Effect of tunable stiffness on immune responses in 3D-bioprinted alginate–gelatin scaffolds
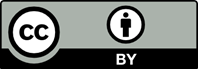
Tissue engineering is an approach used to restore damaged tissues and organs using biomaterials that support cell adhesion, growth, and proliferation. However, immune responses triggered by tissue injury and biomaterial implantation can lead to undesired reactions such as foreign body response and fibrotic capsule formation. Macrophages play a critical role in these immune responses. Therefore, comprehending and controlling the immune responses to biomaterials are crucial for successful clinical translation in tissue engineering. In this experimental study, we fabricated three-dimensional-bioprinted hydrogel scaffolds with adaptable stiffness by adjusting the alginate–gelatin ratio. We examined the physical properties of these scaffolds and assessed the immune responses they provoked both in vitro and in vivo. Our results revealed that higher-stiffness implants could drive macrophage polarization toward pro-inflammatory phenotypes in vivo. Furthermore, our animal experiments demonstrated that high-stiffness hydrogels elicited elevated immune responses through the TLR4/Myd88/NF-κB signaling pathway and IL-6/JAK-STAT signaling pathway. Collectively, our study demonstrates that increased implant stiffness correlates with stronger immune responses. These findings are expected to provide novel insights for the clinical application of alginate–gelatin composite hydrogels.
- Kim S, Uroz M, Bays JL, Chen CS. Harnessing mechanobiology for tissue engineering. Dev Cell. 2021;56(2):180-191. doi: 10.1016/j.devcel.2020.12.017
- Matai I, Kaur G, Seyedsalehi A, McClinton A, Laurencin CT. Progress in 3D bioprinting technology for tissue/organ regenerative engineering. Biomaterials. 2020;226:119536. doi: 10.1016/j.biomaterials.2019.119536
- Taraballi F, Sushnitha M, Tsao C, et al. Biomimetic tissue engineering: tuning the immune and inflammatory response to implantable biomaterials. Adv Healthc Mater. 2018;7(17):e1800490. doi: 10.1002/adhm.201800490
- Crupi A, Costa A, Tarnok A, Melzer S, Teodori L. Inflammation in tissue engineering: the Janus between engraftment and rejection. Eur J Immunol. 2015;45(12): 3222-3236. doi: 10.1002/eji.201545818
- Abaricia JO, Farzad N, Heath TJ, Simmons J, Morandini L, Olivares-Navarrete R. Control of innate immune response by biomaterial surface topography, energy, and stiffness. Acta Biomater. 2021;133:58-73. doi: 10.1016/j.actbio.2021.04.021
- Locati M, Curtale G, Mantovani A. Diversity, mechanisms, and significance of macrophage plasticity. Annu Rev Pathol. 2020;15(1):123-147. doi: 10.1146/annurev-pathmechdis-012418-012718
- Chen S, Saeed A, Liu Q, et al. Macrophages in immunoregulation and therapeutics. Signal Transduct Target Ther. 2023;8(1):207. doi: 10.1038/s41392-023-01452-1
- Al Sadoun H. Macrophage phenotypes in normal and diabetic wound healing and therapeutic interventions. Cells. 2022;11(15). doi: 10.3390/cells11152430
- Wynn TA, Vannella KM. Macrophages in tissue repair, regeneration, and fibrosis. Immunity. 2016;44(3):450-462. doi: 10.1016/j.immuni.2016.02.015
- Balabiyev A, Podolnikova NP, Kilbourne JA, et al. Fibrin polymer on the surface of biomaterial implants drives the foreign body reaction. Biomaterials. 2021;277. doi: 10.1016/j.biomaterials.2021.121087
- Anderson JM, Rodriguez A, Chang DT. Foreign body reaction to biomaterials. Semin Immunol. 2008;20(2): 86-100. doi: 10.1016/j.smim.2007.11.004
