Droplet-based bioprinting for fabrication of tumor spheroids
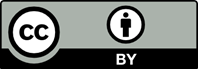
Cancer is now one of the leading causes of mortality worldwide, and the cancer treatment development is still slow due to the lack of efficient in vitro tumor models for studying tumorigenesis and facilitating drug development. Multicellular tumor spheroids can recapitulate the critical properties of tumors in vivo, including spatial organization, physiological responses, and metabolism, and are considered powerful platform for disease study and drug screening. Although several spheroid fabrication methods have been developed, most of them result in uncontrolled cell aggregations, yielding spheroids of variable size and function. Droplet-based bioprinting is capable of depositing cells in spatiotemporal manner so as to control the composition and distribution of printed biological constructs, thereby facilitating high-throughput fabrication of complicated and reproducible tumor spheroids. In this review, we introduce the progress of droplet-based bioprinting technology for the fabrication of tumor spheroids. First, different droplet-based bioprinting technologies are compared in terms of their strengths and shortcomings, which should be taken into account while fabricating tumor spheroids. Second, the latest advances in modeling distinct types of cancers and the enabled applications with tumor spheroids are summarized. Finally, we discuss the challenges and potentials revolving around the advances of bioprinting technology, improvement of spheroid quality, and integration of different technologies.
- Xia C, Dong X, Li H, et al. Cancer statistics in China and United States, 2022: Profiles, trends, and determinants. Chin Med J (Engl). 2022;135(5):584-590. doi: 10.1097/CM9.0000000000002108
- Siegel RL, Miller KD, Fuchs HE, Jemal A. Cancer statistics, 2022. CA Cancer J Clin. 2022;72(1):7-33. doi: 10.3322/caac.21708
- Ferlay J, Colombet M, Soerjomataram I, et al. Cancer statistics for the year 2020: An overview. Int J Cancer. 2021; 149(4):778-789. doi: 10.1002/ijc.33588
- Zugazagoitia J, Guedes C, Ponce S, Ferrer I, Molina-Pinelo S, Paz-Ares L. Current challenges in cancer treatment. Clin Ther. 2016;38(7):1551-1566. doi: 10.1016/j.clinthera.2016.03.026
- Zhuang P, Chiang YH, Fernanda MS, He M. Using spheroids as building blocks towards 3D bioprinting of tumor microenvironment. Int J Bioprint. 2021;7(4):1-26. doi: 10.18063/ijb.v7i4.444
- Jo Y, Choi N, Kim K, Koo H-J, Choi J, Kim HN. Chemoresistance of cancer cells: Requirements of tumor microenvironment-mimicking in vitro models in anti-cancer drug development. Theranostics. 2018;8(19):5259- 5275. doi: 10.7150/thno.29098
- Chramiec A, 2022; A novel human multi-tissue system for preclinical drug evaluation and recapitulation of metastasis, thesis, ProQuest Dissertations & Theses Global, Columbia University, 28963406.
