Three-dimensional bioprinting for musculoskeletal regeneration and disease modeling
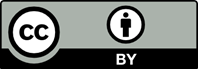
Musculoskeletal disease and injury are highly prevalent disorders that impose tremendous medical and socioeconomic burdens. Tissue engineering has attracted increasing attention as a promising technique of regenerative medicine to restore degenerative or damaged tissues and is used to produce functional disease models. As a revolutionary technology, three-dimensional (3D) bioprinting has demonstrated a considerable potential in enhancing the versatility of tissue engineering. 3D bioprinting allows for the rapid and accurate spatial patterning of cells, growth factors, and biomaterials to generate biomimetic tissue constructs. Meanwhile, 3D-bioprinted in vitro models also offer a viable option to enable precise pharmacological interventions in various diseases. This review provides an overview of 3D bioprinting methods and bioinks for therapeutic applications and describes their potential for musculoskeletal tissue regeneration. We also highlight the fabrication of 3D-bioprinted models for drug development targeting musculoskeletal disease. Finally, the existing challenges and future perspectives of 3D bioprinting for musculoskeletal regeneration and disease modeling are discussed.
- Khodabukus A, Guyer T, Moore AC, Stevens MM, Guldberg RE, Bursac N. Translating musculoskeletal bioengineering into tissue regeneration therapies. Sci Transl Med. 2022;14:eabn9074. doi: 10.1126/scitranslmed.abn9074
- Cieza A, Causey K, Kamenov K, Hanson SW, Chatterji S, Vos T. Global estimates of the need for rehabilitation based on the Global Burden of Disease study 2019: A systematic analysis for the Global Burden of Disease Study 2019. Lancet. 2021;396:2006-2017. doi: 10.1016/s0140-6736(20)32340-0
- Zhang S, Xing M, Li B. Recent advances in musculoskeletal local drug delivery. Acta Biomater. 2019;93:135-151. doi: 10.1016/j.actbio.2019.01.043
- Agarwal R, Williams K, Umscheid CA, Welch WC. Osteoinductive bone graft substitutes for lumbar fusion: A systematic review. J Neurosurg Spine. 2009;11: 729-740. doi: 10.3171/2009.6.Spine08669
- Charalambides C, Beer M, Cobb AG. Poor results after augmenting autograft with xenograft (Surgibone) in hip revision surgery: A report of 27 cases. Acta Orthop. 2005;76: 544-549. doi: 10.1080/17453670510041547
- Shapira A, Dvir T. 3D tissue and organ printing—Hope and reality. Adv Sci. 2021;8:2003751. doi: 10.1002/advs.202003751
- Vanderburgh J, Sterling JA, Guelcher SA. 3D printing of tissue engineered constructs for in vitro modeling of disease progression and drug screening. Ann Biomed Eng. 2017;45:164-179. doi: 10.1007/s10439-016-1640-4
- Cui H, Miao S, Esworthy T, et al. 3D bioprinting for cardiovascular regeneration and pharmacology. Adv Drug Deliv Rev. 2018;132:252-269. doi: 10.1016/j.addr.2018.07.014
- Groll J, Boland T, Blunk T, et al. Biofabrication: Reappraising the definition of an evolving field. Biofabrication. 2016;8:013001. doi: 10.1088/1758-5090/8/1/013001
- Abaci A, Guvendiren M. Designing decellularized extracellular matrix-based bioinks for 3D bioprinting. Adv Healthc Mater. 2020;9:e2000734. doi: 10.1002/adhm.202000734
- Zhang Y, Haghiashtiani G, Hübscher T, et al. 3D extrusion bioprinting. Nat Rev Methods Primers. 2021;1:75. doi: 10.1038/s43586-021-00073-8
- Zhang T, Zhao W, Xiahou Z, Wang X, Zhang K, Yin J. Bioink design for extrusion-based bioprinting. Appl Mater Today. 2021;25:101227. doi: 10.1016/j.apmt.2021.101227
- Mandrycky C, Wang Z, Kim K, Kim D-H. 3D bioprinting for engineering complex tissues. Biotechnol Adv. 2016;34: 422-434. doi: 10.1016/j.biotechadv.2015.12.011
- Cui H, Nowicki M, Fisher JP, Zhang LG. 3D bioprinting for organ regeneration. Adv Healthc Mater. 2017;6:1601118. doi: 10.1002/adhm.201601118
- Li X, Liu B, Pei B, et al. Inkjet bioprinting of biomaterials. Chem Rev. 2020;120:10793-10833. doi: 10.1021/acs.chemrev.0c00008
- Cui X, Li J, Hartanto Y, et al. Advances in extrusion 3D bioprinting: A focus on multicomponent hydrogel-based bioinks. Adv Healthc Mater. 2020;9:1901648. doi: 10.1002/adhm.201901648
- Hölzl K, Lin S, Tytgat L, Vlierberghe SV, Gu L, Ovsianikov A. Bioink properties before, during and after 3D bioprinting. Biofabrication. 2016;8:032002. doi: 10.1088/1758-5090/8/3/032002
- Derakhshanfar S, Mbeleck R, Xu K, Zhang X, Zhong W, Xing M. 3D bioprinting for biomedical devices and tissue engineering: A review of recent trends and advances. Bioact Mater. 2018;3:144-156. doi: 10.1016/j.bioactmat.2017.11.008
- Murphy SV, Atala A. 3D bioprinting of tissues and organs. Nat Biotechnol. 2014;32:773-785. doi: 10.1038/nbt.2958
- Xu K, Han Y, Huang Y, Wei P, Yin J, Jiang J.The application of 3D bioprinting in urological diseases. Mater Today Bio. 2022;16:100388. doi: 10.1016/j.mtbio.2022.100388
- Li M, Sun D, Zhang J, Wang Y, Wei Q, Wang Y. Application and development of 3D bioprinting in cartilage tissue engineering. Biomater Sci. 2022;10:5430-5458. doi: 10.1039/D2BM00709F
- Bernal PN, Delrot P, Loterie D, et al. Volumetric bioprinting of complex living-tissue constructs within seconds. Adv Mater. 2019;31:e1904209. doi: 10.1002/adma.201904209
- Bernal PN, Bouwmeester M, Madrid-Wolff J, et al. Volumetric bioprinting of organoids and optically tuned hydrogels to build liver-like metabolic biofactories. Adv Mater. 2022;34:e2110054. doi: 10.1002/adma.202110054
