Research progress of 3D-bioprinted functional pancreas and in vitro tumor models
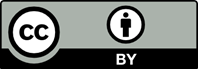
With the rapid development of three-dimensional (3D) bioprinting technology, the research revolving around in vitro functional pancreas and tumor models has become the focus of attention in the field of life sciences. This review aims to summarize and deeply discuss the research progress and prospects of 3D-bioprinted functional pancreas and in vitro tumor models. The efforts in improving 3D printing technology to increase its accuracy and reliability in the biomedical applications have been ramped up over the past few years. Researchers are now able to create highly complex 3D structures through precise layering of biological materials at the micron scale. For instance, a functional pancreas can be printed in vitro by combining cells, biomaterials, and growth factors. The introduction of new technologies allows researchers to more accurately simulate the growth and spread of tumors, providing a more realistic platform for cancer treatment research. This not only helps accelerate the process of drug screening, but also lays the foundation for personalized medicine. As multiple disciplines, such as materials science, cell biology, and engineering, continue to converge with 3D bioprinting, emergence of more innovative applications is anticipated. However, despite significant progress, many technical and ethical challenges still need to be overcome before practical clinical applications can be implemented. In summary, the application of bioprinting technology is of great significance to the study of functional pancreas and in vitro tumor models, which could lead to new breakthroughs in the development of clinical treatment and personalized medicine.
- Yang J, Xu J, Zhang B, et al. Ferroptosis: At the crossroad of gemcitabine resistance and tumorigenesis in pancreatic cancer. Int J Mol Sci. 2021;22(20):10944. doi: 10.3390/ijms222010944
- Zhou P, Li B, Liu F, et al. The epithelial to mesenchymal transition (EMT) and cancer stem cells: Implication for treatment resistance in pancreatic cancer. Mol Cancer. 2017;16(1):52. doi: 10.1186/s12943-017-0624-9
- Sherman MH, Beatty GL. Tumor microenvironment in pancreatic cancer pathogenesis and therapeutic resistance. Annu Rev Pathol. 2023;18: 123-148. doi: 10.1146/annurev-pathmechdis-031621-024600
- Masuo K, Chen R, Yogo A, et al. SNAIL2 contributes to tumorigenicity and chemotherapy resistance in pancreatic cancer by regulating IGFBP2. Cancer Sci. 2021;112(12):4987- 4999. doi: 10.1111/cas.15162
- Wei L, Wen JY, Chen J, et al. Oncogenic ADAM28 induces gemcitabine resistance and predicts a poor prognosis in pancreatic cancer. World J Gastroenterol. 2019;25(37): 5590-5603. doi: 10.3748/wjg.v25.i37.5590
- Thummuri D, Khan S, Underwood PW, et al. Overcoming gemcitabine resistance in pancreatic cancer using the BCL-XL-specific degrader DT2216. Mol Cancer Ther. 2022;21(1):184-192. doi: 10.1158/1535-7163.MCT-21-0474
- Yu S, Wang L, Che D, et al. Targeting CRABP-II overcomes pancreatic cancer drug resistance by reversing lipid raft cholesterol accumulation and AKT survival signaling. J Exp Clin Cancer Res. 2022;41(1):88. doi: 10.1186/s13046-022-02261-0