- Dhiman HK, Ray AR,Panda AK. Three-dimensional chitosan scaffold-based MCF-7 cell culture for the determination of the cytotoxicity of tamoxifen. Biomaterials. 2005;26(9):979-986. doi: 10.1016/j.biomaterials.2004.04.012
- Imamura Y, Mukohara T, Shimono Y, et al. Comparison of 2D- and 3D-culture models as drug-testing platforms in breast cancer. Oncol Rep. 2015; 33(4):1837-1843. doi: 10.3892/or.2015.3767
- Hoarau-Vechot J, Rafii A, Touboul C, Pasquier J. Halfway between 2D and animal models: Are 3D cultures the ideal tool to study cancer-microenvironment interactions? Int J Mol Sci. 2018;19(1):181. doi: 10.3390/ijms19010181
- Kapalczynska M, Kolenda T, Przybyla W, et al. 2D and 3D cell cultures - a comparison of different types of cancer cell cultures. Arch Med Sci. 2018;14(4):910-919. doi: 10.5114/aoms.2016.63743
- Lin RZ, Chou LF, Chien CC, Chang H-Y. Dynamic analysis of hepatoma spheroid formation: Roles of E-cadherin and beta1-integrin. Cell Tissue Res. 2006;324(3):411-422. doi: 10.1007/s00441-005-0148-2
- Nath S, Devi GR. Three-dimensional culture systems in cancer research: Focus on tumor spheroid model. Pharmacol Ther. 2016;163: 94-108. doi: 10.1016/j.pharmthera.2016.03.013
- Xu T, Kincaid H, Atala A, Yoo J. High-throughput production of single-cell microparticles using an inkjet printing technology. J Manuf Sci E-T Asme. 2008;130(2):021017. doi: 10.1115/1.2903064
- Gao G, Yonezawa T, Hubbell K, Dai G, Cui X. Inkjet-bioprinted acrylated peptides and PEG hydrogel with human mesenchymal stem cells promote robust bone and cartilage formation with minimal printhead clogging. Biotechnol J. 2015;10(10):1568-1577. doi: 10.1002/biot.201400635
- Xu T, Gregory CA, Molnar P, et al. Viability and electrophysiology of neural cell structures generated by the inkjet printing method. Biomaterials. 2006;27(19):3580- 3588. doi: 10.1016/j.biomaterials.2006.01.048
- Campbell A, Philipovskiy A, Heydarian R, Varela-Ramirez A. 2D and 3D thermally bioprinted human MCF-7 breast cancer cells: A promising model for drug discovery. J Clin Oncol. 2019;37(15):2605. doi: 10.1200/JCO.2019.37.15_suppl.2605
- Xu T, Zhao W, Zhu JM, Albanna MZ, Yoo JJ, Atala A. Complex heterogeneous tissue constructs containing multiple cell types prepared by inkjet printing technology. Biomaterials. 2013;34(1):130-139. doi: 10.1016/j.biomaterials.2012.09.035
- Xu T, Jin J, Gregory C, J Hickman JJJ, Boland T. Inkjet printing of viable mammalian cells. Biomaterials. 2005;26(1):93-99. doi: 10.1016/j.biomaterials.2004.04.011
- Xu C, Chai W, Huang Y, Markwald RR. Scaffold-free inkjet printing of three-dimensional zigzag cellular tubes. Biotechnol Bioeng. 2012;109(12):3152-3160. doi: 10.1002/bit.24591
- Cheng E, Yu H, Ahmadi A, Cheung KC. Investigation of the hydrodynamic response of cells in drop on demand piezoelectric inkjet nozzles. Biofabrication. 2016;8(1): 015008. doi: 10.1088/1758-5090/8/1/015008
- Wijshoff H. The dynamics of the piezo inkjet printhead operation. Phys Rep. 2010;491(4-5):77-177. doi: 10.1016/j.physrep.2010.03.003
- Shi J, Wu B, Li S, Song J, Song Band Lu WF. Shear stress analysis and its effects on cell viability and cell proliferation in drop-on-demand bioprinting. Biomed Phys Eng Expr. 2018;4(4):045028. doi: 10.1088/2057-1976/aac946
- Kamisuki S, Hagata T, Tezuka C, Nose Y, Fujii M, Atobe M. A low power, small, electrostatically driven driven commercial inkjet head. Micro Electro Mechanical Systems - Ieee Eleventh Annual International Workshop Proceedings. 1998;1998;63-68.
