3D bioprinting strategy for engineering vascularized tissue models
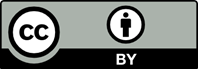
Leveraging three-dimensional (3D) bioprinting in the fields of tissue engineering and regenerative medicine has rapidly accelerated progress toward the development of living tissue constructs and biomedical devices. Ongoing vigorous research has pursued the development of 3D in vitro tissue models to replicate the key aspects of human physiology by incorporating relevant cell populations and adequate environmental cues. Given their advantages of being able to intimately mimic the heterogeneity and complexity of their native counterparts, 3D in vitro models hold promise as alternatives to conventional cell cultures or animal models for translational application to model human physiology/pathology and drug screening. Research has highlighted the importance of in vitro models, and a sophisticated biomanufacturing strategy is vitally required. In particular, vascularization is critical for the prolonged survival and functional maturation of the engineered tissues, which has remained one of the major challenges in the establishment of physiologically relevant 3D in vitro models. To this end, 3D bioprinting can efficiently generate solid and reproducible vascularized tissue models with high architectural and compositional similarity to the native tissues, leading to improve the structural maturation and tissue-specific functionality. Multiple bioprinting strategies have been developed to vascularize in vitro tissues by spatially controlled patterning of vascular precursors or generating readily perfusable vascular structures. This review presents an overview of the advanced 3D bioprinting strategies for vascularized tissue model development. We present the key elements for rebuilding functional vasculature in 3D-bioprinted tissue models and discuss the recent achievements in the engineering of 3D vascularized in vitro models using 3D bioprinting. Finally, we delineate the current challenges and future outlooks of 3D bioprinting-based vascularized tissue models.
Leung CM, de Haan P, Ronaldson-Bouchard K, et al., 2022, A guide to the organ-on-a-chip. Nat Rev Methods Primers, 2(1): 33. https://doi.org/10.1038/s43586-022-00118-6
Wu Q, Liu J, Wang X, et al., 2020, Organ-on-a-chip: Recent breakthroughs and future prospects. Biomed Eng Online, 19(1): 9. https://doi.org/10.1186/s12938-020-0752-0
Fetah K, Tebon P, Goudie MJ, et al., 2019, The emergence of 3D bioprinting in organ-on-chip systems. Prog Biomed Eng, 1(1): 012001. https://dx.doi.org/10.1088/2516-1091/ab23df
Yi H-G, Kim H, Kwon J, et al., 2021, Application of 3D bioprinting in the prevention and the therapy for human diseases. Signal Transduct Target Ther, 6(1): 177. https://doi.org/10.1038/s41392-021-00566-8
Ingber DE, 2022, Human organs-on-chips for disease modelling, drug development and personalized medicine. Nat Rev Genet, 23(8): 467–491. https://doi.org/10.1038/s41576-022-00466-9
Mota C, Camarero-Espinosa S, Baker MB, et al., 2020, Bioprinting: From tissue and organ development to in vitro models. Chem Rev, 120(19): 10547–10607. https://doi.org/10.1021/acs.chemrev.9b00789
O’Connor C, Brady E, Zheng Y, et al., 2022, Engineering the multiscale complexity of vascular networks. Nat Rev Mater, 7(9): 702–716. https://doi.org/10.1038/s41578-022-00447-8