- Yang G, Guan W, Cao Z, et al. Integrative genomic analysis of gemcitabine resistance in pancreatic cancer by patient-derived xenograft models. Clin Cancer Res. 2021;27(12):3383-3396. doi: 10.1158/1078-0432.CCR-19-3975
- Capula M, Perán M, Xu G, et al. Role of drug catabolism, modulation of oncogenic signaling and tumor microenvironment in microbe-mediated pancreatic cancer chemoresistance. Drug Resist Updat. 2022;64: 100864. doi: 10.1016/j.drup.2022.100864
- Palikuqi B, Nguyen DT, Li G, et al. Adaptable haemodynamic endothelial cells for organogenesis and tumorigenesis. Nature. 2020;585(7825):426-432. doi: 10.1038/s41586-020-2712-z
- Meng Q, Liang C, Hua J, et al. A miR-146a-5p/TRAF6/NF-kB p65 axis regulates pancreatic cancer chemoresistance: Functional validation and clinical significance. Theranostics. 2020;10(9):3967-3979. doi: 10.7150/thno.40566
- Xu Y, Song D, Wang X. 3D bioprinting for pancreas engineering/ manufacturing. Polymers (Basel). 2022;14(23):5143. doi: 10.3390/polym14235143
- Zhao T, Xiao D, Jin F, et al. ESE3-positive PSCs drive pancreatic cancer fibrosis, chemoresistance and poor prognosis via tumour-stromal IL-1β/NF-κB/ESE3 signalling axis. Br J Cancer. 2022;127(8):1461-1472. doi: 10.1038/s41416-022-01927-y
- Grasso C, Jansen G, Giovannetti E. Drug resistance in pancreatic cancer: Impact of altered energy metabolism. Crit Rev Oncol Hematol. 2017;114: 139-152. doi: 10.1016/j.critrevonc.2017.03.026
- Niedźwiedzka-Rystwej P, Wołącewicz M, Cywoniuk P, et al. Transformation of stem cells used in the 3D bioprinting process as a personalized treatment method for type 1 diabetes. Arch Immunol Ther Exp (Warsz). 2020;68(2):13. doi: 10.1007/s00005-020-00578-2
- Hennig A, Baenke F, Klimova A, et al. Detecting drug resistance in pancreatic cancer organoids guides optimized chemotherapy treatment. J Pathol. 2022;257(5):607-619. doi: 10.1002/path.5906
- Halbrook CJ, Pontious C, Kovalenko I, et al. Macrophage-released pyrimidines inhibit gemcitabine therapy in pancreatic cancer. Cell Metab. 2019;29(6):1390-1399.e6. doi: 10.1016/j.cmet.2019.02.001
- Grattarola M, Cucci MA, Roetto A, et al. Post-translational down-regulation of Nrf2 and YAP proteins, by targeting deubiquitinases, reduces growth and chemoresistance in pancreatic cancer cells. Free Radic Biol Med. 2021;174: 202-210. doi: 10.1016/j.freeradbiomed.2021.08.006
- Gautam SK, Basu S, Aithal A, et al. Regulation of pancreatic cancer therapy resistance by chemokines. Semin Cancer Biol. 2022;86(Pt 2):69-80. doi: 10.1016/j.semcancer.2022.08.010
- Ning E, Turnbull G, Clarke J, et al. 3D bioprinting of mature bacterial biofilms for antimicrobial resistance drug testing. Biofabrication. 2019;11(4):045018. doi: 10.1088/1758-5090/ab37a0
- Wang Y, Shi W, Kuss M, et al. 3D bioprinting of breast cancer models for drug resistance study. ACS Biomater Sci Eng. 2018;4(12):4401-4411. doi: 10.1021/acsbiomaterials.8b01277
- Papaefthymiou A, Doukatas A, Galanopoulos M. Pancreatic cancer and oligonucleotide therapy: Exploring novel therapeutic options and targeting chemoresistance. Clin Res Hepatol Gastroenterol. 2022;46(5):101911. doi: 10.1016/j.clinre.2022.101911
- Han X, Zhang WH, Wang WQ, et al. Cancer-associated fibroblasts in therapeutic resistance of pancreatic cancer: Present situation, predicaments, and perspectives. Biochim Biophys Acta Rev Cancer. 2020;1874(2):188444. doi: 10.1016/j.bbcan.2020.188444