- Lee SC, Gillispie G, Prim P, Lee SJ. Physical and chemical factors influencing the printability of hydrogel-based extrusion bioinks. Chem Rev. 2020;120(19):10834-10886. doi: 10.1021/acs.chemrev.0c00015
- Bu W, Wu Y, Ghaemmaghami AM, Sun H, Mata A. Rational design of hydrogels for immunomodulation. Regen Biomater. 2022;9:rbac009. doi: 10.1093/rb/rbac009
- Camarero‐Espinosa S, Carlos‐Oliveira M, Liu H, Mano JF, Bouvy N, Moroni L. 3D printed dual‐porosity scaffolds: the combined effect of stiffness and porosity in the modulation of macrophage polarization. Adv Healthc Mater. 2021;11(1). doi: 10.1002/adhm.202101415
- Sridharan R, Cavanagh B, Cameron AR, Kelly DJ, O’Brien FJ. Material stiffness influences the polarization state, function and migration mode of macrophages. Acta Biomater. 2019;89:47-59. doi: 10.1016/j.actbio.2019.02.048
- Previtera ML, Sengupta A. Substrate stiffness regulates proinflammatory mediator production through TLR4 Activity in macrophages. PLoS One. 2015;10(12): e0145813. doi: 10.1371/journal.pone.0145813
- He H, Xiao Z, Zhou Y, et al. Zwitterionic poly(sulfobetaine methacrylate) hydrogels with optimal mechanical properties for improving wound healing in vivo. J Mater Chem B. 2019;7(10):1697-1707. doi: 10.1039/c8tb02590h
- Xing X, Wang Y, Zhang X, et al. Matrix stiffness‐mediated effects on macrophages polarization and their LOXL2 expression. FEBS J. 2020;288(11):3465-3477. doi: 10.1111/febs.15566
- Chen M, Zhang Y, Zhou P, et al. Substrate stiffness modulates bone marrow-derived macrophage polarization through NF-kappaB signaling pathway. Bioact Mater. 2020;5(4):880-890. doi: 10.1016/j.bioactmat.2020.05.004
- Ng WL, Huang X, Shkolnikov V, Suntornnond R, Yeong WY. Polyvinylpyrrolidone-based bioink: influence of bioink properties on printing performance and cell proliferation during inkjet-based bioprinting. Bio-Des Manuf. 2023;6(6):676-690. doi: 10.1007/s42242-023-00245-3
- Li X, Liu B, Pei B, et al. Inkjet bioprinting of biomaterials. Chem Rev. 2020;120(19):10793-10833. doi: 10.1021/acs.chemrev.0c00008
- Chartrain NA, Williams CB, Whittington AR. A review on fabricating tissue scaffolds using vat photopolymerization. Acta Biomater. 2018;2018(74):90-111. doi: 10.1016/j.actbio.2018.05.010
- Ramesh S, Harrysson OLA, Rao PK, et al. Extrusion bioprinting: recent progress, challenges, and future opportunities. Bioprinting. 2021;21. doi: 10.1016/j.bprint.2020.e00116
- Vijayavenkataraman S, Yan WC, Lu WF, Wang CH, Fuh JYH. 3D bioprinting of tissues and organs for regenerative medicine. Adv Drug Deliv Rev. 2018;132:296-332. doi: 10.1016/j.addr.2018.07.004
- Labowska MB, Cierluk K, Jankowska AM, Kulbacka J, Detyna J, Michalak I. A review on the adaption of alginate-gelatin hydrogels for 3D cultures and bioprinting. Materials. 2021;14(4). doi: 10.3390/ma14040858
- Jorgensen AM, Yoo JJ, Atala A. Solid organ bioprinting: strategies to achieve organ function. Chem Rev. 2020;120(19):11093-11127. doi: 10.1021/acs.chemrev.0c00145
- Giuseppe MD, Law N, Webb B, et al. Mechanical behaviour of alginate-gelatin hydrogels for 3D bioprinting. J Mech Behav Biomed Mater. 2018;79:150-157. doi: 10.1016/j.jmbbm.2017.12.018
- Liu Y, Li Z, Li J, et al. Stiffness-mediated mesenchymal stem cell fate decision in 3D-bioprinted hydrogels. Burns Trauma. 2020;8. doi: 10.1093/burnst/tkaa029
- Liang L, Li Z, Yao B, et al. Extrusion bioprinting of cellular aggregates improves mesenchymal stem cell proliferation and differentiation. Biomater Adv. 2023;149:213369. doi: 10.1016/j.bioadv.2023.213369
- Yu X, Wang Y, Zhang M, et al. 3D printing of gear-inspired biomaterials: immunomodulation and bone regeneration. Acta Biomater. 2023;156:222-233. doi: 10.1016/j.actbio.2022.09.008
