Hydrogel bioink formulation for 3D bioprinting: Sustained delivery of PDGF-BB and VEGF in biomimetic scaffolds for tendon partial rupture repair
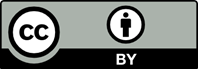
In the evolving field of tissue engineering, the power of three-dimensional (3D) bioprinting technology can be harnessed by innovative methodologies to address the complex challenges of treating partial tendon injuries. In order to engineer a solution for this type of musculoskeletal injuries, a biomimetic bioink and a scaffold developed using 3D bioprinting technology and capable of delivering cells and growth factors were investigated. For the development of the bioink, a hydrogel type structure was selected based on a strategic combination of alginate, hyaluronic acid, gelatin, and fibrinogen. This tailored combination exhibited favorable rheological behavior and impeccable printability. The bioink, demonstrating promising characteristics, was then employed to fabricate both acellular scaffolds and tissue constructs. The structures possessed mechanical properties suitable and adequate for addressing partial tendon injuries and achieved a microenvironment that allowed good metabolic activity of tenocytes, maintenance of their phenotype, and overexpression of genes related to macromolecules of tendon extracellular matrix. Regarding growth factors delivery, vascular endothelial growth factor (VEGF165) and platelet-derived growth factor (PDGF-BB) were successfully incorporated into the bioink. Their release profile was thoroughly studied, and their activity once released was demonstrated. Together, these results suggest that the developed bioink and the resulting 3D structures can have an important impact on tendon partial injury therapies. The multifaceted capabilities of the bioink and the developed tissue constructs position them as crucial contributors to the advancement of tendon injury therapies, marking a significant stride toward enhanced patient outcomes and regenerative medicine practices.
- Cieza A, Causey K, Kamenov K, Hanson SW, Chatterji S, Vos T. Global estimates of the need for rehabilitation based on the Global Burden of Disease study 2019: a systematic analysis for the Global Burden of Disease Study 2019. Lancet. 2021;396(10267):2006-2017. doi: 10.1016/S0140-6736(20)32340-0
- Liu S, Wang B, Fan S, Wang Y, Zhan Y, Ye D. Global burden of musculoskeletal disorders and attributable factors in 204 countries and territories: a secondary analysis of the Global Burden of Disease 2019 study. BMJ Open. 2022;12(6):e062183-062183. doi: 10.1136/bmjopen-2022-062183
- National Academies of Sciences, Engineering, and Medicine; Health and Medicine Division; Board on Health Care Services; Committee on Identifying Disabling Medical Conditions Likely to Improve with Treatment. Selected Health Conditions and Likelihood of Improvement with Treatment. Washington, DC: National Academies Press; 2020.
- Longo UG, Denaro V. Textbook of Musculoskeletal Disorders. 1st ed. Switzerland AG: Springer Cham; 2023. doi: 10.1007/978-3-031-20987-1
- Mahler F, Fritschy D. Partial and complete ruptures of the Achilles tendon and local corticosteroid injections. Br J Sports Med. 1992;26(1):7-14. doi: 10.1136/bjsm.26.1.7
- Gatz M, Spang C, Alfredson H. Partial Achilles tendon rupture-a neglected entity: a narrative literature review on diagnostics and treatment options. J Clin Med. 2020;9(10):3380. doi: 10.3390/jcm9103380
- Riggin CN, Morris TR, Soslowsky LJ. Tendinopathy II: etiology, pathology, and healing of tendon injury and disease. In: Gomes ME, Reis RL, Rodrigues MT, eds. Tendon Regeneration. Academic Press; 2015:149-183. doi: 10.1016/B978-0-12-801590-2.00005-3
- Andarawis-Puri N, Flatow EL, Soslowsky LJ. Tendon basic science: development, repair, regeneration, and healing. J Orthop Res. 2015;33(6):780-784. doi: 10.1002/jor.22869
- Kwan TD, El Haj AJ. Multifactorial tendon tissue engineering strategies. In: Gomes ME, Reis RL, Rodrigues MT, eds. Tendon Regeneration. Cambridge, Massachusetts, US: Academic Press; 2015:281-320. doi: 10.1016/B978-0-12-801590-2.00011-9
- Engebretson B, Mussett Z, Williams C, Simmons A, Sikavitsas V. Tendon tissue engineering: combined tissue engineering approach for the regeneration of tendons. In: Gomes ME, Reis RL, Rodrigues MT, eds. Tendon Regeneration. Cambridge, Massachusetts, US: Academic Press; 2015:281-320. doi: 10.1016/B978-0-12-801590-2.00011-9
- Bianchi E, Ruggeri M, Rossi S, et al. Innovative strategies in tendon tissue engineering. Pharmaceutics. 2021;13(1):89. doi: 10.3390/pharmaceutics13010089
- Matai I, Kaur G, Seyedsalehi A, McClinton A, Laurencin CT. Progress in 3D bioprinting technology for tissue/organ regenerative engineering. Biomaterials. 2020;226:119536. doi: 10.1016/j.biomaterials.2019.119536
- Ruiz-Alonso S, Lafuente-Merchan M, Ciriza J, Saenz-Del- Burgo L, Pedraz JL. Tendon tissue engineering: cells, growth factors, scaffolds and production techniques. J Control Release. 2021;333:448-486. doi: 10.1016/j.jconrel.2021.03.040
- Chen XB, Anvari-Yazdi AF, Duan X, et al. Biomaterials / bioinks and extrusion bioprinting. Bioact Mater. 2023;28:511-536. doi: 10.1016/j.bioactmat.2023.06.006
- ASTM International. ISO/ASTM 52900 2021(E). Additive Manufacturing—General Principles—Fundamentals and Vocabulary. Geneva: ASTM International; 2021:1–5.