- Nishiyama Y, Nakamura M, Henmi C, et al. Development of a three-dimensional bioprinter: construction of cell supporting structures using hydrogel and State-Of-The-Art inkjet technology. J Biomech Eng. 2009;131(3):035001. doi: 10.1115/1.3002759
- Gao D, Yao D, Leist SK, Fei Y, Zhou J. Mechanisms and modeling of electrohydrodynamic phenomena. Int J Bioprint. 2019;5(1):166. doi: 10.18063/ijb.v5i1.166
- Eagles PA, Qureshi AN, Jayasinghe SN. Electrohydrodynamic jetting of mouse neuronal cells. Biochem J. 2006;394(2): 375-378. doi: 10.1042/BJ20051838
- Workman VL, Tezera LB, Elkington PT, Jayasinghe SN. Controlled generation of microspheres incorporating extracellular matrix fibrils for three-dimensional cell culture. Adv Funct Mater. 2014;24(18):2648-2657. doi: 10.1002/adfm.201303891
- Gasperini L, Maniglio D, Motta A, Migliaresi C. An electrohydrodynamic bioprinter for alginate hydrogels containing living cells. Tissue Eng Part C Methods. 2015;21(2):123-132. doi: 10.1089/ten.TEC.2014.0149
- Chen CH, Saville DA, Aksay IA. Electrohydrodynamic “drop-and-place” particle deployment. Appl Phys Lett. 2006;88(15):154104. doi: 10.1063/1.2191733
- Poellmann MJ, Barton KL, Mishra S, Johnson AJW. Patterned hydrogel substrates for cell culture with electrohydrodynamic jet printing. Macromol Biosci. 2011;11(9):1164-1168. doi: 10.1002/mabi.201100004
- Gudapati H, Dey M, Ozbolat I. A comprehensive review on droplet-based bioprinting: Past, present and future. Biomaterials. 2016;102: 20-42. doi: 10.1016/j.biomaterials.2016.06.012
- Onses MS, Sutanto E, Ferreira PM, Alleyne AG, Rogers JA. Mechanisms, capabilities, and applications of high-resolution electrohydrodynamic jet printing. Small. 2015;11(34):4237-4266. doi: 10.1002/smll.201500593
- Kim HS, Lee DY, Park JH, Hwang JH, Jung HI. Optimization of electrohydrodynamic writing technique to print collagen. Exp Tech. 2007;31(4):15-19. doi: 10.1111/j.1747-1567.2007.00154.x
- Chen X, O’Mahony AP,Barber T. The assessment of average cell number inside in-flight 3D printed droplets in microvalve-based bioprinting. J Appl Phys. 2022;131(22):224701. doi: 10.1063/5.0096468
- Ng WL, Lee JM, Yeong WY, Naing MW. Microvalve-based bioprinting process, bioinks and applications. Biomater Sci. 2017;5(4):632-647. doi: 10.1039/c6bm00861e
- Faulkner-Jones A, Fyfe C, Cornelissen DJ, et al. Bioprinting of human pluripotent stem cells and their directed differentiation into hepatocyte-like cells for the generation of mini-livers in 3D. Biofabrication. 2015;7(4):044102. doi: 10.1088/1758-5090/7/4/044102
- Chen X, O’Mahony AP, Barber T. Experimental study of the stable droplet formation process during micro-valve-based three-dimensional bioprinting. Phys Fluids. 2023;35(1):011903. doi: 10.1063/5.0129985
- Xu F, Moon SJ, Emre AE, et al. A droplet-based building block approach for bladder smooth muscle cell (SMC) proliferation. Biofabrication. 2010;2(1):014105. doi: 10.1088/1758-5082/2/1/014105
- Xu F, Celli J, Rizvi I, Moon Sangjun, Hasan T, Demirci U. A three-dimensional in vitro ovarian cancer coculture model using a high-throughput cell patterning platform. Biotechnol J. 2011;6(2):204-212. doi: 10.1002/biot.201000340
- Demirci U, Montesano G. Cell encapsulating droplet vitrification. Lab Chip. 2007;7(11):1428-1433. doi: 10.1039/b705809h
- Faulkner-Jones A, Greenhough S, King JA, Gardner J, Courtney A, Shu W. Development of a valve-based cell printer for the formation of human embryonic stem cell spheroid aggregates. Biofabrication. 2013;5(1):015013. doi: 10.1088/1758-5082/5/1/015013