- Rizzo R, Ruetsche D, Liu H, Zenobi-Wong M. Optimized photoclick (bio)resins for fast volumetric bioprinting. Adv Mater. 2021;33:e2102900. doi: 10.1002/adma.202102900
- Gehlen J, Qiu W, Schädli GN, Müller R, Qin X-H. Tomographic volumetric bioprinting of heterocellular bone-like tissues in seconds. Acta Biomater. 2023;156:49-60. doi: 10.1016/j.actbio.2022.06.020
- Ouyang L, Yao R, Zhao Y, Sun W. Effect of bioink properties on printability and cell viability for 3D bioplotting of embryonic stem cells. Biofabrication. 2016;8:035020. doi: 10.1088/1758-5090/8/3/035020
- Samandari M, Quint J, Rodríguez-delaRosa A, Pourquié O, Tamayol A. Bioinks and bioprinting strategies for skeletal muscle tissue engineering. Adv Mater. 2022;34:e2105883. doi: 10.1002/adma.202105883
- Beketov EE, Isaeva EV, Yakovleva ND, et al. Bioprinting of cartilage with bioink based on high-concentration collagen and chondrocytes. Int J Mol Sci. 2021;22:11351. doi: 10.3390/ijms222111351
- Hwangbo H, Lee H, Jin EJ, et al. Bio-printing of aligned GelMa-based cell-laden structure for muscle tissue regeneration. Bioact Mater. 2022;8:57-70. doi: 10.1016/j.bioactmat.2021.06.031
- Sonaye SY, Ertugral EG, Kothapalli CR, Sikder P. Extrusion 3D (bio)printing of alginate-gelatin-based composite scaffolds for skeletal muscle tissue engineering. Materials. 2022;15:7945. doi: 10.3390/ma15227945
- Fan T, Wang S, Jiang Z, et al. Controllable assembly of skeletal muscle-like bundles through 3D bioprinting. Biofabrication. 2021;14:015009. doi: 10.1088/1758-5090/ac3aca
- De Santis MM, Alsafadi HN, Tas S, et al. Extracellular-matrix-reinforced bioinks for 3D bioprinting human tissue. Adv Mater. 2021;33:e2005476. doi: 10.1002/adma.202005476
- Yang X, Lu Z, Wu H, Li W, Zheng L, Zhao J. Collagen-alginate as bioink for three-dimensional (3D) cell printing based cartilage tissue engineering. Mater Sci Eng C Mater Biol Appl. 2018;83:195-201. doi: 10.1016/j.msec.2017.09.002
- de Melo BAG, Jodat YA, Cruz EM, Benincasa JC, Shin SR, Porcionatto MA. Strategies to use fibrinogen as bioink for 3D bioprinting fibrin-based soft and hard tissues. Acta Biomater. 2020;117:60-76. doi: 10.1016/j.actbio.2020.09.024
- de Melo BAG, Jodat YA, Mehrotra S, et al. 3D printed cartilage-like tissue constructs with spatially controlled mechanical properties. Adv Funct Mater. 2019;29: 1906330. doi: 10.1002/adfm.201906330
- Li T, Hou J, Wang L, et al. Bioprinted anisotropic scaffolds with fast stress relaxation bioink for engineering 3D skeletal muscle and repairing volumetric muscle loss. Acta Biomater. 2022;156:21-36. doi: 10.1016/j.actbio.2022.08.037
- Visscher DO, Lee H, van Zuijlen PPM, et al. A photo-crosslinkable cartilage-derived extracellular matrix bioink for auricular cartilage tissue engineering. Acta Biomater. 2021;121:193-203. doi: 10.1016/j.actbio.2020.11.029
- Sahranavard M, Sarkari S, Safavi S, Ghorbani F. Three-dimensional bio-printing of decellularized extracellular matrix-based bio-inks for cartilage regeneration: A systematic review. Biomater Transl. 2022;3:105-115. doi: 10.12336/biomatertransl.2022.02.004
- Kim BS, Das S, Jang J, Cho D-W. Decellularized extracellular matrix-based bioinks for engineering tissue- and organ-specific microenvironments. Chem Rev. 2020;120:10608-10661. doi: 10.1021/acs.chemrev.9b00808
- Lee J, Hong J, Kim W, Kim GH. Bone-derived dECM/ alginate bioink for fabricating a 3D cell-laden mesh structure for bone tissue engineering. Carbohydr Polym. 2020;250:116914. doi: 10.1016/j.carbpol.2020.116914
- Yoncheva K, Calleja P, Agüeros M, et al. Stabilized micelles as delivery vehicles for paclitaxel. Int J Pharm. 2012;436:258- 264. doi: 10.1016/j.ijpharm.2012.06.030
- Shamma RN, Sayed RH, Madry H, El Sayed NS, Cucchiarini M. Triblock copolymer bioinks in hydrogel three-dimensional printing for regenerative medicine: A focus on pluronic f127. Tissue Eng Part B Rev. 2022;28:451-463. doi: 10.1089/ten.TEB.2021.0026
- Mozetic P, Giannitelli SM, Gori M, Trombetta M, Rainer A. Engineering muscle cell alignment through 3D bioprinting. J Biomed Mater Res A. 2017;105:2582-2588. doi: 10.1002/jbm.a.36117
- Shin YJ, Shafranek RT, Tsui JH, Walcott J, Nelson A, Kim D-H. 3D bioprinting of mechanically tuned bioinks derived from cardiac decellularized extracellular matrix. Acta Biomater. 2021;119:75-88. doi: 10.1016/j.actbio.2020.11.006
- Ying GL, Jiang N, Maharjan S, et al. Aqueous two-phase emulsion bioink-enabled 3D bioprinting of porous hydrogels. Adv Mater. 2018;30:e1805460. doi: 10.1002/adma.201805460
- Jia L, Hua Y, Zeng J, et al. Bioprinting and regeneration of auricular cartilage using a bioactive bioink based on microporous photocrosslinkable acellular cartilage matrix. Bioact Mater. 2022;16:66-81. doi: 10.1016/j.bioactmat.2022.02.032
- Zhang W, Wang N, Yang M, et al. Periosteum and development of the tissue-engineered periosteum for guided bone regeneration. J Orthop Translat. 2022;33: 41-54. doi: 10.1016/j.jot.2022.01.002
- Li Y, Pan Q, Xu J, et al. Overview of methods for enhancing bone regeneration in distraction osteogenesis: Potential roles of biometals. J Orthop Translat. 2021;27:110-118. doi: 10.1016/j.jot.2020.11.008
- Agarwal R, García AJ. Biomaterial strategies for engineering implants for enhanced osseointegration and bone repair. Adv Drug Deliv Rev. 2015;94:53-62. doi: 10.1016/j.addr.2015.03.013
- Ho-Shui-Ling A, Bolander J, Rustom LE, Johnson AW, Luyten FP, Picart C. Bone regeneration strategies: Engineered scaffolds, bioactive molecules and stem cells current stage and future perspectives. Biomaterials. 2018;180:143-162. doi: 10.1016/j.biomaterials.2018.07.017