- Rastogi P, Kandasubramanian B. Review of alginate-based hydrogel bioprinting for application in tissue engineering. Biofabrication. 2019;11(4). doi: 10.1088/1758-5090/ab331e
- Li J, Zhang Y, Enhe J, et al. Bioactive nanoparticle reinforced alginate/gelatin bioink for the maintenance of stem cell stemness. Mater Sci Eng C Mater Biol Appl. 2021;126:112193. doi: 10.1016/j.msec.2021.112193
- Wang X, Ji L, Wang J, Liu C. Matrix stiffness regulates osteoclast fate through integrin-dependent mechanotransduction. Bioact Mater. 2023;27:138-153. doi: 10.1016/j.bioactmat.2023.03.014
- Yi H-G, Kim H, Kwon J, Choi Y-J, Jang J, Cho D-W. Application of 3D bioprinting in the prevention and the therapy for human diseases. Signal Transduct Target Ther. 2021;6(1). doi: 10.1038/s41392-021-00566-8
- Di X, Gao X, Peng L, et al. Cellular mechanotransduction in health and diseases: from molecular mechanism to therapeutic targets. Signal Transduct Target Ther. 2023;8(1):282. doi: 10.1038/s41392-023-01501-9
- Eppler HB, Jewell CM. Biomaterials as tools to decode immunity. Adv Mater. 2019;32(13). doi: 10.1002/adma.201903367
- Bialik-Was K, Krolicka E, Malina D. Impact of the type of crosslinking agents on the properties of modified sodium alginate/poly(vinyl alcohol) hydrogels. Molecules. 2021;26(8). doi: 10.3390/molecules26082381
- Meli VS, Atcha H, Veerasubramanian PK, et al. YAP-mediated mechanotransduction tunes the macrophage inflammatory response. Sci Adv. 2020;6(49):eabb8471. doi: 10.1126/sciadv.abb8471
- Hsieh JY, Keating MT, Smith TD, et al. Matrix crosslinking enhances macrophage adhesion, migration, and inflammatory activation. APL Bioeng. 2019;3(1):016103. doi: 10.1063/1.5067301
- Sadtler K, Wolf MT, Ganguly S, et al. Divergent immune responses to synthetic and biological scaffolds. Biomaterials. 2019;192:405-415. doi: 10.1016/j.biomaterials.2018.11.002
- Wang D, Zhang S, Li L, Liu X, Mei K, Wang X. Structural insights into the assembly and activation of IL-1beta with its receptors. Nat Immunol. 2010;11(10):905-911. doi: 10.1038/ni.1925
- Bogdan C. Nitric oxide synthase in innate and adaptive immunity: an update. Trends Immunol. 2015;36(3): 161-178. doi: 10.1016/j.it.2015.01.003
- Amarante-Mendes GP, Adjemian S, Branco LM, Zanetti LC, Weinlich R, Bortoluci KR. Pattern recognition receptors and the host cell death molecular machinery. Front Immunol. 2018;9:2379. doi: 10.3389/fimmu.2018.02379
- Shekarian T, Valsesia-Wittmann S, Brody J, et al. Pattern recognition receptors: immune targets to enhance cancer immunotherapy. Ann Oncol. 2017;28(8):1756-1766. doi: 10.1093/annonc/mdx179
- Kim HJ, Kim H, Lee JH, Hwangbo C. Toll-like receptor 4 (TLR4): new insight immune and aging. Immun Ageing. 2023;20(1):67. doi: 10.1186/s12979-023-00383-3
- Brubaker SW, Bonham KS, Zanoni I, Kagan JC. Innate immune pattern recognition: a cell biological perspective. Annu Rev Immunol. 2015;33:257-290. doi: 10.1146/annurev-immunol-032414-112240
- Yu H, Lin L, Zhang Z, Zhang H, Hu H. Targeting NF-kappaB pathway for the therapy of diseases: mechanism and clinical study. Signal Transduct Target Ther. 2020;5(1):209. doi: 10.1038/s41392-020-00312-6
- Banerjee S, Biehl A, Gadina M, Hasni S, Schwartz DM. JAK-STAT signaling as a target for inflammatory and autoimmune diseases: current and future prospects. Drugs. 2017;77(5):521-546. doi: 10.1007/s40265-017-0701-9
- Hu X, Li J, Fu M, Zhao X, Wang W. The JAK/STAT signaling pathway: from bench to clinic. Signal Transduct Target Ther. 2021;6(1):402. doi: 10.1038/s41392-021-00791-1