Grebenyuk S, Abdel Fattah AR, Kumar M, et al., 2023, Large-scale perfused tissues via synthetic 3D soft microfluidics. Nat Commun, 14(1): 193. https://doi.org/10.1038/s41467-022-35619-1
Fleischer S, Tavakol DN, Vunjak-Novakovic G, 2020, From arteries to capillaries: Approaches to engineering human vasculature. Adv Funct Mater, 30(37): 1910811. https://doi.org/10.1002/adfm.201910811
Li S, Jin J, Zhang C, et al., 2023, 3D bioprinting vascular networks in suspension baths. Appl Mater Today, 30: 101729. https://doi.org/10.1016/j.apmt.2022.101729
Seah T, Wong WL, Beh C, 2022, Vascularization strategies for bioprinting. Mater Today, 70: 638–642. https://doi.org/10.1016/j.matpr.2022.10.026
Yang Q, Lian Q, Xu F, 2017, Perspective: Fabrication of integrated organ-on-a-chip via bioprinting. Biomicrofluidics, 11(3): 031301. https://doi.org/10.1063/1.4982945
Zhang Q, Bosch-Rué È, Pérez RA, et al., 2021, Biofabrication of tissue engineering vascular systems. APL Bioeng, 5(2): 021507. https://doi.org/10.1063/5.0039628
Hedegaard CL, Mata A, 2020, Integrating self-assembly and biofabrication for the development of structures with enhanced complexity and hierarchical control. Biofabrication, 12(3): 032002. https://dx.doi.org/10.1088/1758-5090/ab84cb
Wu Y, Fortunato GM, Okesola BO, et al., 2021, An interfacial self-assembling bioink for the manufacturing of capillary-like structures with tuneable and anisotropic permeability. Biofabrication, 13(3): 035027. https://dx.doi.org/10.1088/1758-5090/abe4c3
Wu Y, Okesola BO, Xu J, et al., 2020, Disordered protein-graphene oxide co-assembly and supramolecular biofabrication of functional fluidic devices. Nat Commun, 11(1): 1182. https://doi.org/10.1038/s41467-020-14716-z
Ha D-H, Chae S, Lee JY, et al., 2021, Therapeutic effect of decellularized extracellular matrix-based hydrogel for radiation esophagitis by 3D printed esophageal stent. Biomaterials, 266: 120477. https://doi.org/10.1016/j.biomaterials.2020.120477
Chae S, Yong U, Park W, et al., 2023, 3D cell-printing of gradient multi-tissue interfaces for rotator cuff regeneration. Bioact Mater, 19: 611–625. https://doi.org/10.1016/j.bioactmat.2022.05.004s
Bliley JM, Shiwarski DJ, Feinberg AW, 2022, 3D-bioprinted human tissue and the path toward clinical translation. Sci Transl Med, 14(666): eabo7047. https://doi.org/10.1126/scitranslmed.abo7047
Zhang Y, Kumar P, Lv S, et al., 2021, Recent advances in 3D bioprinting of vascularized tissues. Mater Design, 199: 109398. https://doi.org/10.1016/j.matdes.2020.109398
Song HHG, Rumma RT, Ozaki CK, et al., 2018, Vascular tissue engineering: progress, challenges, and clinical promise. Cell Stem Cell, 22(3): 340–354. https://doi.org/10.1016/j.stem.2018.02.009
Chae S, Cho D-W, 2022, Three-dimensional bioprinting with decellularized extracellular matrix-based bioinks in translational regenerative medicine. MRS Bull, 47(1): 70–79. https://doi.org/10.1557/s43577-021-00260-8
Li J, Wu C, Chu PK, et al., 2020, 3D printing of hydrogels: Rational design strategies and emerging biomedical applications. Mater Sci Eng R Rep, 140: 100543. https://doi.org/10.1016/j.mser.2020.100543
Chae S, Cho D-W, 2023, Biomaterial-based 3D bioprinting strategy for orthopedic tissue engineering. Acta Biomater, 156: 4–20. https://doi.org/10.1016/j.actbio.2022.08.004
Daly AC, Prendergast ME, Hughes AJ, et al., 2021, Bioprinting for the biologist. Cell, 184(1): 18–32. https://doi.org/10.1016/j.cell.2020.12.002