- Sheikh R, Walsh N, Clynes M, et al. Challenges of drug resistance in the management of pancreatic cancer. Expert Rev Anticancer Ther. 2010;10(10):1647-1661. doi: 10.1586/era.10.148
- Long J, Zhang Y, Yu X, et al. Overcoming drug resistance in pancreatic cancer. Expert Opin Ther Targets. 2011;15(7):817- 828. doi: 10.1517/14728222.2011.566216
- Hakobyan D, Médina C, Dusserre N, et al. Laser-assisted 3D bioprinting of exocrine pancreas spheroid models for cancer initiation study. Biofabrication. 2020;12(3):035001. doi: 10.1088/1758-5090/ab7cb8
- Kuo YC, Kou HW, Hsu CP, et al. Identification and clinical significance of pancreatic cancer stem cells and their chemotherapeutic drug resistance. Int J Mol Sci. 2023; 24(8):7331. doi: 10.3390/ijms24087331
- Capurso G, Sette C. Drug resistance in pancreatic cancer: New player caught in act. EBioMedicine. 2019;40: 39-40. doi: 10.1016/j.ebiom.2019.02.008
- Beatty GL, Werba G, Lyssiotis CA, et al. The biological underpinnings of therapeutic resistance in pancreatic cancer. Genes Dev. 2021;35(13-14):940-962. doi: 10.1101/gad.348523.121
- Gungor-Ozkerim PS, Inci I, Zhang YS, Khademhosseini A, Dokmeci MR. Bioinks for 3D bioprinting: An overview. Biomater Sci. 2018;6(5):915-946. doi: 10.1039/c7bm00765e
- Fitzgerald TL, McCubrey JA. Pancreatic cancer stem cells: Association with cell surface markers, prognosis, resistance, metastasis and treatment. Adv Biol Regul. 2014;56: 45-50. doi: 10.1016/j.jbior.2014.05.001
- Okazaki J, Tanahashi T, Sato Y, et al. MicroRNA-296-5p promotes cell invasion and drug resistance by targeting Bcl2-related ovarian killer, leading to a poor prognosis in pancreatic cancer. Digestion. 2020;101(6):794-806. doi: 10.1159/000503225
- Zhan T, Chen X, Tian X, et al. MiR-331-3p links to drug resistance of pancreatic cancer cells by activating WNT/β- catenin signal via ST7L. Technol Cancer Res Treat. 2020;19: 1533033820945801. doi: 10.1177/1533033820945801
- Hong N, Yang GH, Lee J, et al. 3D bioprinting and its in vivo applications. J Biomed Mater Res B Appl Biomater. 2018;106(1):444-459. doi: 10.1002/jbm.b.33826
- Yu S, Wang M, Zhang H, et al. Circ_0092367 inhibits EMT and gemcitabine resistance in pancreatic cancer via regulating the miR-1206/ESRP1 axis. Genes (Basel). 2021;12(11):1701. doi: 10.3390/genes12111701
- Szlasa W, Michel O, Sauer N, et al. Nanosecond pulsed electric field suppresses growth and reduces multi-drug resistance effect in pancreatic cancer. Sci Rep. 2023;13(1):351. doi: 10.1038/s41598-023-27605-4
- Cui J, Jiang W, Wang S, et al. Role of Wnt/β-catenin signaling in drug resistance of pancreatic cancer. Curr Pharm Des. 2012;18(17):2464-2471. doi: 10.2174/13816128112092464
- Liang C, Shi S, Meng Q, et al. Complex roles of the stroma in the intrinsic resistance to gemcitabine in pancreatic cancer: Where we are and where we are going. Exp Mol Med. 2017;49(12):e406. doi: 10.1038/emm.2017.255
- Rahnama N, Jahangir M, Alesaeid S, et al. Association between microRNAs and chemoresistance in pancreatic cancer: Current knowledge, new insights, and forthcoming perspectives. Pathol Res Pract. 2022;236: 153982. doi: 10.1016/j.prp.2022.153982
- Chen Q, Wang Q, Wang Y, et al. Penetrating micelle for reversing immunosuppression and drug resistance in pancreatic cancer treatment. Small. 2022;18(18): e2107712. doi: 10.1002/smll.202107712