- Lee W, Debasitis JC, Lee VK, et al. Multi-layered culture of human skin fibroblasts and keratinocytes through three-dimensional freeform fabrication. Biomaterials. 2009;30(8):1587-1595. doi: 10.1016/j.biomaterials.2008.12.009
- Moon S, Hasan SK, Song YS, et al. Layer by layer three-dimensional tissue epitaxy by cell-laden hydrogel droplets. Tissue Eng Part C-Me. 2010;16(1):157-166. doi: 10.1089/ten.tec.2009.0179
- Jentsch S, Nasehi R, Kuckelkorn C, Gundert B, Aveic S, Fischer H. Multiscale 3D bioprinting by nozzle-free acoustic droplet ejection. Small Methods. 2021;5(6):2000971. doi: 10.1002/smtd.202000971
- Chen K, Jiang E, Wei X, et al. The acoustic droplet printing of functional tumor microenvironments. Lab Chip. 2021;21(8):1604-1612. doi: 10.1039/d1lc00003a
- Hadimioglu B, Stearns R, Ellson R. Moving liquids with sound: The physics of acoustic droplet ejection for robust laboratory automation in life sciences. J Lab Autom. 2016;21(1):4-18. doi: 10.1177/2211068215615096
- Elrod SA, Hadimioglu B, Khuri-Yakub BT, et al. Nozzleless droplet formation with focused acoustic beams. J Appl Phys. 1989;65(9):3441-3447. doi: 10.1063/1.342663
- Demirci U, Montesano G. Single cell epitaxy by acoustic picolitre droplets. Lab Chip. 2007;7(9):1139-1145. doi: 10.1039/b704965j
- Sriphutkiat Y, Kasetsirikul S, Ketpun D, Zhou Y. Cell alignment and accumulation using acoustic nozzle for bioprinting. Sci Rep. 2019;9(1):17774. doi: 10.1038/s41598-019-54330-8
- Chen Y, Liu X, Zhang C, Zhao Y. Enhancing and suppressing effects of an inner droplet on deformation of a double emulsion droplet under shear. Lab Chip. 2015;15(5):1255-1261. doi: 10.1039/c4lc01231c
- Zhang S, Li G, Man J, et al. Fabrication of microspheres from high-viscosity bioink using a novel microfluidic-based 3D bioprinting nozzle. Micromachine Basel. 2020; 11(7):681. doi: 10.3390/mi11070681
- Mesquita CRS, Charelli LE, Baptista LS, Naveira-Cotta CP, Balbino TA. 2021;Continuous-mode encapsulation of human stem cell spheroids using droplet-based glass-capillary microfluidic device for 3D bioprinting technology. Biochem Eng J. 174: 8. doi: 10.1016/j.bej.2021.108122
- Zhang P, Abate AR. High-definition single-cell printing: cell-by-cell fabrication of biological structures. Adv Mater. 2020;32(52):2005346. doi: 10.1002/adma.202005346
- Gunti S, Hoke ATK, Vu KP, London NR. Organoid and spheroid tumor models: Techniques and applications. Cancers. 2021;13(4):874. doi: 10.3390/cancers13040874
- Fennema E, Rivron N, Rouwkema J, van Blitterswijk C, de Boer J. Spheroid culture as a tool for creating 3D complex tissues. Trends Biotechnol. 2013;31(2):108-115. doi: 10.1016/j.tibtech.2012.12.003
- Baghban R, Roshangar L, Jahanban-Esfahlan R, et al. Tumor microenvironment complexity and therapeutic implications at a glance. Cell Commun Signal. 2020;18(1):59. doi: 10.1186/s12964-020-0530-4
- Vinci M, Gowan S, Boxall F, et al. Advances in establishment and analysis of three-dimensional tumor spheroid-based functional assays for target validation and drug evaluation. Bmc Biology. 2012;10: 29. doi: 10.1186/1741-7007-10-29
- Qi X, Prokhorova AV, Mezentsev AV, et al. Comparison of EMT-related and multi-drug resistant gene expression, extracellular matrix production, and drug sensitivity in NSCLC spheroids generated by scaffold-free and scaffold-based methods. Int J Mol Sci. 2022;23(21):13306. doi: 10.3390/ijms232113306
- Ho WJ, Pham EA, Kim JW, et al. Incorporation of multicellular spheroids into 3-D polymeric scaffolds provides an improved tumor model for screening anticancer drugs. Cancer Sci. 2010;101(12):2637-2643. doi: 10.1111/j.1349-7006.2010.01723.x