- Hospodiuk M, Moncal KK, Dey M, Ozbolat IT. Extrusion-based biofabrication in tissue engineering and regenerative medicine. In Ovsianikov A, Yoo J, Mironov V, eds. 3D Printing and Biofabrication. New York City, New York, US: Springer International Publishing; 2016:1-27. doi: 10.1007/978-3-319-40498-1_10-1
- Kaliaraj GS, Shanmugam DK, Dasan A, Mosas KK. Hydrogels—a promising materials for 3D printing technology. Gels. 2023;9(3). doi: 10.3390/gels9030260
- Li X, Sun Q, Li Q, Kawazoe N, Chen G. Functional hydrogels with tunable structures and properties for tissue engineering applications. Front Chem. 2018;6. doi: 10.3389/fchem.2018.00499
- Thang NH, Chien TB, Cuong DX. Polymer-based hydrogels applied in drug delivery: an overview. Gels. 2023; 9(7):523. doi: 10.3390/gels9070523
- Schwab A, Levato R, D’Este M, Piluso S, Eglin D, Malda J. Printability and shape fidelity of bioinks in 3D bioprinting. Chem Rev. 2020;120(19):11028-11055. doi: 10.1021/acs.chemrev.0c00084
- Lin X, Zhao X, Xu C, Wang L, Xia Y. Progress in the mechanical enhancement of hydrogels: fabrication strategies and underlying mechanisms. J Polym Sci. 2022;60(17):2525-2542. doi: 10.1002/pol.20220154
- Hu J, Liu S, Fan C. Applications of functionally-adapted hydrogels in tendon repair. Front Bioeng Biotechnol. 2023;11:1135090. doi: 10.3389/fbioe.2023.1135090
- Tabata Y. Tissue regeneration based on growth factor release. Tissue Eng. 2003;9:5-15. doi: 10.1089/10763270360696941
- Koria P. Delivery of growth factors for tissue regeneration and wound healing. BioDrugs. 2012;26(3):163-175. doi: 10.2165/11631850-000000000-00000
- Creaney L, Hamilton B. Growth factor delivery methods in the management of sports injuries: the state of play. Br J Sports Med. 2008;42(5):314. doi: 10.1136/bjsm.2007.040071
- Kuroda Y, Kawai T, Goto K, Matsuda S. Clinical application of injectable growth factor for bone regeneration: a systematic review. Inflamm Regen. 2019;39(1):20. doi: 10.1186/s41232-019-0109-x
- Lee K, Silva EA, Mooney DJ. Growth factor delivery-based tissue engineering: general approaches and a review of recent developments. J R Soc Interface. 2011;8(55):153-170. doi: 10.1098/rsif.2010.0223
- Wang Z, Wang Z, Lu WW, Zhen W, Yang D, Peng S. Novel biomaterial strategies for controlled growth factor delivery for biomedical applications. NPG Asia Mater. 2017;9(10):e435. doi: 10.1038/am.2017.171
- Li H, Luo S, Wang H, et al. The mechanisms and functions of TGF-β1 in tendon healing. Injury. 2023;54(11):111052. doi: 10.1016/j.injury.2023.111052
- Evrova O, Buschmann J. In vitro and in vivo effects of PDGF-BB delivery strategies on tendon healing: a review. Eur Cell Mater. 2017;34:15-39. doi: 10.22203/eCM.v034a02
- Wang Y, Li J. Current progress in growth factors and extracellular vesicles in tendon healing. Int Wound J. 2023;20(9):3871-3883. doi: 10.1111/iwj.14261
- Molloy T, Wang Y, Murrell G. The roles of growth factors in tendon and ligament healing. Sports Med. 2003;33(5): 381-394. doi: 10.2165/00007256-200333050-00004
