Bottom-up and top-down VAT photopolymerization bioprinting for rapid fabrication of multi-material microtissues
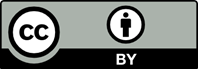
Over the years, three-dimensional (3D) bioprinting has attracted attention for being a highly automated manufacturing system that allows for the precise design of living constructs where cells and biomaterials are displaced in predefined positions to recreate cell–matrix and cell–cell interactions similar to native tissues. Such technologies rarely offer multi-material features. In this paper, we present a new approach for bioprinting of multi-material tissue constructs using VAT photopolymerization at high resolution and fidelity. We developed a versatile dual-mode bioprinter that can easily be modulated to print in both top-down and bottom-up approaches. The custom-built platform was then used to fabricate microtissues and hydrogel microfluidic models. Combining bottom-up and top-down biofabrication tools can offer an optimal solution for hard–soft multi-material composites and for bioprinting tissue–tissue interface models. We demonstrated the possibility for hard–soft multi-material bioprinting by generating musculoskeletal tissue with integrated microvasculature. Combining multiple material bioprinting and microfluidic chips shows advantages in two aspects: precise regulation of microenvironment and accurate emulation of multi-tissue interfaces.
- Wu Q, Liu J, Wang X, et al. Organ-on-a-chip: Recent breakthroughs and future prospects. Biomed Eng Online. 2020;19:1-19.
- Bhatia SN, Ingber DE. Microfluidic organs-on-chips. Nat Biotechnol. 2014;32(8): 760-772.
- Mathur A, Loskill P, Shao K, et al. Human iPSC-based cardiac microphysiological system for drug screening applications. Sci Rep. 2015;5:1-7.
- Neužil P, Giselbrecht S, Länge K, Huang TJ, Manz A. Revisiting lab-on-a-chip technology for drug discovery. Nat Rev Drug Discov. 2012;11(8):620-632.
- Ribas J, Sadeghi H, Manbachi A, et al. Cardiovascular organ-on-a-chip platforms for drug discovery and development. Appl In Vitro Toxicol. 2016;2:82-96.
- Ho CT, Lin RZ, Chen RJ, et al. Liver-cell patterning lab chip: Mimicking the morphology of liver lobule tissue. Lab Chip. 2013;13:3578-3587.
- Booth R, Kim H. Characterization of a microfluidic in vitro model of the blood-brain barrier (μBBB). Lab Chip. 2012;12(10):1784-1792.
- Lee SH, Jun B-H. Advances in dynamic microphysiological organ-on-a-chip: Design principle and its biomedical application. J Indus Eng Chem. 2019;(71):65-77.
- Huh D, Matthews BD, Mammoto A, et al. Reconstituting organ-level lung functions on a chip. Science. 2010;328(5986):1662-1668.
- Beckwitt CH, Clark AM, Wheeler S, et al. Liver ‘organ on a chip’. Exp Cell Res. 2018;63(1):15-25.
- Kim HJ, Huh D, Hamilton G, Ingber DE. Human gut-on-a-chip inhabited by microbial flora that experiences intestinal peristalsis-like motions and flow. Lab Chip. 2012;12: 2165-2174.
- Wilmer MJ, Ng CP, Lanz HL, Vulto P, Suter-Dick L, Masereeuw R. Kidney-on-a-chip technology for drug-induced nephrotoxicity screening. Trends Biotechnol. 2016;34(2):156-170.
- Phan DT, Bender RHF, Andrejecsk JW, et al. Blood–brain barrier-on-a-chip: Microphysiological systems that capture the complexity of the blood–central nervous system interface. Exp Biol Med. 2017;242(17):1669-1678.
- Huebsch N, Loskill P, Deveshwar N, et al. Miniaturized iPS-cell-derived cardiac muscles for physiologically relevant drug response analyses. Sci Rep. 2016;(6):1-12.
- Mandrycky C, Wang Z, Kim K, Kim D-H. 3D bioprinting for engineering complex tissues. Biotechnol Adv. 2016;34(4): 422-434.
- Arrigoni C, Lopa S, Candrian C, Moretti M. Organs-on-a-chip as model systems for multifactorial musculoskeletal diseases. Curr Opin Biotechnol. 2020;63:79-88.
- Datta P, Dey M, Ataie Z, Unutmaz D, Ozbolat IT. 3D bioprinting for reconstituting the cancer microenvironment. NPJ Precis Oncol. 2020;4:1-13.