- Qiu P, Qu X, Brackett DJ, Lerner MR, Li D, Mao C. Silica-based branched hollow microfibers as a biomimetic extracellular matrix for promoting tumor cell growth in vitro and in vivo. Adv Mater. 2013;25(17):2492-2496. doi: 10.1002/adma.201204472
- Ong SM, Zhao Z, Arooz T, et al. Engineering a scaffold-free 3D tumor model for in vitro drug penetration studies. Biomaterials. 2010;31(6):1180-1190. doi: 10.1016/j.biomaterials.2009.10.049
- Oliveira MB, Neto AI, Correia CR, Rial-Hermida MI, Alvarez-Lorenzo C, Mano JF. Superhydrophobic chips for cell spheroids high-throughput generation and drug screening. ACS Appl Mater Interfaces. 2014;6(12):9488-9495. doi: 10.1021/am5018607
- Franchi-Mendes T, Lopes N, Brito C. Heterotypic tumor spheroids in agitation-based cultures: A scaffold-free cell model that sustains long-term survival of endothelial cells. Front Bioeng Biotechnol. 2021;9: 649949. doi: 10.3389/fbioe.2021.649949
- Tu TY, Wang Z, Bai J, et al. Rapid prototyping of concave microwells for the formation of 3D multicellular cancer aggregates for drug screening. Adv Healthc Mater. 2014;3(4):609-616. doi: 10.1002/adhm.201300151
- Kim JA, Choi JH, Kim M, et al. High-throughput generation of spheroids using magnetic nanoparticles for three-dimensional cell culture. Biomaterials. 2013;34(34):8555-8563. doi: 10.1016/j.biomaterials.2013.07.056
- Bowser DA, Moore MJ. 2019;Biofabrication of neural microphysiological systems using magnetic spheroid bioprinting. Biofabrication. 12(1):015002. doi: 10.1088/1758-5090/ab41b4
- Chen K, Wu M, Guo F, et al. Rapid formation of size-controllable multicellular spheroids via 3D acoustic tweezers. Lab Chip. 2016;16(14):2636-2643. doi: 10.1039/c6lc00444j
- Rasouli R, Tabrizian M. Rapid formation of multicellular spheroids in boundary-driven acoustic microstreams. Small. 2021;17(39):2101931. doi: 10.1002/smll.202101931
- Sebastian A, Buckle AM, Markx GH. Tissue engineering with electric fields: Immobilization of mammalian cells in multilayer aggregates using dielectrophoresis. Biotechnol Bioeng. 2007;98(3):694-700. doi: 10.1002/bit.21416
- Maris JM, Matthay KK. Molecular biology of neuroblastoma. J Clin Oncol. 1999;17(7):2264. doi: 10.1200/jco.1999.17.7.2264
- Bhoopathi P, Pradhan AK, Bacolod MD, et al. Regulation of neuroblastoma migration, invasion, and in vivo metastasis by genetic and pharmacological manipulation of MDA-9/ Syntenin. Oncogene. 2019;38(41):6781-6793. doi: 10.1038/s41388-019-0920-5
- Ducker M, Millar V, Ebner D, Szele FG. A semi-automated and scalable 3D spheroid assay to study neuroblast migration. Stem Cell Rep. 2020;15(3):789-802. doi: 10.1016/j.stemcr.2020.07.012
- Utama RH, Atapattu L, O’Mahony AP, et al. A 3D bioprinter specifically designed for the high-throughput production of matrix-embedded multicellular spheroids. iScience. 2020;23(10):101621. doi: 10.1016/j.isci.2020.101621
- Martin-Belmonte F, Perez-Moreno M. Epithelial cell polarity, stem cells and cancer. Nat Rev Cancer. 2011;12(1):23-38. doi: 10.1038/nrc3169
- Trondle K, Rizzo L, Pichler R, et al. Scalable fabrication of renal spheroids and nephron-like tubules by bioprinting and controlled self-assembly of epithelial cells. Biofabrication. 2021;13(3):035019. doi: 10.1088/1758-5090/abe185
- Caruso S, Calatayud AL, Pilet J, et al. Analysis of liver cancer cell lines identifies agents with likely efficacy against hepatocellular carcinoma and markers of response. Gastroenterology. 2019;157(3):760-776. doi: 10.1053/j.gastro.2019.05.001