- Mauffrey C, Barlow BT, Smith W. Management of segmental bone defects. J Am Acad Orthop Surg. 2015;23:143-153. doi: 10.5435/jaaos-d-14-00018
- Tang H, Bi F, Chen G, et al. 3D-bioprinted recombination structure of Hertwig’s epithelial root sheath cells and dental papilla cells for alveolar bone regeneration. Int J Bioprint. 2022;8:512. doi: 10.18063/ijb.v8i3.512
- Nulty J, Freeman FE, Browe DC, et al. 3D bioprinting of prevascularised implants for the repair of critically-sized bone defects. Acta Biomater. 2021;126:154-169. doi: 10.1016/j.actbio.2021.03.003
- Shen M, Wang L, Gao Y, et al. 3D bioprinting of in situ vascularized tissue engineered bone for repairing large segmental bone defects. Mater Today Bio. 2022;16: 100382. doi: 10.1016/j.mtbio.2022.100382
- Moncal KK, Tigli Aydın RS, Godzik KP, et al. Controlled Co-delivery of pPDGF-B and pBMP-2 from intraoperatively bioprinted bone constructs improves the repair of calvarial defects in rats. Biomaterials. 2022;281:121333. doi: 10.1016/j.biomaterials.2021.121333
- Kim W, Jang CH, Kim G. Bone tissue engineering supported by bioprinted cell constructs with endothelial cell spheroids. Theranostics. 2022;12:5404-5417. doi: 10.7150/thno.74852
- Wang M, Li H, Yang Y, et al. A 3D-bioprinted scaffold with doxycycline-controlled BMP2-expressing cells for inducing bone regeneration and inhibiting bacterial infection. Bioact Mater. 2021;6:1318-1329. doi: 10.1016/j.bioactmat.2020.10.022
- Sun X, Ma Z, Zhao X, et al. Three-dimensional bioprinting of multicell-laden scaffolds containing bone morphogenic protein-4 for promoting M2 macrophage polarization and accelerating bone defect repair in diabetes mellitus. Bioact Mater. 2021;6:757-769. doi: 10.1016/j.bioactmat.2020.08.030
- Zhu H, Monavari M, Zheng K, et al. 3D bioprinting of multifunctional dynamic nanocomposite bioinks incorporating Cu-doped mesoporous bioactive glass nanoparticles for bone tissue engineering. Small. 2022;18:e2104996. doi: 10.1002/smll.202104996
- Yu K, Huangfu H, Qin Q, et al. Application of bone marrow-derived macrophages combined with bone mesenchymal stem cells in dual-channel three-dimensional bioprinting scaffolds for early immune regulation and osteogenic induction in rat calvarial defects. ACS Appl Mater Interfaces. 2022;14:47052-47065. doi: 10.1021/acsami.2c13557
- Sun X, Jiao X, Yang X, et al. 3D bioprinting of osteon-mimetic scaffolds with hierarchical microchannels for vascularized bone tissue regeneration. Biofabrication. 2022;14:035008. doi: 10.1088/1758-5090/ac6700
- Pitacco P, Sadowska JM, O’Brien FJ, Kelly DJ. 3D bioprinting of cartilaginous templates for large bone defect healing. Acta Biomater. 2023;156:61-74. doi: 10.1016/j.actbio.2022.07.037
- Li Z, Li S, Yang J, et al. 3D bioprinted gelatin/gellan gum-based scaffold with double-crosslinking network for vascularized bone regeneration. Carbohydr Polym. 2022;290:119469. doi: 10.1016/j.carbpol.2022.119469
- Zhang J, Eyisoylu H, Qin XH, Rubert M, Müller R. 3D bioprinting of graphene oxide-incorporated cell-laden bone mimicking scaffolds for promoting scaffold fidelity, osteogenic differentiation and mineralization. Acta Biomater. 2021;121: 637-652. doi: 10.1016/j.actbio.2020.12.026
- Parthiban SP, Athirasala A, Tahayeri A, Abdelmoniem R, George A, Bertassoni LE. BoneMA-synthesis and characterization of a methacrylated bone-derived hydrogel for bioprinting of in-vitro vascularized tissue constructs. Biofabrication. 2021;13:035031. doi: 10.1088/1758-5090/abb11f
- Li L, Shi J, Ma K, et al. Robotic in situ 3D bio-printing technology for repairing large segmental bone defects. J Adv Res. 2021;30:75-84. doi: 10.1016/j.jare.2020.11.011
- Gehlen J, Qiu W, Schädli GN, Müller R, Qin X-H. Tomographic volumetric bioprinting of heterocellular bone-like tissues in seconds. Acta Biomater. 2022;156: 49-60. doi: 10.1016/j.actbio.2022.06.020
- Touya N, Devun M, Handschin C, et al. In vitro and in vivo characterization of a novel tricalcium silicate-based ink for bone regeneration using laser-assisted bioprinting. Biofabrication. 2022;14:024104. doi: 10.1088/1758-5090/ac584b
- Tao J, Zhu S, Liao X, et al. DLP-based bioprinting of void-forming hydrogels for enhanced stem-cell-mediated bone regeneration. Mater Today Bio. 2022;17:100487. doi: 10.1016/j.mtbio.2022.100487
- Rajput M, Mondal P, Yadav P, Chatterjee K. Light-based 3D bioprinting of bone tissue scaffolds with tunable mechanical properties and architecture from photocurable silk fibroin. Int J Biol Macromol. 2022;202:644-656. doi: 10.1016/j.ijbiomac.2022.01.081
- Ryan EJ, Ryan AJ, González-Vázquez A, et al. Collagen scaffolds functionalised with copper-eluting bioactive glass reduce infection and enhance osteogenesis and angiogenesis both in vitro and in vivo. Biomaterials. 2019;197: 405-416. doi: 10.1016/j.biomaterials.2019.01.031
- Wang J, Wang H, Wang Y, et al. Endothelialized microvessels fabricated by microfluidics facilitate osteogenic differentiation and promote bone repair. Acta Biomater. 2022;142:85-98. doi: 10.1016/j.actbio.2022.01.055
- Amler AK, Thomas A, Tüzüner S, et al. 3D bioprinting of tissue-specific osteoblasts and endothelial cells to model the human jawbone. Sci Rep. 2021;11:4876. doi: 10.1038/s41598-021-84483-4
- Li J, Han F, Ma J, et al. Targeting endogenous hydrogen peroxide at bone defects promotes bone repair. Adv Funct Mater. 2022;32:2111208. doi: 10.1002/adfm.202111208