- Xiang Y, Miller K, Guan J, Kiratitanaporn W, Tang M, Chen S. 3D bioprinting of complex tissues in vitro: State-of-the-art and future perspectives. Arch Toxicol. 2022;96(3):691-710 .
- Jiang T, Munguia-Lopez JG, Flores-Torres S, Kort-Mascort J, Kinsella JM. Extrusion bioprinting of soft materials: An emerging technique for biological model fabrication. Appl Phys Rev. 2019;l6(1):011310.
- Ozbolat IT, Hospodiuk M. Current advances and future perspectives in extrusion‐based bioprinting. Biomaterials. 2016;76:76321-76343.
- Li X, Liu B, Pei B, et al. Inkjet Bioprinting of Biomaterials. Chem Rev. 2020;120(19):10793-10833.
- Ng WL, Lee JM, Zhou M, et al. Vat polymerization-based bioprinting-process, materials, applications and regulatory challenges. Biofabrication. 2020;12(2):022001.
- Antoshin AA, Churbanov SN, Minaev NV, et al. LIFT‐bioprinting, is it worth it? Biofabrication. 2019;15:e00052.
- Grigoryan B, Sazer DW, Avila A, et al. Development, characterization, and applications of multi-material stereolithography bioprinting. Sci Rep. 2021;11(1):3171.
- Zhang R, Larsen NB. Stereolithographic hydrogel printing of 3D culture chips with biofunctionalized complex 3D perfusion networks. Lab Chip. 2017;17(24):4273-4282.
- Miri AK, Nieto D, Iglesias L, et al. Microfluidics‐enabled multimaterial maskless stereolithographic bioprinting. Adv Mater. 2018;30:1800242.
- Grogan SP, Chung PH, Soman P, et al. Digital micromirror device projection printing system for meniscus tissue engineering. Acta Biomater. 2013;9(7):7218-7226.
- Bhusal A, Dogan E, Nguyen HA, et al. Multi-material digital light processing bioprinting of hydrogel-based microfluidic chips. Biofabrication. 2021;14:014103.
- Dogan E, Bhusal A, Cecen B, Miri AK. Miri. 3D printing metamaterials towards tissue engineering. Appl Mater Today. 2020;20:100752.
- Mobaraki M, Ghaffari M, Yazdanpanah A, Luo Y, Mills DK. Bioinks and bioprinting: A focused review. Bioprinting. 2020;18:e00080.
- Kolesky DB, Homan KA, Skylar-Scott MA, Lewis JA. Three-dimensional bioprinting of thick vascularized tissues. Proc Natl Acad Sci. 2016;113(12):3179-3184.
- Homan KA, Kolesky DB, Skylar-Scott MA, et al. Bioprinting of 3D convoluted renal proximal tubules on perfusable chips. Sci Rep. 2016;6:34845.
- Zhu W, Qu X, Zhu J, et al. Direct 3D bioprinting of prevascularized tissue constructs with complex microarchitecture. Biomaterials. 2017;124:106–115.
- Miri AK, Mirzaee I, Hassan S, et al. Effective bioprinting resolution in tissue model fabrication. Lab Chip. 2019;19(11):2019-2037.
- Miri AK, Mostafavi E, Khorsandi D, Hu S-K, Malpica M, Khademhosseini A. Bioprinters for organs-on-chips. Biofabrication. 2019;11(4):042002.
- Zheng Z, Eiglin D, Alini M, Richards GR, Qin L, Lai Y. Visible light-induced 3D bioprinting technologies and corresponding bioink materials for tissue engineering: A review. Engineering. 2021;7:966-978.
- Cook AB, Clemons TD. Bottom‐up versus top‐down strategies for morphology control in polymer‐based biomedical materials. Adv NanoBiomed Res. 2022;2(1): 2100087.
- Kumar H, Kim K. Stereolithography 3D bioprinting. Methods Mol Biol. 2020;2140:93-108.
- Li W, Wang M, Ma H, Chapa-Villarreal abiola A, Lobo AO, Zhang YS. Stereolithography apparatus and digital light processing-based 3D bioprinting for tissue fabrication. iScience. 2023;26(2):106039.
- Kuhnt T, Marroquín R, Camarero-Espinosa S, et al. Poly (caprolactone-co-trime thylenecarbonate) urethane acrylate resins for digital light processing of bioresorbable tissue engineering implants. Biomater Sci, 2019;7(12):4984-4989.