- Chen Y, Sun W, Kang L, et al. Microfluidic co-culture of liver tumor spheroids with stellate cells for the investigation of drug resistance and intercellular interactions. Analyst. 2019;144(14):4233-4240. doi: 10.1039/c9an00612e
- Khomich O, Ivanov AV, Bartosch B. Metabolic hallmarks of hepatic stellate cells in liver fibrosis. Cells. 2020;9(1):24. doi: 10.3390/cells9010024
- Hong G, Kim J, Oh H, et al. Production of multiple cell-laden microtissue spheroids with a biomimetic hepatic-lobule-like structure. Adv Mater. 2021;33(36):2102624. doi: 10.1002/adma.202102624
- Zhang P, Li X, Chen JY, Abate AR. Controlled fabrication of functional liver spheroids with microfluidic flow cytometric printing. Biofabrication. 2022;14(4):045011. doi: 10.1088/1758-5090/ac8622
- Trounson A. The production and directed differentiation of human embryonic stem cells. Endocr Rev. 2006;27(2):208-219. doi: 10.1210/er.2005-0016
- Sun YS, Zhao Z, Yang ZN, et al. Risk factors and preventions of breast cancer. Int J Biol Sci. 2017;13(11):1387-1397. doi: 10.7150/ijbs.21635
- Polyak K. Breast cancer: origins and evolution. J Clin Invest. 2007;117(11):3155-3163. doi: 10.1172/JCI33295
- Markovitz-Bishitz Y, Tauber Y, Afrimzon E, et al. A polymer microstructure array for the formation, culturing, and high throughput drug screening of breast cancer spheroids. Biomaterials. 2010;31(32):8436-8444. doi: 10.1016/j.biomaterials.2010.07.050
- Ling K, Huang G, Liu J, et al. Bioprinting-based high-throughput fabrication of three-dimensional MCF-7 human breast cancer cellular spheroids. Engineering. 2015;1(2):269- 274. doi: 10.15302/j-eng-2015062
- Johnson PA, Menegatti S, Chambers AC, et al. A rapid high throughput bioprinted colorectal cancer spheroid platform for in vitro drug- and radiation-response. Biofabrication. 2022;15(1):014103. doi: 10.1088/1758-5090/ac999f
- Wang H, Tian T, Zhang J. Tumor-associated macrophages (TAMs) in colorectal cancer (CRC):From mechanism to therapy and prognosis. Int J Mol Sci. 2021;22(16):8470. doi: 10.3390/ijms22168470
- Jiang L, Ji N, Zhou Y, et al. CAL 27 is an oral adenosquamous carcinoma cell line. Oral Oncol. 2009;45(11):204-207. doi: 10.1016/j.oraloncology.2009.06.001
- Tanaka T, Ishigamori R. Understanding carcinogenesis for fighting oral cancer. J Oncol. 2011;2011: 603740. doi: 10.1155/2011/603740
- Kronemberger GS, Miranda G, Tavares RSN, Montenegro B. Recapitulating tumorigenesis in vitro: opportunities and challenges of 3D bioprinting. Front Bioeng Biotechnol. 2021;9: 682498. doi: 10.3389/fbioe.2021.682498
- Ota H, Miki N. Microfluidic experimental platform for producing size-controlled three-dimensional spheroids. Sens Actuators, A. 2011;169(2):266-273. doi: 10.1016/j.sna.2011.03.051
- Dornhof J, Zieger V, Kieninger J, et al. Bioprinting-based automated deposition of single cancer cell spheroids into oxygen sensor microelectrode wells. Lab Chip. 2022;22(22):4369-4381. doi: 10.1039/d2lc00705c
- Chang HN, Moo-Young M. Estimation of oxygen penetration depth in immobilized cells. Appl Microbiol Biotechnol. 1988;29(2):107-112. doi: 10.1007/BF00939293
- Rouwkema J, Rivron NC,van Blitterswijk CA. Vascularization in tissue engineering. Trends Biotechnol. 2008;26(8): 434-441. doi: 10.1016/j.tibtech.2008.04.009
- Lee VK, Guohao D, Hongyan Z, Yoo S. Generation of 3-D glioblastoma-vascular niche using 3-D bioprinting. Proceedings of the 41st Annual Northeast Biomedical Engineering Conference (NEBEC). 2015;1-2.