- Gurlin RE, Giraldo JA, Latres E. 3D bioprinting and translation of beta cell replacement therapies for type 1 diabetes. Tissue Eng Part B Rev. 2021;27(3):238-252. doi: 10.1089/ten.TEB.2020.0192
- Klak M, Kowalska P, Dobrzański T, et al. Bionic organs: Shear forces reduce pancreatic islet and mammalian cell viability during the process of 3D bioprinting. Micromachines (Basel). 2021;12(3):304. doi: 10.3390/mi12030304
- Duin S, Schütz K, Ahlfeld T, et al. 3D bioprinting of functional islets of langerhans in an alginate/methylcellulose hydrogel blend. Adv Healthc Mater. 2019;8(7):e1801631. doi: 10.1002/adhm.201801631
- Shim IK, Yi HJ, Yi HG, et al. Locally-applied 5-fluorouracil-loaded slow-release patch prevents pancreatic cancer growth in an orthotopic mouse model. Oncotarget, 2017;8(25):40140-40151. doi: 10.18632/oncotarget.17370
- Lee EM, Jung JI, Alam Z, et al. Effect of an oxygen-generating scaffold on the viability and insulin secretion function of porcine neonatal pancreatic cell clusters. Xenotransplantation. 2018;25(2):e12378. doi: 10.1111/xen.12378
- Kim J, Shim IK, Hwang DG, et al. 3D cell printing of islet-laden pancreatic tissue-derived extracellular matrix bioink constructs for enhancing pancreatic functions. J Mater Chem B. 2019;7(10):1773-1781. doi: 10.1039/c8tb02787k
- Ahn, CB, Lee, JH, et al. Development of a 3D subcutaneous construct containing insulin-producing beta cells using. bioprinting. Bio-des Manuf. 2022;5: 265-276. doi: 10.1007/s42242-021-00178-9
- Lee SJ, Lee JB, Park YW, et al. 3D bioprinting for artificial pancreas organ. Adv Exp Med Biol, 2018;1064: 355-374. doi: 10.1007/978-981-13-0445-3_21
- Wszoła M, Nitarska D, Cywoniuk P, et al. Stem cells as a source of pancreatic cells for production of 3D bioprinted bionic pancreas in the treatment of type 1 diabetes. Cells. 2021;10(6):1544. doi: 10.3390/cells10061544
- Safir F, Vu N, Tadesse LF, et al. Combining acoustic bioprinting with AI-assisted raman spectroscopy for high-throughput identification of bacteria in blood. Nano Lett. 2023;23(6):2065-2073. doi: 10.1021/acs.nanolett.2c03015
- Christou CD, Tsoulfas G. Role of three-dimensional printing and artificial intelligence in the management of hepatocellular carcinoma: Challenges and opportunities. World J Gastrointest Oncol. 2022;14(4):765-793. doi: 10.4251/wjgo.v14.i4.765
- Jiang T, Yang T, Bao Q, et al. Construction of tissue-customized hydrogels from cross-linkable materials for effective tissue regeneration. J Mater Chem B. 2022;10(25):4741-4758. doi: 10.1039/d1tb01935j
- Salg GA, Poisel E, Neulinger-Munoz M, et al. Toward 3D-bioprinting of an endocrine pancreas: A building-block concept for bioartificial insulin-secreting tissue. J Tissue Eng. 2022;13: 20417314221091033. doi: 10.1177/20417314221091033
- Farina M, Ballerini A, Fraga DW, et al. 3D printed vascularized device for subcutaneous transplantation of human islets. Biotechnol J. 2017; 12(9):1700169. doi: 10.1002/biot.201700169
- Hwang DG, Jo Y, Kim M, et al. A 3D bioprinted hybrid encapsulation system for delivery of human pluripotent stem cell-derived pancreatic islet-like aggregates. Biofabrication. 2021;14(1). doi: 10.1088/1758-5090/ac23ac
- Ravnic DJ, Leberfinger AN, Ozbolat IT. Bioprinting and cellular therapies for type 1 diabetes. Trends Biotechnol. 2017;35(11):1025-1034. doi: 10.1016/j.tibtech.2017.07.006