- Arciola CR, Campoccia D, Montanaro L. Implant infections: Adhesion, biofilm formation and immune evasion. Nat Rev Microbiol. 2018;16:397-409. doi: 10.1038/s41579-018-0019-y
- Josse J, Valour F, Maali Y, et al. Interaction between staphylococcal biofilm and bone: How does the presence of biofilm promote prosthesis loosening? Front Microbiol. 2019;10:1602. doi: 10.3389/fmicb.2019.01602
- Schon BS, Hooper GJ, Woodfield TBF. Modular tissue assembly strategies for biofabrication of engineered cartilage. Ann Biomed Eng. 2017;45:100-114. doi: 10.1007/s10439-016-1609-3
- Wei W, Dai H. Articular cartilage and osteochondral tissue engineering techniques: Recent advances and challenges. Bioact Mater. 2021;6:4830-4855. doi: 10.1016/j.bioactmat.2021.05.011
- Borrelli J, Jr., Olson SA, Godbout C, Schemitsch EH, Stannard JP, Giannoudis PV. Understanding articular cartilage injury and potential treatments. J Orthop Trauma. 2019;33(Suppl 6):S6-S12. doi: 10.1097/bot.0000000000001472
- Daly AC, Freeman FE, Gonzalez-Fernandez T, Critchley SE, Nulty J, Kelly DJ. 3D bioprinting for cartilage and osteochondral tissue engineering. Adv Healthc Mater. 2017;6:1700298. doi: 10.1002/adhm.201700298
- Liu Y, Shah KM, Luo J. Strategies for articular cartilage repair and regeneration. Front Bioeng Biotechnol. 2021;9:770655. doi: 10.3389/fbioe.2021.770655
- Zhu S, Chen P, Chen Y, et al. 3D-printed extracellular matrix/polyethylene glycol diacrylate hydrogel incorporating the anti-inflammatory phytomolecule honokiol for regeneration of osteochondral defects. Am J Sports Med. 2020;48:2808-2818. doi: 10.1177/0363546520941842
- Hotham WE, Malviya A. A systematic review of surgical methods to restore articular cartilage in the hip. Bone Joint Res. 2018;7:336-342. doi: 10.1302/2046-3758.75.Bjr-2017-0331
- Hunziker EB. Articular cartilage repair: Basic science and clinical progress. A review of the current status and prospects. Osteoarthritis Cartilage. 2002;10:432-463. doi: 10.1053/joca.2002.0801
- Lim KS, Abinzano F, Bernal PN, et al. One-step photoactivation of a dual-functionalized bioink as cell carrier and cartilage-binding glue for chondral regeneration. Adv Healthc Mater. 2020;9:e1901792. doi: 10.1002/adhm.201901792
- Rathan S, Dejob L, Schipani R, Haffner B, Möbius ME, Kelly DJ. Fiber reinforced cartilage ECM functionalized bioinks for functional cartilage tissue engineering. Adv Healthc Mater. 2019;8:e1801501. doi: 10.1002/adhm.201801501
- Sun Y, You Y, Jiang W, Wang B, Wu Q, Dai K. 3D bioprinting dual-factor releasing and gradient-structured constructs ready to implant for anisotropic cartilage regeneration. Sci Adv. 2020;6:eaay1422. doi: 10.1126/sciadv.aay1422
- Zhang L, Tang H, Xiahou Z, et al. Solid multifunctional granular bioink for constructing chondroid basing on stem cell spheroids and chondrocytes. Biofabrication. 2022;14:035003. doi: 10.1088/1758-5090/ac63ee
- Bonifacio MA, Cometa S, Cochis A, et al. A bioprintable gellan gum/lignin hydrogel: A smart and sustainable route for cartilage regeneration. Int J Biol Macromol. 2022;216:336- 346. doi: 10.1016/j.ijbiomac.2022.07.002
- Wang B, Diaz-Payno PJ, Browe DC, et al. Affinity-bound growth factor within sulfated interpenetrating network bioinks for bioprinting cartilaginous tissues. Acta Biomater. 2021;128:130-142. doi: 10.1016/j.actbio.2021.04.016
- Shi W, Fang F, Kong Y, et al. Dynamic hyaluronic acid hydrogel with covalent linked gelatin as an anti-oxidative bioink for cartilage tissue engineering. Biofabrication. 2021;14:014107. doi: 10.1088/1758-5090/ac42de
- Li Z, Zhang X, Yuan T, et al. Addition of platelet-rich plasma to silk fibroin hydrogel bioprinting for cartilage regeneration. Tissue Eng Part A. 2020;26:886-895. doi: 10.1089/ten.TEA.2019.0304
- İlhan GT, Irmak G, Gümüşderelioğlu M. Microwave assisted methacrylation of Kappa carrageenan: A bioink for cartilage tissue engineering. Int J Biol Macromol. 2020;164:3523-3534. doi: 10.1016/j.ijbiomac.2020.08.241
- Antich C, de Vicente J, Jiménez G, et al. Bio-inspired hydrogel composed of hyaluronic acid and alginate as a potential bioink for 3D bioprinting of articular cartilage engineering constructs. Acta Biomater. 2020;106:114-123. doi: 10.1016/j.actbio.2020.01.046
- Sun Y, You Y, Jiang W, Zhai Z, Dai K. 3D-bioprinting a genetically inspired cartilage scaffold with GDF5-conjugated BMSC-laden hydrogel and polymer for cartilage repair. Theranostics. 2019;9:6949-6961. doi: 10.7150/thno.38061
- Kosik-Kozioł A, Costantini M, Mróz A, et al. 3D bioprinted hydrogel model incorporating β-tricalcium phosphate for calcified cartilage tissue engineering. Biofabrication. 2019;11:035016. doi: 10.1088/1758-5090/ab15cb
- Galarraga JH, Kwon MY, Burdick JA. 3D bioprinting via an in situ crosslinking technique towards engineering cartilage tissue. Sci Rep. 2019;9:19987. doi: 10.1038/s41598-019-56117-3
- Onofrillo C, Duchi S, O’Connell CD, et al. Biofabrication of human articular cartilage: A path towards the development of a clinical treatment. Biofabrication. 2018;10:045006. doi: 10.1088/1758-5090/aad8d9
- Huang Y, Meng X, Zhou Z, et al. A naringin-derived bioink enhances the shape fidelity of 3D bioprinting and efficiency of cartilage defect repair. J Mater Chem B. 2022;10:7030-7044. doi: 10.1039/d2tb01247b
- Lee AK, Lin YH, Tsai CH, Chang W-T, Lin T-L, Shie M-Y. Digital light processing bioprinted human chondrocyte-laden poly (γ-glutamic acid)/hyaluronic acid bio-ink towards cartilage tissue engineering. Biomedicines. 2021;9:714. doi: 10.3390/biomedicines9070714