- Trondle K, Koch F, Finkenzeller G, et al. Bioprinting of high cell-density constructs leads to controlled lumen formation with self-assembly of endothelial cells. J Tissue Eng Regen Med. 2019;13(10):1883-1895. doi: 10.1002/term.2939
- Chambers AF, Groom AC, MacDonald IC. Dissemination and growth of cancer cells in metastatic sites. Nat Rev Cancer. 2002;2(8):563-572. doi: 10.1038/nrc865
- Yilmaz M, Christofori G, Lehembre F. Distinct mechanisms of tumor invasion and metastasis. Trends Mol Med. 2007;13(12):535-541. doi: 10.1016/j.molmed.2007.10.004
- Pennacchietti S, Michieli P, Galluzzo M, Mazzone M, Giordano S, Comoglio PM. Hypoxia promotes invasive growth by transcriptional activation of the met protooncogene. Cancer Cell. 2003;3(4):347-361. doi: 10.1016/S1535-6108(03)00085-0
- Chen H, Du L, Li J, et al. Modeling cancer metastasis using acoustically bio-printed patient-derived 3D tumor microtissues. J Mater Chem B. 2022;10(11): 1843-1852. doi: 10.1039/d1tb02789a
- Seftor RE, Hess AR, Seftor EA, et al. Tumor cell vasculogenic mimicry: From controversy to therapeutic promise. Am J Pathol. 2012;181(4):1115-1125. doi: 10.1016/j.ajpath.2012.07.013
- Lee JM, Choi JW, Ahrberg CD, et al. Generation of tumor spheroids using a droplet-based microfluidic device for photothermal therapy. Microsyst Nanoeng. 2020;6(1):52. doi: 10.1038/s41378-020-0167-x
- Yu L, Chen MC, Cheung KC. Droplet-based microfluidic system for multicellular tumor spheroid formation and anticancer drug testing. Lab Chip. 2010;10(18):2424-2432. doi: 10.1039/c004590j
- Shi J, Song J, Song B, Lu WF. Multi-objective optimization design through machine learning for Drop-on-Demand bioprinting. Engineering. 2019;5(3):586-593. doi: 10.1016/j.eng.2018.12.009
- Ota S, Horisaki R, Kawamura Y, et al. Ghost cytometry. Science. 2018;360(6394):1246-1251. doi: 10.1126/science.aan0096
- De Moor L, Merovci I, Baetens S, et al. High-throughput fabrication of vascularized spheroids for bioprinting. Biofabrication. 2018;10(3):035009. doi: 10.1088/1758-5090/aac7e6
- Souza TKF, Nucci MP, Mamani JB, et al. Image and motor behavior for monitoring tumor growth in C6 glioma model. PLoS ONE. 2018;13(7):e0201453. doi: 10.1371/journal.pone.0201453
- 109. Liu J, Li K, Liu B. Far-red/near-infrared conjugated polymer nanoparticles for long-term in situ monitoring of liver tumor growth. Adv Sci. 2015;2(5):1500008. doi: 10.1002/advs.201500008