- Kumar SA, Delgado M, Mendez VE, et al. Applications of stem cells and bioprinting for potential treatment of diabetes. World J Stem Cells. 2019;11(1):13-32. doi: 10.4252/wjsc.v11.i1.13
- Di Piazza E, Pandolfi E, Cacciotti I, et al. Bioprinting technology in skin, heart, pancreas and cartilage tissues: Progress and challenges in clinical practice. Int J Environ Res Public Health. 2021;18(20):10806. doi: 10.3390/ijerph182010806
- Xu Y, Song D, Wang X. 3D bioprinting for pancreas engineering/ manufacturing. Polymers (Basel). 2022;14(23):5143. doi: 10.3390/polym14235143
- Huang B, Wei X, Chen K, et al. Bioprinting of hydrogel beads to engineer pancreatic tumor-stroma microtissues for drug screening. Int J Bioprint. 2023;9(3):676. doi: 10.18063/ijb.676
- Noel P, Muñoz R, Rogers GW, et al. Preparation and metabolic assay of 3-dimensional spheroid co-cultures of pancreatic cancer cells and fibroblasts. J Vis Exp. 2017;(126):56081. doi: 10.3791/56081
- Lee H. Engineering in vitro models: Bioprinting of organoids with artificial intelligence. Cyborg Bionic Syst. 2023;4: 0018. doi: 10.34133/cbsystems.0018
- Shukla P, Yeleswarapu S, Heinrich MA, et al. Mimicking tumor microenvironment by 3D bioprinting: 3D cancer modeling. Biofabrication, 2022;14(3). doi: 10.1088/1758-5090/ac6d11
- Qu J, Kalyani FS, Liu L, et al. Tumor organoids: Synergistic applications, current challenges, and future prospects in cancer therapy. Cancer Commun (Lond). 2021;41(12):1331-1353. doi: 10.1002/cac2.12224
- Fang L, Liu Y, Qiu J, et al. Bioprinting and its use in tumor-on-a-chip technology for cancer drug screening: A review. Int J Bioprint. 2022;8(4):603. doi: 10.18063/ijb.v8i4.603
- Lin M, Tang M, Duan W, et al. 3D bioprinting for tumor metastasis research. ACS Biomater Sci Eng. 2023;9(6): 3116-3133. doi: 10.1021/acsbiomaterials.3c00239
- Das R, Fernandez JG. Biomaterials for Mimicking and Modelling Tumor Microenvironment. Adv Exp Med Biol. 2022;1379:139-170. doi: 10.1007/978-3-031-04039-9_6
- Tang M, Xie Q, Gimple RC, et al. Three-dimensional bioprinted glioblastoma microenvironments model cellular dependencies and immune interactions. Cell Res. 2020;30(10):833-853. doi: 10.1038/s41422-020-0338-1
- Mi X, Su Z, Yue X, et al. 3D bioprinting tumor models mimic the tumor microenvironment for drug screening. Biomater Sci. 2023;11(11):3813-3827. doi: 10.1039/d3bm00159h
- Mao S, Pang Y, Liu T, et al. Bioprinting of in vitro tumor models for personalized cancer treatment: A review. Biofabrication, 2020;12(4):042001. doi: 10.1088/1758-5090/ab97c0
- Staros R, Michalak A, Rusinek K, et al. Perspectives for 3D-bioprinting in modeling of tumor immune evasion. Cancers (Basel), 2022;14(13):3126. doi: 10.3390/cancers14133126
- Jung M, Ghamrawi S, Du EY, et al. Advances in 3D bioprinting for cancer biology and precision medicine: From matrix design to application. Adv Healthc Mater. 2022;11(24): e2200690. doi: 10.1002/adhm.202200690
- Ringquist R, Ghoshal D, Jain R, et al. Understanding and improving cellular immunotherapies against cancer: From cell-manufacturing to tumor-immune models. Adv Drug Deliv Rev. 2021;179: 114003. doi: 10.1016/j.addr.2021.114003
- Parodi I, Di Lisa D, Pastorino L, et al. 3D bioprinting as a powerful technique for recreating the tumor microenvironment. Gels. 2023;9(6):482. doi: 10.3390/gels9060482