- Miescher I, Rieber J, Calcagni M, Buschmann J. In vitro and in vivo effects of IGF-1 delivery strategies on tendon healing: a review. Int J Mol Sci. 2023;24(3):2370. doi: 10.3390/ijms24032370
- Leong NL, Kator JL, Clemens TL, James A, Enamoto- Iwamoto M, Jiang J. Tendon and ligament healing and current approaches to tendon and ligament regeneration. J Orthop Res. 2020;38(1):7-12. doi: 10.1002/jor.24475
- Hope M, Saxby TS. Tendon healing. Foot Ankle Clin. 2007;12(4):553-567. doi: 10.1016/j.fcl.2007.07.003
- Hee CK, Dines JS, Solchaga LA, Shah VR, Hollinger JO. Regenerative tendon and ligament healing: opportunities with recombinant human platelet-derived growth factor BB-homodimer. Tissue Eng. Part B Rev. 2012;18(3): 225-234. doi: 10.1089/ten.teb.2011.0603
- Naderi H, Matin MM, Bahrami AR. Review paper: critical issues in tissue engineering: biomaterials, cell sources, angiogenesis, and drug delivery systems. J Biomater Appl. 2011;26(4):383-417. doi: 10.1177/0885328211408946
- Naghieh S, Chen X. Printability–a key issue in extrusion-based bioprinting. J Pharm Anal. 2021;11(5):564-579. doi: 10.1016/j.jpha.2021.02.001
- Małkowski P, Ostrowski L, Brodny J. Analysis of Young’s modulus for carboniferous sedimentary rocks and its relationship with uniaxial compressive strength using different methods of modulus determination. J Sustain Min. 2018;17(3):145-157. doi: 10.1016/j.jsm.2018.07.002
- Barreto IS, Pierantoni M, Hammerman M, et al. Nanoscale characterization of collagen structural responses to in situ loading in rat Achilles tendons. Matrix Biol. 2023;115:32-47. doi: 10.1016/j.matbio.2022.11.006
- Lukin I, Erezuma I, Maeso L, et al. Progress in gelatin as biomaterial for tissue engineering. Pharmaceutics. 2022;14(6):1177. doi: 1110.3390/pharmaceutics14061177
- Saravanakumar K, Park S, Santosh SS, et al. Application of hyaluronic acid in tissue engineering, regenerative medicine, and nanomedicine: a review. Int J Biol Macromol. 2022;222:2744-2760. doi: 10.1016/j.ijbiomac.2022.10.055
43 Farshidfar N, Iravani S, Varma RS. Alginate-based biomaterials in tissue engineering and regenerative medicine. Mar Drugs. 2023;21(3):189. doi: 10.3390/md21030189
- Sanz-Horta R, Matesanz A, Gallardo A, et al. Technological advances in fibrin for tissue engineering. J Tissue Eng. 2023;14:20417314231190288. doi: 10.1177/20417314231190288
- Bird B, Armstrong RC, Hassager O. Dynamics of Polymeric Liquids. Volume 1 Fluid Mechanics. Hoboken, New Jersey, US: John Wiley & Sons; 1987.
- Cooke ME, Rosenzweig DH. The rheology of direct and suspended extrusion bioprinting. APL Bioeng. 2021;5(1):011502. doi: 10.1063/5.0031475
- Thompson RL, Sica LUR, de Souza Mendes PR. The yield stress tensor. J Non Newtonian Fluid Mech. 2018;261:211-219. doi: 10.1016/j.jnnfm.2018.09.003
- Mezger TG. The Rheology Handbook. 4th ed. Hanover, Germany: Vincentz Network; 2014.