- Lipskas J, Deep K, Yao W. Robotic-assisted 3D bio-printing for repairing bone and cartilage defects through a minimally invasive approach. Sci Rep. 2019;9:3746. doi: 10.1038/s41598-019-38972-2
- Ma K, Zhao T, Yang L, et al. Application of robotic-assisted in situ 3D printing in cartilage regeneration with HAMA hydrogel: An in vivo study. J Adv Res. 2020;23:123-132. doi: 10.1016/j.jare.2020.01.010
- Zhu W, Cui H, Boualam B, et al. 3D bioprinting mesenchymal stem cell-laden construct with core-shell nanospheres for cartilage tissue engineering. Nanotechnology. 2018;29:185101. doi: 10.1088/1361-6528/aaafa1
- Cui X, Breitenkamp K, Finn MG, Martin Lotz, D’Lima DD. Direct human cartilage repair using three-dimensional bioprinting technology. Tissue Eng Part A. 2012;18:1304-1312. doi: 10.1089/ten.TEA.2011.0543
- Burdis R, Chariyev-Prinz F, Kelly DJ. Bioprinting of biomimetic self-organised cartilage with a supporting joint fixation device. Biofabrication. 2021;14:015008. doi: 10.1088/1758-5090/ac36be
- Daly AC, Cunniffe GM, Sathy BN, Jeon O, Alsberg E, Kelly DJ. 3D bioprinting of developmentally inspired templates for whole bone organ engineering. Adv Healthc Mater. 2016;5: 2352-2352. doi: 10.1002/adhm.201670100
- Critchley SE, Kelly DJ. Bioinks for bioprinting functional meniscus and articular cartilage. J 3D Print Med. 2017;1: 269-290. doi: 10.2217/3dp-2017-0012
- Kosik-Kozioł A, Costantini M, Bolek T, et al. PLA short sub-micron fiber reinforcement of 3D bioprinted alginate constructs for cartilage regeneration. Biofabrication. 2017;9:044105. doi: 10.1088/1758-5090/aa90d7
- Pei Z, Gao M, Xing J, et al. Experimental study on repair of cartilage defects in the rabbits with GelMA-MSCs scaffold prepared by three-dimensional bioprinting. Int J Bioprint. 2023;9. doi: 10.18063/ijb.v9i2.662
- Mancini IAD, Vindas Bolaños RA, Brommer H, et al. Fixation of hydrogel constructs for cartilage repair in the equine model: A challenging issue. Tissue Eng Part C Methods. 2017;23:804-814. doi: 10.1089/ten.TEC.2017.0200
- Ofek G, Athanasiou K. Micromechanical properties of chondrocytes and chondrons: Relevance to articular cartilage tissue engineering. J Mech Mater Struct. 2007;2:1059-1086. doi: 10.2140/jomms.2007.2.1059
- Idaszek J, Costantini M, Karlsen TA, et al. 3D bioprinting of hydrogel constructs with cell and material gradients for the regeneration of full-thickness chondral defect using a microfluidic printing head. Biofabrication. 2019;11:044101. doi: 10.1088/1758-5090/ab2622
- Wu Y, Ayan B, Moncal KK, et al. Hybrid bioprinting of zonally stratified human articular cartilage using scaffold-free tissue strands as building blocks. Adv Healthc Mater. 2020;9:e2001657. doi: 10.1002/adhm.202001657
- Dai W, Zhang L, Yu Y, et al. 3D bioprinting of heterogeneous constructs providing tissue-specific microenvironment based on host–guest modulated dynamic hydrogel bioink for osteochondral regeneration. Adv Funct Mater. 2022;32:2200710. doi: 10.1002/adfm.202200710
- Distler T, Solisito AA, Schneidereit D, Friedrich O, Detsch R, Boccaccini AR. 3D printed oxidized alginate-gelatin bioink provides guidance for C2C12 muscle precursor cell orientation and differentiation via shear stress during bioprinting. Biofabrication. 2020;12:045005. doi: 10.1088/1758-5090/ab98e4
- Liu J, Saul D, Böker KO, Ernst J, Lehman W, Schilling AF. Current methods for skeletal muscle tissue repair and regeneration. Biomed Res Int. 2018;2018:1984879. doi: 10.1155/2018/1984879
- Ostrovidov S, Salehi S, Costantini M, et al. 3D bioprinting in skeletal muscle tissue engineering. Small. 2019;15:e1805530. doi: 10.1002/smll.201805530
- Tidball JG. Mechanisms of muscle injury, repair, and regeneration. Compr Physiol. 2011;1:2029-2062. doi: 10.1002/cphy.c100092
- Corona BT, Rivera JC, Owens JG, Wenke JC, Rathbone CR. Volumetric muscle loss leads to permanent disability following extremity trauma. J Rehabil Res Dev. 2015;52:785-792. doi: 10.1682/JRRD.2014.07.0165
- Langridge B, Griffin M, Butler PE. Regenerative medicine for skeletal muscle loss: A review of current tissue engineering approaches. J Mater Sci Mater Med. 2021;32:15. doi: 10.1007/s10856-020-06476-5
- Lin CH, Lin YT, Yeh JT, Chen C-T. Free functioning muscle transfer for lower extremity posttraumatic composite structure and functional defect. Plast Reconstr Surg. 2007;119:2118-2126. doi: 10.1097/01.prs.0000260595.85557.41
- Aguilar CA, Greising SM, Watts A, et al. Multiscale analysis of a regenerative therapy for treatment of volumetric muscle loss injury. Cell Death Discov. 2018;4:33. doi: 10.1038/s41420-018-0027-8
- Choi YJ, Jun YJ, Kim DY, et al. A 3D cell printed muscle construct with tissue-derived bioink for the treatment of volumetric muscle loss. Biomaterials. 2019;206:160-169. doi: 10.1016/j.biomaterials.2019.03.036
- Behre A, Tashman JW, Dikyol C, et al. 3D bioprinted patient-specific extracellular matrix scaffolds for soft tissue defects. Adv Healthc Mater. 2022;11:e2200866. doi: 10.1002/adhm.202200866
- Luo Z, Tang G, Ravanbakhsh H, et al. Vertical extrusion cryo(bio)printing for anisotropic tissue manufacturing. Adv Mater. 2022;34:e2108931. doi: 10.1002/adma.202108931
- Mostafavi A, Samandari M, Karvar M, et al. Colloidal multiscale porous adhesive (bio)inks facilitate scaffold integration. Appl Phys Rev. 2021;8:041415. doi: 10.1063/5.0062823