- Germain N, Dhayer M, Dekiouk S, et al. Current advances in 3D bioprinting for cancer modeling and personalized medicine. Int J Mol Sci. 2022;23(7):3432. doi: 10.3390/ijms23073432
- Jubelin C, Muñoz-Garcia J, Griscom L, et al. Three-dimensional in vitro culture models in oncology research. Cell Biosci. 2022;12(1):155. doi: 10.1186/s13578-022-00887-3
- Wang R, Zhang C, Li D, et al. Tumor-on-a-chip: Perfusable vascular incorporation brings new approach to tumor metastasis research and drug development. Front Bioeng Biotechnol. 2022;10: 1057913. doi: 10.3389/fbioe.2022.1057913
- Neufeld L, Yeini E, Pozzi S, et al. 3D bioprinted cancer models: From basic biology to drug development. Nat Rev Cancer. 2022;22(12):679-692. doi: 10.1038/s41568-022-00514-w
- Visalakshan RM, Lowrey MK, Sousa MGC, et al. Opportunities and challenges to engineer 3D models of tumor-adaptive immune interactions. Front Immunol. 2023;14: 1162905. doi: 10.3389/fimmu.2023.1162905
- Murphy SV, De Coppi P, Atala A. Opportunities and challenges of translational 3D bioprinting. Nat Biomed Eng. 2020;4(4):370-380. doi: 10.1038/s41551-019-0471-7
- Kronemberger GS, Miranda GASC, Tavares RSN, et al. Recapitulating tumorigenesis in vitro: Opportunities and challenges of 3D bioprinting. Front Bioeng Biotechnol. 2021;9: 682498. doi: 10.3389/fbioe.2021.682498
- Molander D, Sbirkov Y, Sarafian V. 3D bioprinting as an emerging standard for cancer modeling and drug testing. Folia Med (Plovdiv), 2022;64(4):559-565. doi: 10.3897/folmed.64.e73419
- Kang Y, Datta P, Shanmughapriya S, et al. 3D bioprinting of tumor models for cancer research. ACS Appl Bio Mater. 2020;3(9):5552-5573. doi: 10.1021/acsabm.0c00791
- Hwang DG, Choi YM, Jang J. 3D bioprinting-based vascularized tissue models mimicking tissue-specific architecture and pathophysiology for in vitro studies. Front Bioeng Biotechnol. 2021;9: 685507. doi: 10.3389/fbioe.2021.685507
- Yang H, Yang KH, Narayan RJ, et al. Laser-based bioprinting for multilayer cell patterning in tissue engineering and cancer research. Essays Biochem. 2021;65(3):409-416. doi: 10.1042/EBC20200093
- Tiwari AP, Thorat ND, Pricl S, et al. Bioink: a 3D-bioprinting tool for anticancer drug discovery and cancer management. Drug Discov Today. 2021;26(7):1574-1590. doi: 10.1016/j.drudis.2021.03.010
- Prashantha K, Krishnappa A, Muthappa M. 3D bioprinting of gastrointestinal cancer models: A comprehensive review on processing, properties, and therapeutic implications. Biointerphases. 2023;18(2):020801. doi: 10.1116/6.0002372
- Palikuqi B, Nguyen DT, Li G, et al., 2020, Adaptable haemodynamic endothelial cells for organogenesis and tumorigenesis. Nature, 585(7825):426-432. doi: 10.1038/s41586-020-2712-z
- Wang D, Guo Y, Zhu J, et al. Hyaluronic acid methacrylate/ pancreatic extracellular matrix as a potential 3D printing bioink for constructing islet organoids. Acta Biomater. 2023;165: 86-101. doi: 10.1016/j.actbio.2022.06.036
- Hakobyan D, Médina C, Dusserre N, et al. Laser-assisted 3D bioprinting of exocrine pancreas spheroid models for cancer initiation study. Biofabrication. 2020;12(3):035001. doi: 10.1088/1758-5090/ab7cb8
- Zhuang X, Deng G, Wu X, et al. Recent advances of three-dimensional bioprinting technology in hepato-pancreato-biliary cancer models. Front Oncol. 2023;13: 1143600. doi: 10.3389/fonc.2023.1143600