Jang J, Park H-J, Kim S-W, et al., 2017, 3D printed complex tissue construct using stem cell-laden decellularized extracellular matrix bioinks for cardiac repair. Biomaterials, 112: 264–274. https://doi.org/10.1016/j.biomaterials.2016.10.026
Maiullari F, Costantini M, Milan M, et al., 2018, A multi-cellular 3D bioprinting approach for vascularized heart tissue engineering based on HUVECs and iPSC-derived cardiomyocytes. Sci Rep, 8(1): 13532. https://doi.org/10.1038/s41598-018-31848-x
Homan KA, Kolesky DB, Skylar-Scott MA, et al., 2016, Bioprinting of 3D convoluted renal proximal tubules on perfusable chips. Sci Rep, 6(1): 34845. https://doi.org/10.1038/srep34845
Kolesky DB, Truby RL, Gladman AS, et al., 2014, 3D bioprinting of vascularized, heterogeneous cell-laden tissue constructs. Adv Mater, 26(19): 3124–3130. https://doi.org/10.1002/adma.201305506
Miller JS, Stevens KR, Yang MT, et al., 2012, Rapid casting of patterned vascular networks for perfusable engineered three-dimensional tissues. Nat Mater, 11(9): 768–774. https://doi.org/10.1038/nmat3357
Skylar-Scott MA, Uzel SGM, Nam LL, et al., 2019, Biomanufacturing of organ-specific tissues with high cellular density and embedded vascular channels. Sci Adv, 5(9): eaaw2459. https://doi.org/10.1126/sciadv.aaw2459
Ouyang L, Armstrong JPK, Chen Q, et al., 2020, Void-free 3D bioprinting for in situ endothelialization and microfluidic perfusion. Adv Funct Mater, 30(1): 1908349. https://doi.org/10.1002/adfm.201908349
Lee A, Hudson AR, Shiwarski DJ, et al., 2019, 3D bioprinting of collagen to rebuild components of the human heart. Science, 365(6452): 482–487. https://doi.org/10.1126/science.aav9051
Noor N, Shapira A, Edri R, et al., 2019, 3D printing of personalized thick and perfusable cardiac patches and hearts. Adv Sci, 6(11): 1900344. https://doi.org/10.1002/advs.201900344
Choi Y-J, Jun Y-J, Kim DY, et al., 2019, A 3D cell printed muscle construct with tissue-derived bioink for the treatment of volumetric muscle loss. Biomaterials, 206: 160–169. https://doi.org/10.1016/j.biomaterials.2019.03.036
Zhang W, Feng C, Yang G, et al., 2017, 3D-printed scaffolds with synergistic effect of hollow-pipe structure and bioactive ions for vascularized bone regeneration. Biomaterials, 135: 85–95. https://doi.org/10.1016/j.biomaterials.2017.05.005
Gao Q, Liu Z, Lin Z, et al., 2017, 3D bioprinting of vessel-like structures with multilevel fluidic channels. ACS Biomater Sci Eng, 3(3): 399–408. https://doi.org/10.1021/acsbiomaterials.6b00643
Gao Q, He Y, Fu J-z, et al., 2015, Coaxial nozzle-assisted 3D bioprinting with built-in microchannels for nutrients delivery. Biomaterials, 61: 203–215. https://doi.org/10.1016/j.biomaterials.2015.05.031
Jia W, Gungor-Ozkerim PS, Zhang YS, et al., 2016, Direct 3D bioprinting of perfusable vascular constructs using a blend bioink. Biomaterials, 106: 58–68. https://doi.org/10.1016/j.biomaterials.2016.07.038
Gao G, Park W, Kim BS, et al., 2021, Construction of a novel in vitro atherosclerotic model from geometry-tunable artery equivalents engineered via in-bath coaxial cell printing. Adv Funct Mater, 31(10): 2008878. https://doi.org/10.1002/adfm.202008878
Xiang Y, Miller K, Guan J, et al., 2022, 3D bioprinting of complex tissues in vitro: State-of-the-art and future perspectives. Arch Toxicol, 96(3): 691–710. https://doi.org/10.1007/s00204-021-03212-y
Kolesky DB, Homan KA, Skylar-Scott MA, et al., 2016, Three-dimensional bioprinting of thick vascularized tissues. Proc Natl Acad Sci, 113(12): 3179–3184. https://doi.org/10.1073/pnas.1521342113