- Kim JH, Kim I, Seol YJ, et al. Neural cell integration into 3D bioprinted skeletal muscle constructs accelerates restoration of muscle function. Nat Commun. 2020;11:1025. doi: 10.1038/s41467-020-14930-9
- Christensen KW, Turner J, Coughenour K, et al. Assembled cell-decorated collagen (AC-DC) fiber bioprinted implants with musculoskeletal tissue properties promote functional recovery in volumetric muscle loss. Adv Healthc Mater. 2022;11:e2101357. doi: 10.1002/adhm.202101357
- Wang Y, Wang Q, Luo S, et al. 3D bioprinting of conductive hydrogel for enhanced myogenic differentiation. Regen Biomater. 2021;8:rbab035. doi: 10.1093/rb/rbab035
- Kim JH, Seol YJ, Ko IK, et al. 3D bioprinted human skeletal muscle constructs for muscle function restoration. Sci Rep. 2018;8:12307. doi: 10.1038/s41598-018-29968-5
- Yang GH, Kim W, Kim J, Kim GH. A skeleton muscle model using GelMA-based cell-aligned bioink processed with an electric-field assisted 3D/4D bioprinting. Theranostics. 2021;11:48-63. doi: 10.7150/thno.50794
- Kim W, Jang CH, Kim GH. A myoblast-laden collagen bioink with fully aligned Au nanowires for muscle-tissue regeneration. Nano Lett. 2019;19:8612-8620. doi: 10.1021/acs.nanolett.9b03182
- Yeo M, Kim G. Electrohydrodynamic-direct-printed cell-laden microfibrous structure using alginate-based bioink for effective myotube formation. Carbohydr Polym. 2021;272:118444. doi: 10.1016/j.carbpol.2021.118444
- Chen Y, Zhang J, Liu X, et al. Noninvasive in vivo 3D bioprinting. Sci Adv. 2020;6:eaba7406. doi: 10.1126/sciadv.aba7406
- Urciuolo A, Poli I, Brandolino L, et al. Intravital three-dimensional bioprinting. Nat Biomed Eng. 2020;4:901-915. doi: 10.1038/s41551-020-0568-z
- Jana S, Levengood SK, Zhang M. Anisotropic materials for skeletal-muscle-tissue engineering. Adv Mater. 2016;28:10588-10612. doi: 10.1002/adma.201600240
- Zhang Y, Zhang Z, Wang Y, Su Y, Chen M. 3D myotube guidance on hierarchically organized anisotropic and conductive fibers for skeletal muscle tissue engineering. Mater Sci Eng C Mater Biol Appl. 2020;116:111070. doi: 10.1016/j.msec.2020.111070
- Ostrovidov S, Hosseini V, Ahadian S, et al. Skeletal muscle tissue engineering: Methods to form skeletal myotubes and their applications. Tissue Eng Part B Rev. 2014;20:403-436. doi: 10.1089/ten.TEB.2013.0534
- Yeo M, Kim G. Three-dimensional microfibrous bundle structure fabricated using an electric field-assisted/cell printing process for muscle tissue regeneration. ACS Biomater Sci Eng. 2018;4:728-738. doi: 10.1021/acsbiomaterials.7b00983
- Bilgen B, Jayasuriya CT, Owens BD. Current concepts in meniscus tissue engineering and repair. Adv Healthc Mater. 2018;7:1701407. doi: 10.1002/adhm.201701407
- Chae S, Lee SS, Choi YJ, et al. 3D cell-printing of biocompatible and functional meniscus constructs using meniscus-derived bioink. Biomaterials. 2021;267:120466. doi: 10.1016/j.biomaterials.2020.120466
- Kwon H, Brown WE, Lee CA, et al. Surgical and tissue engineering strategies for articular cartilage and meniscus repair. Nat Rev Rheumatol. 2019;15:550-570. doi: 10.1038/s41584-019-0255-1
- Roemer FW, Kwoh CK, Hannon MJ, et al. Partial meniscectomy is associated with increased risk of incident radiographic osteoarthritis and worsening cartilage damage in the following year. Eur Radiol. 2017;27:404-413. doi: 10.1007/s00330-016-4361-z
- Noyes FR, Barber-Westin SD. Long-term survivorship and function of meniscus transplantation. Am J Sports Med. 2016; 44:2330-2338. doi: 10.1177/0363546516646375
- Rosso F, Bisicchia S, Bonasia DE, Amendola A. Meniscal allograft transplantation: A systematic review. Am J Sports Med. 2015;43:998-1007. doi: 10.1177/0363546514536021
- Jiang D, Ao YF, Gong X, Wang Y-J, Zheng Z-Z, Yu J-K. Comparative study on immediate versus delayed meniscus allograft transplantation: 4- to 6-year follow-up. Am J Sports Med. 2014;42:2329-2337. doi: 10.1177/0363546514541653
- Costa JB, Park J, Jorgensen AM, et al. 3D bioprinted highly elastic hybrid constructs for advanced fibrocartilaginous tissue regeneration. Chem Mater. 2020;32: 8733-8746. doi: 10.1021/acs.chemmater.0c03556
- Jian Z, Zhuang T, Qinyu T, et al. 3D bioprinting of a biomimetic meniscal scaffold for application in tissue engineering. Bioact Mater. 2021;6:1711-1726. doi: 10.1016/j.bioactmat.2020.11.027
- Stocco TD, Moreira Silva MC, Corat MAF, AO L. Towards bioinspired meniscus-regenerative scaffolds: Engineering a novel 3D bioprinted patient-specific construct reinforced by biomimetically aligned nanofibers. Int J Nanomed. 2022;17:1111-1124. doi: 10.2147/ijn.S353937
- Terpstra ML, Li J, Mensinga A, et al. Bioink with cartilage-derived extracellular matrix microfibers enables spatial control of vascular capillary formation in bioprinted constructs. Biofabrication. 2022;14:034104. doi: 10.1088/1758-5090/ac6282
- Sathish PB, Gayathri S, Priyanka J, et al. Tricomposite gelatin-carboxymethylcellulose-alginate bioink for direct and indirect 3D printing of human knee meniscal scaffold. Int J Biol Macromol. 2022;195:179-189. doi: 10.1016/j.ijbiomac.2021.11.184
- Sun Y, Zhang Y, Wu Q, et al. 3D-bioprinting ready-to-implant anisotropic menisci recapitulate healthy meniscus phenotype and prevent secondary joint degeneration. Theranostics. 2021;11:5160-5173. doi: 10.7150/thno.54864
- Lan X, Ma Z, Szojka ARA, et al. TEMPO-oxidized cellulose nanofiber-alginate hydrogel as a bioink for human meniscus tissue engineering. Front Bioeng Biotechnol. 2021;9: 766399. doi: 10.3389/fbioe.2021.766399