- Nieto D, Jiménez G, Moroni L, López‐Ruiz E, Gálvez‐Martín P, Marchal JA. Biofabrication approaches and regulatory framework of metastatic tumor-on-a-chip models for precision oncology. Med Res Rev. 2021;42(5):1978-2001.
- Hu Y, Zhang H, Wang S, et al. Bone/cartilage organoid on-chip: Construction strategy and application. Bioact Mater. 2023;25:29-41.
- Bowles RD, Setton LA. Biomaterials for intervertebral disc regeneration and repair. Biomaterials. 2017;129:54-67.
- Hosseinabadi HG, Nieto D, Yousefinejad A, Fattel H, Ionov L, Miri AK. Ink material selection and optical design considerations in DLP 3D printing. Appl Mater Today. 2023;30:101721.
- Scaricamazza S, Salvatori I, Ferri A, Valle C. Skeletal muscle in ALS: An unappreciated therapeutic opportunity? Cells. 2021;10(3):525. https://doi.org/10.3390/cells10030525
- Paganoni S, Karam C, Joyce N, Bedlack R, Carter GT. Comprehensive rehabilitative care across the spectrum of amyotrophic lateral sclerosis. NeuroRehabilitation. 2015;37(1):53-68. https://doi.org/10.3233/nre-151240
- Zhou SW, Wang J, Chen SY, Ren K-F, Wang Y-X, Ji J. The substrate stiffness at physiological range significantly modulates vascular cell behavior. Colloids Surf B Biointerfaces. 2022;(214):112483.
- Lee Y, Kim M, Lee H. The measurement of stiffness for major muscles with shear wave elastography and myoton: A quantitative analysis study. Diagnostics. 2021;11(3):524.
- Singh G, Chanda A. Mechanical properties of whole-body soft human tissues: A review. Biomed Mater. 2021;16(6):062004.
- Huh D, Hamilton GA, Ingber DE. From 3D cell culture to organs-on-chips. Trends Cell Biol. 2011;21(12):745-754.
- Low LA, Mummery C, Berridge BR, Austin CP, Tagle DA. Organs-on-chips: Into the next decade. Nat Rev Drug Discov, 2021;20(5):345-361.
- Ajalik RE, Alenchery RG, Cognetti JS, et al. Human organ-on-a-chip microphysiological systems to model musculoskeletal pathologies and accelerate therapeutic discovery. Front Bioeng Biotechnol. 2022;(10):846230.
- Scaricamazza S, Salvatori I, Ferri A, Valle C. Skeletal muscle in ALS: An unappreciated therapeutic opportunity? Cells. 2021;10(3):525. doi: 10.3390/cells10030525
- Ma J, Holden K, Zhu J, Pan H, Li Y. The application of three-dimensional collagen-scaffolds seeded with myoblasts to repair skeletal muscle defects. J Biomed Biotechnol. 2011;2011: 812135. doi: 10.1155/2011/812135
- Pan C-C, Bruyas A, Yang YP. Bioprinting for tissue engineering and regenerative medicine. Mater Matt Aldrich. 2016;(11):49-52.
- Rainbow R, Ren W, Zeng L. Inflammation and joint tissue interactions in OA: Implications for potential therapeutic approaches. Arthritis. 2012;741582.
- Pirosa A, Gottardi R, Alexander PG, Tuan RS. Engineering in-vitro stem cell-based vascularized bone models for drug screening and predictive toxicology. Stem Cell Res Ther. 2018;9: 112. doi: 10.1186/s13287-018-0847-8
- McDowell SAC, Quail DF. Immunological regulation of vascular inflammation during cancer metastasis. Front Immunol. 2019;10:1984.
- Goel S, Duda DG, Xu L, et al. Normalization of the vasculature for treatment of cancer and other diseases. Physiol Rev. 2011;91(3):1071-1121. https://doi.org/10.1152/physrev.00038.2010
- Naghieh S, Lindberg G, Tamaddon M, Liu C. Biofabrication strategies for musculoskeletal disorders: Evolution towards clinical applications. Bioengineering (Basel). 2021;8(9):123.
- Volpi M, Paradiso A, Costantini M, Świȩszkowski W. Hydrogel-based fiber biofabrication techniques for skeletal muscle tissue engineering. ACS Biomater Sci Eng. 2022;8:379-405.