- Mortimer S, Ryan AJ, Stanford JL. Rheological behavior and gel-point determination for a model lewis acid-initiated chain growth epoxy resin. Macromolecules. 2001;34(9):2973-2980. doi: 10.1021/ma001835x
- Augst AD, Kong HJ, Mooney DJ. Alginate hydrogels as biomaterials. Macromol Biosci. 2006;6(8):623-633. doi: 10.1002/mabi.200600069
- Whelihan MF, Kiankhooy A, Brummel-Ziedins K. Thrombin generation and fibrin clot formation under hypothermic conditions: an in vitro evaluation of tissue factor initiated whole blood coagulation. J Crit Care. 2014;29(1):24-30. doi: 10.1016/j.jcrc.2013.10.010
- Tang JD, Caliari SR, Lampe KJ. Temperature-dependent complex coacervation of engineered elastin-like polypeptide and hyaluronic acid polyelectrolytes. Biomacromolecules. 2018;19(10):3925-3935. doi: 10.1021/acs.biomac.8b00837
- Boularaoui S, Al Hussein G, Khan KA, Christoforou N, Stefanini C. An overview of extrusion-based bioprinting with a focus on induced shear stress and its effect on cell viability. Bioprinting. 2020;20:e00093. doi: 10.1016/j.bprint.2020.e00093
- Fakhruddin K, Hamzah MSA, Razak SIA. Effects of extrusion pressure and printing speed of 3D bioprinted construct on the fibroblast cells viability. IOP Conf Ser: Mater Sci Eng. 2018;440(1):012042. doi: 10.1088/1757-899X/440/1/012042
- Xu H, Liu J, Zhang Z, Xu C. A review on cell damage, viability, and functionality during 3D bioprinting. Mil Med Res. 2022;9(1):70. doi: 10.1186/s40779-022-00429-5
- Wang J, Wei Y, Zhao S, et al. The analysis of viability for mammalian cells treated at different temperatures and its application in cell shipment. PLoS One. 2017;12(4):e0176120. doi: 10.1371/journal.pone.0176120
- Murphy CM, O’Brien FJ. Understanding the effect of mean pore size on cell activity in collagen-glycosaminoglycan scaffolds. Cell Adh Migr. 2010;4(3):377-381. doi: 10.4161/cam.4.3.11747
- Loh QL, Choong C. Three-dimensional scaffolds for tissue engineering applications: role of porosity and pore size. Tissue Eng Part B Rev. 2013;19(6):485-502. doi: 10.1089/ten.TEB.2012.0437
- Voleti PB, Buckley MR, Soslowsky LJ. Tendon healing: repair and regeneration. Annu Rev Biomed Eng. 2012;14(1):47-71. doi: 10.1146/annurev-bioeng-071811-150122
- Rolnick KI, Choe JA, Leiferman EM, et al. Periostin modulates extracellular matrix behavior in tendons. Matrix Biol Plus. 2022;16:100124. doi: 10.1016/j.mbplus.2022.100124
- Luo T, Tan B, Zhu L, Wang Y, Liao J. A review on the design of hydrogels with different stiffness and their effects on tissue repair. Front Bioeng Biotechnol. 2022;10:817391. doi: 10.3389/fbioe.2022.817391
- Abalymov A, Parakhonskiy B, Skirtach AG. Polymer- and hybrid-based biomaterials for interstitial, connective, vascular, nerve, visceral and musculoskeletal tissue engineering. Polymers. 2020;12(3):620. doi: 10.3390/polym12030620
- Cox TR, Erler JT. Remodeling and homeostasis of the extracellular matrix: implications for fibrotic diseases and cancer. Dis Model Mech. 2011;4(2):165-178. doi: 10.1242/dmm.004077
- Saha K, Pollock JF, Schaffer DV, Healy KE. Designing synthetic materials to control stem cell phenotype. Curr Opin Chem Biol. 2007;11(4):381-387. doi: 10.1016/j.cbpa.2007.05.030
- Hwang NS, Varghese S, Elisseeff J. Controlled differentiation of stem cells. Adv Drug Deliv Rev. 2008;60(2):199-214. doi: 10.1016/j.addr.2007.08.036
- O’Brien FJ. Biomaterials & scaffolds for tissue engineering. Mater Today. 2011;14(3):88-95. doi: 10.1016/S1369-7021(11)70058-X
- Zhang X, Kim T, Thauland TJ, et al. Unraveling the mechanobiology of immune cells. Curr Opin Biotechnol. 2020;66:236-245. doi: 10.1016/j.copbio.2020.09.004
- Breuls RGM, Jiya TU, Smit TH. Scaffold stiffness influences cell behavior: opportunities for skeletal tissue engineering. Open Orthop J. 2008;2:103-109. doi: 10.2174/1874325000802010103
- Schuurman W, Levett PA, Pot MW, et al. Gelatin-methacrylamide hydrogels as potential biomaterials for fabrication of tissue-engineered cartilage constructs. Macromol Biosci. 2013;13(5):551-561. doi: 10.1002/mabi.201200471
- Zhao X, Lang Q, Yildirimer L, et al. Photocrosslinkable gelatin hydrogel for epidermal tissue engineering. Adv Healthc Mater. 2016;5(1):108-118. doi: 10.1002/adhm.201500005
- Luo Q, Song G, Song Y, Xu B, Qin J, Shi Y. Indirect co-culture with tenocytes promotes proliferation and mRNA expression of tendon/ligament related genes in rat bone marrow mesenchymal stem cells. Cytotechnology. 2009;61(1-2):1-10. doi: 10.1007/s10616-009-9233-9
- Güngörmüş C, Kolankaya D. Gene expression of tendon collagens and tenocyte markers in long-term monolayer and high-density cultures of rat tenocytes. Connect Tissue Res. 2012;53(6):485-491. doi: 10.3109/03008207.2012.694511