Gao G, Park JY, Kim BS, et al., 2018, Coaxial cell printing of freestanding, perfusable, and functional in vitro vascular models for recapitulation of native vascular endothelium pathophysiology. Adv Healthc Mater, 7(23): 1801102. https://doi.org/10.1002/adhm.201801102
Son J, Hong SJ, Lim JW, et al., 2021, Engineering tissue-specific, multiscale microvasculature with a capillary network for prevascularized tissue. Small Methods, 5(10): 2100632. https://doi.org/10.1002/smtd.202100632
Ma L, Wu Y, Li Y, et al., 2020, Current advances on 3D-bioprinted liver tissue models. Adv Healthc Mater, 9(24): 2001517. https://doi.org/10.1002/adhm.202001517
Lee H, Chae S, Kim JY, et al., 2019, Cell-printed 3D liver-on-a-chip possessing a liver microenvironment and biliary system. Biofabrication, 11(2): 025001. https://dx.doi.org/10.1088/1758-5090/aaf9fa
Liu X, Wang X, Zhang L, et al., 2021, 3D liver tissue model with branched vascular networks by multimaterial bioprinting. Adv Healthc Mater, 10(23): 2101405. https://doi.org/10.1002/adhm.202101405
Taymour R, Chicaiza-Cabezas NA, Gelinsky M, et al., 2022, Core–shell bioprinting of vascularized in vitro liver sinusoid models. Biofabrication, 14(4): 045019. https://dx.doi.org/10.1088/1758-5090/ac9019
Kang D, Hong G, An S, et al., 2020, Bioprinting of multiscaled hepatic lobules within a highly vascularized construct. Small, 16(13): 1905505. https://doi.org/10.1002/smll.201905505
Lee H, Kim J, Choi Y, et al., 2020, Application of gelatin bioinks and cell-printing technology to enhance cell delivery capability for 3D liver fibrosis-on-a-chip development. ACS Biomater Sci Eng, 6(4): 2469–2477. https://doi.org/10.1021/acsbiomaterials.9b01735
Sakolish CM, Philip B, Mahler GJ, 2019, A human proximal tubule-on-a-chip to study renal disease and toxicity. Biomicrofluidics, 13(1): 014107. https://doi.org/10.1063/1.5083138
Schutgens F, Rookmaaker MB, Margaritis T, et al., 2019, Tubuloids derived from human adult kidney and urine for personalized disease modeling. Nat Biotechnol, 37(3): 303–313. https://doi.org/10.1038/s41587-019-0048-8
Lin NYC, Homan KA, Robinson SS, et al., 2019, Renal reabsorption in 3D vascularized proximal tubule models. Proc Natl Acad Sci, 116(12): 5399–5404. https://doi.org/10.1073/pnas.1815208116
Singh NK, Han W, Nam SA, et al., 2020, Three-dimensional cell-printing of advanced renal tubular tissue analogue. Biomaterials, 232: 119734. https://doi.org/10.1016/j.biomaterials.2019.119734
Yoon J, Singh NK, Jang J, et al., 2022, 3D bioprinted in vitro secondary hyperoxaluria model by mimicking intestinal-oxalate-malabsorption-related kidney stone disease. Appl Phys Rev, 9(4): 041408. https://doi.org/10.1063/5.0087345
Jung M, Ghamrawi S, Du EY, et al., 2022, Advances in 3D bioprinting for cancer biology and precision medicine: From matrix design to application. Adv Healthc Mater, 11(24): 2200690. https://doi.org/10.1002/adhm.202200690
Kang Y, Datta P, Shanmughapriya S, et al., 2020, 3D bioprinting of tumor models for cancer research. ACS Appl Biomater, 3(9): 5552–5573. https://doi.org/10.1021/acsabm.0c00791
Neufeld L, Yeini E, Pozzi S, et al., 2022, 3D bioprinted cancer models: From basic biology to drug development. Nat Rev Cancer, 22(12): 679–692. https://doi.org/10.1038/s41568-022-00514-w