- Hao L, Tianyuan Z, Zhen Y, et al. Biofabrication of cell-free dual drug-releasing biomimetic scaffolds for meniscal regeneration. Biofabrication. 2021;14:015001. doi: 10.1088/1758-5090/ac2cd7
- Filardo G, Petretta M, Cavallo C, et al. Patient-specific meniscus prototype based on 3D bioprinting of human cell-laden scaffold. Bone Joint Res. 2019;8:101-106. doi: 10.1302/2046-3758.82.Bjr-2018-0134.R1
- Chansoria P, Narayanan LK, Schuchard K, Shirwaiker R. Ultrasound-assisted biofabrication and bioprinting of preferentially aligned three-dimensional cellular constructs. Biofabrication. 2019;11:035015. doi: 10.1088/1758-5090/ab15cf
- Ali AM, Newman SDS, Hooper PA, Davies CM, Cobb JP. The effect of implant position on bone strain following lateral unicompartmental knee arthroplasty: A biomechanical model using digital image correlation. Bone Joint Res. 2017;6: 522-529. doi: 10.1302/2046-3758.68.Bjr-2017-0067.R1
- Badylak SF. The extracellular matrix as a biologic scaffold material. Biomaterials. 2007;28:3587-3593. doi: 10.1016/j.biomaterials.2007.04.043
- Frantz C, Stewart KM, Weaver VM. The extracellular matrix at a glance. J Cell Sci. 2010;123:4195-4200. doi: 10.1242/jcs.023820
- Makris EA, Hadidi P, Athanasiou KA. The knee meniscus: Structure-function, pathophysiology, current repair techniques, and prospects for regeneration. Biomaterials. 2011;32:7411-7431. doi: 10.1016/j.biomaterials.2011.06.037
- Narayanan LK, Huebner P, Fisher MB, Spang JT, Starly B, Shirwaiker RA. 3D-bioprinting of polylactic acid (PLA) nanofiber-alginate hydrogel bioink containing human adipose-derived stem cells. ACS Biomater Sci Eng. 2016;2:1732-1742. doi: 10.1021/acsbiomaterials.6b00196
- MacBarb RF, Chen AL, Hu JC, Athanasiou KA. Engineering functional anisotropy in fibrocartilage neotissues. Biomaterials. 2013;34:9980-9989. doi: 10.1016/j.biomaterials.2013.09.026
- Bonnet CS, Walsh DA. Osteoarthritis, angiogenesis and inflammation. Rheumatology. 2005;44:7-16. doi: 10.1093/rheumatology/keh344
- Mapp PI, Walsh DA. Mechanisms and targets of angiogenesis and nerve growth in osteoarthritis. Nat Rev Rheumatol. 2012;8:390-398. doi: 10.1038/nrrheum.2012.80
- Ashraf S, Wibberley H, Mapp PI, Hill R, Wilson D, Walsh DA. Increased vascular penetration and nerve growth in the meniscus: A potential source of pain in osteoarthritis. Ann Rheum Dis. 2011;70:523-529. doi: 10.1136/ard.2010.137844
- Freemont AJ, Watkins A, Le Maitre C, et al. Nerve growth factor expression and innervation of the painful intervertebral disc. J Pathol. 2002;197:286-292. doi: 10.1002/path.1108
- Ohnishi T, Iwasaki N, Sudo H. Causes of and molecular targets for the treatment of intervertebral disc degeneration: A review. Cells. 2022;11:394. doi: 10.3390/cells11030394
- Peng Y, Qing X, Shu H, et al. Proper animal experimental designs for preclinical research of biomaterials for intervertebral disc regeneration. Biomater Transl. 2021;2:91-142. doi: 10.12336/biomatertransl.2021.02.003
- Feng Y, Egan B, Wang J. Genetic factors in intervertebral disc degeneration. Genes Dis. 2016;3:178-185. doi: 10.1016/j.gendis.2016.04.005
- Wu D, Li G, Zhou X, Zhang W. Repair strategies and bioactive functional materials for intervertebral disc. Adv Funct Mater. 2022;32:2209471. doi: 10.1002/adfm.202209471
- van Uden S, Silva-Correia J, Oliveira JM, Reis RL. Current strategies for treatment of intervertebral disc degeneration: Substitution and regeneration possibilities. Biomater Res. 2017;21:22. doi: 10.1186/s40824-017-0106-6
- JT M, AH M, JA C, et al. Translation of an engineered nanofibrous disc-like angle-ply structure for intervertebral disc replacement in a small animal model. Acta Biomater. 2014;10:2473-2481. doi: 10.1016/j.actbio.2014.02.024
- Yang J, Wang L, Zhang W, et al. Reverse reconstruction and bioprinting of bacterial cellulose-based functional total intervertebral disc for therapeutic implantation. Small. 2018;14:1702582. doi: 10.1002/smll.201702582
- Gullbrand SE, Kim DH, Bonnevie E, et al. Towards the scale up of tissue engineered intervertebral discs for clinical application. Acta Biomater. 2018;70:154-164. doi: 10.1016/j.actbio.2018.01.050
- Yang J, Yang X, Wang L, et al. Biomimetic nanofibers can construct effective tissue-engineered intervertebral discs for therapeutic implantation. Nanoscale. 2017;9:13095. doi: 10.1039/c7nr03944a
- Bhunia BK, Dey S, Bandyopadhyay A, Mandal BB. 3D printing of annulus fibrosus anatomical equivalents recapitulating angle-ply architecture for intervertebral disc replacement. Appl Mater Today. 2021;23:101031. doi: 10.1016/j.apmt.2021.101031
- Liu Z, Wang H, Yuan Z, et al. High-resolution 3D printing of angle-ply annulus fibrosus scaffolds for intervertebral disc regeneration. Biofabrication. 2022;15:015015. doi: 10.1088/1758-5090/aca71f
- Hu D, Wu D, Huang L, et al. 3D bioprinting of cell-laden scaffolds for intervertebral disc regeneration. Mater Lett. 2018;223:219-222. doi: 10.1016/j.matlet.2018.03.204
- Parker KK, Healy K, Bursac N. Tissue-engineered disease models. Nat Biomed Eng. 2018;2:879-880. doi: 10.1038/s41551-018-0339-2
- Moran CJ, Ramesh A, Brama PA, O’Byrne JM, O’Brien FJ, Levingstone TJ. The benefits and limitations of animal models for translational research in cartilage repair. J Exp Orthop. 2016;3:1. doi: 10.1186/s40634-015-0037-x
- Benam KH, Dauth S, Hassell B, et al. Engineered in vitro disease models. Annu Rev Pathol. 2015;10:195-262. doi: 10.1146/annurev-pathol-012414-040418
- Takahashi K, Yamanaka S. Induction of pluripotent stem cells from mouse embryonic and adult fibroblast cultures by defined factors. Cell. 2006;126:663-676. doi: 10.1016/j.cell.2006.07.024
