nagao E, Shimamura M, Zhang G, Torii H. Scaffold-free 3D bio-printed human liver tissue stably maintains metabolic functions useful for drug discovery. Biochem Biophys Rep. 2017;10:186-191. doi: 10.1016/j.bbrep.2017.04.004
- Dhawan A, Mitry RR, Hughes RD, et al. Hepatocyte transplantation for inherited factor VII deficiency. Transplantation. 2004;78(12):1812-1814. doi: 10.1097/01.TP.0000146386.77076.47
- Meyburg J, Das AM, Hoerster F, et al. One liver for four children: first clinical series of liver cell transplantation for severe neonatal urea cycle defects. Transplantation. 2009;87(5):636-641. doi: 10.1097/TP.0b013e318199936a
- Anderson TN, Zarrinpar A. Hepatocyte transplantation: past efforts, current technology, and future expansion of therapeutic potential. J Surg Res. 2018;226:48-55. doi: 10.1016/j.jss.2018.01.031
- Mazza G, Rombouts K, Rennie Hall A, et al. Decellularized human liver as a natural 3D-scaffold for liver bioengineering and transplantation. Sci Rep. 2015;5(1):13079. doi: 10.1038/srep13079
- Hammond JS, Beckingham IJ, Shakesheff KM. Scaffolds for liver tissue engineering. Expert Rev Med Devices. 2006;3(1):21-27. doi: 10.1586/17434440.3.1.21
- Jitraruch S, Dhawan A, Hughes RD, et al. Alginate microencapsulated hepatocytes optimised for transplantation in acute liver failure. PLoS One. 2014;9(12):1- 23. doi: 10.1371/journal.pone.0113609
- Parveen N, Khan AA, Baskar S, et al. Intraperitoneal transplantation of hepatocytes embedded in thermoreversible gelation polymer (Mebiol Gel) in acute liver failure rat model. J Hepatol. 2008;48:S71. doi: 10.1016/S0168-8278(08)60166-X
- Chiang CH, Wu WW, Li HY, et al. Enhanced antioxidant capacity of dental pulp-derived iPSC-differentiated hepatocytes and liver regeneration by injectable HGF-releasing hydrogel in fulminant hepatic failure. Cell Transplant. 2015;24(3):541-559. doi: 10.3727/096368915X686986
- Katsuda T, Teratani T, Ochiya T, Sakai Y. Transplantation of a fetal liver cell-loaded hyaluronic acid sponge onto the mesentery recovers a Wilson’s disease model rat. J Biochem. 2010;148(3):281-288. doi: 10.1093/jb/mvq063
- Zhang W, Du A, Liu S, Lv M, Chen S. Research progress in decellularized extracellular matrix-derived hydrogels. Regen Ther. 2021;18:88-96. doi: 10.1016/j.reth.2021.04.002
- Vishwakarma SK, Bardia A, Lakkireddy C, et al. Intraperitoneal transplantation of bioengineered humanized liver grafts supports failing liver in acute condition. Mater Sci Eng C. 2019;98:861-873. doi: 10.1016/j.msec.2019.01.045
- Higashi H, Yagi H, Kuroda K, et al. Transplantation of bioengineered liver capable of extended function in a preclinical liver failure model. Am J Transplant. 2022;22(3):731-744. doi: 10.1111/ajt.16928
- Tai BCU, Du C, Gao S, Wan ACA, Ying JY. The use of a polyelectrolyte fibrous scaffold to deliver differentiated hMSCs to the liver. Biomaterials. 2010;31(1):48-57. doi: 10.1016/j.biomaterials.2009.09.022
- Lin J, Meng L, Yao Z, et al. Use an alginate scaffold-bone marrow stromal cell (BMSC) complex for the treatment of acute liver failure in rats. Int J Clin Exp Med. 2015;8(8):12593- 12600.
- Zhang LK, Wang H, Yang R, et al. Bone marrow stem cells combined with polycaprolactone-polylactic acid-polypropylene amine scaffolds for the treatment of acute liver failure. Chem Eng J. 2019;360:1564-1576. doi: 10.1016/j.cej.2018.10.230
- Dhawan A, Chaijitraruch N, Fitzpatrick E, et al. Alginate microencapsulated human hepatocytes for the treatment of acute liver failure in children. J Hepatol. 2020;72(5): 877-884. doi: 10.1016/j.jhep.2019.12.002
- McKiernan PJ, Squires RH. Bridging transplantation with beads in paediatric acute liver failure. Nat Rev Gastroenterol Hepatol. 2020;17(4):197-198. doi: 10.1038/s41575-020-0281-0
- Zhong C, Xie HY, Zhou L, Xu X, Zheng SS. Human hepatocytes loaded in 3D bioprinting generate mini-liver. Hepatobiliary Pancreat Dis Int. 2016;15(5):512-518. doi: 10.1016/S1499-3872(16)60119-4
- Zhao Y, Xu B, Liang W, et al. Multisite injection of bioengineered hepatic units from collagen hydrogel and neonatal liver cells in parenchyma improves liver cirrhosis. Tissue Eng Part A. 2019;25(15-16):1167-1174. doi: 10.1089/ten.tea.2018.0107
- Shagidulin M, Onishchenko N, Sevastianov V, et al. Experimental correction and treatment of chronic liver failure using implantable cell-engineering constructs of the auxiliary liver based on a bioactive heterogeneous biopolymer hydrogel. Gels. 2023;9(6):456. doi: 10.3390/gels9060456
- Nagamoto Y, Takayama K, Ohashi K, et al. Transplantation of a human iPSC-derived hepatocyte sheet increases survival in mice with acute liver failure. J Hepatol. 2016;64(5):1068- 1075. doi: 10.1016/j.jhep.2016.01.004
- Baimakhanov Z, Yamanouchi K, Sakai Y, et al. Efficacy of multilayered hepatocyte sheet transplantation for radiation‑induced liver damage and partial hepatectomy in a rat model. Cell Transplant. 2016;25(3):549-558. doi: 10.3727/096368915X688669
- Demetriou AA, Levenson SM, Novikoff PM, et al. Survival, organization, and function of microcarrier-attached hepatocytes transplanted in rats. Proc Natl Acad Sci U S A. 1986; 83:7475-7479. doi: 10.1073/pnas.83.19.7475
- Jiang WC, Cheng YH, Yen MH, Chang Y, Yang VW, Lee OK. Cryo-chemical decellularization of the whole liver for mesenchymal stem cells-based functional hepatic tissue engineering. Biomaterials. 2014;35(11):3607-3617. doi: 10.1016/j.biomaterials.2014.01.024
- Bao J, Wu Q, Sun J, et al. Hemocompatibility improvement of perfusion-decellularized clinical-scale liver scaffold through heparin immobilization. Sci Rep. 2015;5(1):10756. doi: 10.1038/srep10756
- Sarkis R, Honiger J, Chafai N, et al. Semiautomatic macroencapsulation of fresh or cryopreserved porcine hepatocytes maintain their ability for treatment of acute liver failure. Cell Transplant. 2001;10(7):601-607. doi: 10.3727/000000001783986314
- Segovia-Zafra A, Di Zeo-Sánchez DE, López-Gómez C, et al. Preclinical models of idiosyncratic drug-induced liver injury (iDILI): moving towards prediction. Acta Pharm Sin B. 2021;11(12):3685-3726. doi: 10.1016/j.apsb.2021.11.013