Yi H-G, Jeong YH, Kim Y, et al., 2019, A bioprinted human-glioblastoma-on-a-chip for the identification of patient-specific responses to chemoradiotherapy. Nat Biomed Eng, 3(7): 509–519. https://doi.org/10.1038/s41551-019-0363-x
Neufeld L, Yeini E, Reisman N, et al., 2021, Microengineered perfusable 3D-bioprinted glioblastoma model for in vivo mimicry of tumor microenvironment. Sci Adv, 7(34): eabi9119. https://doi.org/10.1126/sciadv.abi9119
Ozturk MS, Lee VK, Zou H, et al., 2020, High-resolution tomographic analysis of in vitro 3D glioblastoma tumor model under long-term drug treatment. Sci Adv, 6(10): eaay7513. https://doi.org/10.1126/sciadv.aay7513
Meng F, Meyer CM, Joung D, et al., 2019, 3D bioprinted in vitro metastatic models via reconstruction of tumor microenvironments. Adv Mater, 31(10): 1806899. https://doi.org/10.1002/adma.201806899
Kim BS, Cho W-W, Gao G, et al., 2021, Construction of tissue-level cancer-vascular model with high-precision position control via in situ 3D cell printing. Small Methods, 5(7): 2100072. https://doi.org/10.1002/smtd.202100072
Obinu A, Gavini E, Rassu G, et al., 2018, Lymph node metastases: Importance of detection and treatment strategies. Expert Opin Drug Deliv, 15(5): 459–467. https://doi.org/10.1080/17425247.2018.1446937
Cao X, Ashfaq R, Cheng F, et al., 2019, A tumor-on-a-chip system with bioprinted blood and lymphatic vessel pair. Adv Funct Mater, 29(31): 1807173. https://doi.org/10.1002/adfm.201807173
Cho W-W, Ahn M, Kim BS, et al., 2022, Blood-lymphatic integrated system with heterogeneous melanoma spheroids via in-bath three-dimensional bioprinting for modelling of combinational targeted therapy. Adv Sci, 9(29): 2202093. https://doi.org/10.1002/advs.202202093
Chiesa I, De Maria C, Lapomarda A, et al., 2020, Endothelial cells support osteogenesis in an in vitro vascularized bone model developed by 3D bioprinting. Biofabrication, 12(2): 025013. https://dx.doi.org/10.1088/1758-5090/ab6a1d
Kim BS, Gao G, Kim JY, et al., 2019, 3D cell printing of perfusable vascularized human skin equivalent composed of epidermis, dermis, and hypodermis for better structural recapitulation of native skin. Adv Healthc Mater, 8(7): 1801019. https://doi.org/10.1002/adhm.201801019
Chae S, Kim J, Yi H-G, et al., 2022, 3D bioprinting of an in vitro model of a biomimetic urinary bladder with a contract-release system. Micromachines, 13(2): 277. https://doi.org/10.3390/mi13020277
Zhao Z, Chen X, Dowbaj AM, et al., 2022, Organoids. Nat Rev Methods Primers, 2(1): 94. https://doi.org/10.1038/s43586-022-00174-y
Chae S, Lee S-S, Choi Y-J, et al., 2021, 3D cell-printing of biocompatible and functional meniscus constructs using meniscus‐derived bioink. Biomaterials, 267: 120466. https://doi.org/10.1016/j.biomaterials.2020.120466
Kim BS, Das S, Jang J, et al., 2020, Decellularized extracellular matrix-based bioinks for engineering tissue-and organ-specific microenvironments. Chem Rev, 120(19): 10608–10661. https://doi.org/10.1021/acs.chemrev.9b00808
Chae S, Sun Y, Choi Y-J, et al., 2021, 3D cell-printing of tendon-bone interface using tissue-derived extracellular matrix bioinks for chronic rotator cuff repair. Biofabrication, 13(3): 035005. https://dx.doi.org/10.1088/1758-5090/abd159
Chae S, Choi Y-J, Cho D-W, 2022, Mechanically and biologically promoted cell-laden constructs generated using tissue-specific bioinks for tendon/ligament tissue engineering applications. Biofabrication, 14(2): 025013. https://dx.doi.org/10.1088/1758-5090/ac4fb6