- Vandana JJ, Manrique C, Lacko LA, Chen S. Human pluripotent-stem-cell-derived organoids for drug discovery and evaluation. Cell Stem Cell. 2023;30:571-591. doi: 10.1016/j.stem.2023.04.011
- Corsini NS, Knoblich JA. Human organoids: New strategies and methods for analyzing human development and disease. Cell. 2022;185:2756-2769. doi: 10.1016/j.cell.2022.06.051
- Mota C, Camarero-Espinosa S, Baker MB, Wieringa P, Moroni L. Bioprinting: From tissue and organ development to in vitro models. Chem Rev. 2020;120:10547-10607. doi: 10.1021/acs.chemrev.9b00789
- Kurian AG, Singh RK, Patel KD, Lee J-H, Kim H-W. Multifunctional GelMA platforms with nanomaterials for advanced tissue therapeutics. Bioact Mater. 2022;8:267-295. doi: 10.1016/j.bioactmat.2021.06.027
- Kang HW, Lee SJ, Ko IK, Kengla C, Yoo JJ, Atala A. A 3D bioprinting system to produce human-scale tissue constructs with structural integrity. Nat Biotechnol. 2016;34: 312-319. doi: 10.1038/nbt.3413
- Kim BS, Ahn M, Cho WW, Gao G, Jang J, Cho D-W. Engineering of diseased human skin equivalent using 3D cell printing for representing pathophysiological hallmarks of type 2 diabetes in vitro. Biomaterials. 2021;272: 120776. doi: 10.1016/j.biomaterials.2021.120776
- Bin Y, Dongzhen Z, Xiaoli C, et al. Modeling human hypertrophic scars with 3D preformed cellular aggregates bioprinting. Bioact Mater. 2022;10:247-254. doi: 10.1016/j.bioactmat.2021.09.004
- Neufeld L, Yeini E, Pozzi S, Satchi-Fainaro R. 3D bioprinted cancer models: From basic biology to drug development. Nat Rev Cancer. 2022;22:679-692. doi: 10.1038/s41568-022-00514-w
- Jain P, Kathuria H, Dubey N. Advances in 3D bioprinting of tissues/organs for regenerative medicine and in-vitro models. Biomaterials. 2022;287:121639. doi: 10.1016/j.biomaterials.2022.121639
- Han J, Jeon S, Kim MK, Jeong W, Yoo JJ, Kang H-W. In vitro breast cancer model with patient-specific morphological features for personalized medicine. Biofabrication. 2022;14:034102. doi: 10.1088/1758-5090/ac6127
- Neufeld L, Yeini E, Reisman N, et al. Microengineered perfusable 3D-bioprinted glioblastoma model for in vivo mimicry of tumor microenvironment. Sci Adv. 2021;7:eabi9119. doi: 10.1126/sciadv.abi9119
- Hakobyan D, Médina C, Dusserre N, et al. Laser-assisted 3D bioprinting of exocrine pancreas spheroid models for cancer initiation study. Biofabrication. 2020;12:035001. doi: 10.1088/1758-5090/ab7cb8
- Tang M, Rich JN, Chen S. Biomaterials and 3D bioprinting strategies to model glioblastoma and the blood-brain barrier. Adv Mater. 2021;33:e2004776. doi: 10.1002/adma.202004776
- Gao Y, Fu Z, Guan J, Liu X, Zhang Q. The role of Notch signaling pathway in metabolic bone diseases. Biochem Pharmacol. 2023;207:115377. doi: 10.1016/j.bcp.2022.115377
- Greenblatt MB, Tsai JN, Wein MN. Bone turnover markers in the diagnosis and monitoring of metabolic bone disease. Clin Chem. 2017;63:464-474. doi: 10.1373/clinchem.2016.259085
- Breathwaite E, Weaver J, Odanga J, dela Pena-Ponce M, Lee JB. 3D bioprinted osteogenic tissue models for in vitro drug screening. Molecules. 2020;25:3442. doi: 10.3390/molecules25153442
- Breathwaite EK, Weaver JR, Murchison AC, Treadwell ML, Odanga JJ, Lee JB. Scaffold-free bioprinted osteogenic and chondrogenic systems to model osteochondral physiology. Biomed Mater. 2019;14:065010. doi: 10.1088/1748-605X/ab4243
- Martel-Pelletier J, Barr AJ, Cicuttini FM, et al. Osteoarthritis. Nat Rev Dis Primers. 2016;2:16072. doi: 10.1038/nrdp.2016.72
- Singh YP, Moses JC, Bandyopadhyay A, Mandal BB. 3D bioprinted silk-based in vitro osteochondral model for osteoarthritis therapeutics. Adv Healthc Mater. 2022;11:2200209. doi: 10.1002/adhm.202200209
- Furrer R, Handschin C. Muscle wasting diseases: Novel targets and treatments. Annu Rev Pharmacol Toxicol. 2019;59:315-339. doi: 10.1146/annurev-pharmtox-010818-021041
- Alave Reyes-Furrer A, De Andrade S, Bachmann D, et al. Matrigel 3D bioprinting of contractile human skeletal muscle models recapitulating exercise and pharmacological responses. Commun Biol. 2021;4:1183. doi: 10.1038/s42003-021-02691-0
- Datta P, Wu Y, Yu Y, Moncal KK, Ozbolat IT. A scaffold free 3D bioprinted cartilage model for in vitro toxicology. Methods Mol Biol. 2021;2147:175-183. doi: 10.1007/978-1-0716-0611-7_15
- Ouyang L. Pushing the rheological and mechanical boundaries of extrusion-based 3D bioprinting. Trends Biotechnol. 2022;40:891-902. doi: 10.1016/j.tibtech.2022.01.001
- Ouyang L, Armstrong JPK, Lin Y, et al. Expanding and optimizing 3D bioprinting capabilities using complementary network bioinks. Sci Adv. 2020;6:eabc5529. doi: 10.1126/sciadv.abc5529
- Chimene D, Peak CW, Gentry JL, et al. Nanoengineered ionic– covalent entanglement (nice) bioinks for 3D bioprinting. ACS Appl Mater Interfaces. 2018;10:9957-9968. doi: 10.1021/acsami.7b19808
- Dubey N, Ferreira JA, Daghrery A, et al. Highly tunable bioactive fiber-reinforced hydrogel for guided bone regeneration. Acta Biomater. 2020;113:164-176. doi: 10.1016/j.actbio.2020.06.011
- Shao L, Gao Q, Xie C, Fu J, Xiang M, He Y. Synchronous 3D bioprinting of large-scale cell-laden constructs with nutrient networks. Adv Healthc Mater. 2020;9:e1901142. doi: 10.1002/adhm.201901142
- Brassard JA, Nikolaev M, Hübscher T, Hofer M, Lutolf MP. Recapitulating macro-scale tissue self-organization through organoid bioprinting. Nat Mater. 2021;20:22-29. doi: 10.1038/s41563-020-00803-5