- Pignatelli C, Campo F, Neroni A, et al. Bioengineering the vascularized endocrine pancreas: A fine-tuned interplay between vascularization, extracellular-matrix-based scaffold architecture, and insulin-producing cells. Transpl Int. 2022;35: 10555. doi: 10.3389/ti.2022.10555
- Lee A, Hudson AR, Shiwarski DJ, et al. 3D bioprinting of collagen to rebuild components of the human heart. Science. 2019;365(6452):482-487. doi: 10.1126/science.aav9051
- Chaudhary S, Chakraborty E. Hydrogel based tissue engineering and its future applications in personalized disease modeling and regenerative therapy. Beni Suef Univ J Basic Appl Sci, 2022;11(1):3. doi: 10.1186/s43088-021-00172-1
- Hospodiuk-Karwowski M, Chi K, Pritchard J, et al. Vascularized pancreas-on-a-chip device produced using a printable simulated extracellular matrix. Biomed Mater. 2022;17(6):065006. doi: 10.1088/1748-605X/ac8c74
- Wang Z, Wang L, Li T, et al. 3D bioprinting in cardiac tissue engineering. Theranostics. 2021;11(16):7948-7969. doi: 10.7150/thno.61621
- Brandão LMS, Barbosa MS, Souza RL, et al. Lipase activation by molecular bioimprinting: The role of interactions between fatty acids and enzyme active site. Biotechnol Prog. 2021;37(1):e3064. doi: 10.1002/btpr.3064
- Manoukian P, Bijlsma M, Van Laarhoven H. The cellular origins of cancer-associated fibroblasts and their opposing contributions to pancreatic cancer growth. Front Cell Dev Biol. 2021;9: 743907. doi: 10.3389/fcell.2021.743907
- Bengtsson A, Andersson R, Rahm J, et al. Organoid technology for personalized pancreatic cancer therapy. Cell Oncol (Dordr). 2021;44(2):251-260. doi: 10.1007/s13402-021-00585-1
- Melzer MK, Resheq Y, Navaee F, et al. The application of pancreatic cancer organoids for novel drug discovery. Expert Opin Drug Discov. 2023;18(4):429-444. doi: 10.1080/17460441.2023.2194627
- Sun H, Wang Y, Yang H. Revolutionizing preclinical research for pancreatic cancer: the potential of 3D bioprinting technology for personalized therapy. Hepatobiliary Surg Nutr. 2023;12(4):616-618. doi: 10.21037/hbsn-23-248
- Monteiro MV, Ferreira LP, Rocha M, et al. Advances in bioengineering pancreatic tumor-stroma physiomimetic biomodels. Biomaterials; 2022;287:121653. doi: 10.1016/j.biomaterials.2022.121653
- Swayden M, Soubeyran P, Iovanna J. Upcoming revolutionary paths in preclinical modeling of pancreatic adenocarcinoma. Front Oncol. 2020;9: 1443. doi: 10.3389/fonc.2019.01443
- Banda Sánchez C, Cubo Mateo N, Saldaña L, et al. Selection and optimization of a bioink based on PANC-1- plasma/ alginate/methylcellulose for pancreatic tumour modelling. Polymers (Basel). 2023;15(15):3196. doi: 10.3390/polym15153196
- Xue W, Yu SY, Kuss M, et al. 3D bioprinted white adipose model for in vitro study of cancer-associated cachexia induced adipose tissue remodeling. Biofabrication. 2022;14(3). doi: 10.1088/1758-5090/ac6c4b
- De Barros NR, Gomez A, Ermis M, et al. Gelatin methacryloyl and Laponite bioink for 3D bioprinted organotypic tumor modeling. Biofabrication. 2023;15(4). doi: 10.1088/1758-5090/ace0db
- Utama RH, Tan VTG, Tjandra KC, et al. A covalently crosslinked ink for multimaterials drop-on-demand 3D bioprinting of 3D cell cultures. Macromol Biosci. 2021;21(9): e2100125. doi: 10.1002/mabi.202100125
- Freeman S, Calabro S, Williams R, et al. Bioink formulation and machine learning-empowered bioprinting optimization. Front Bioeng Biotechnol, 2022;10: 913579. doi: 10.3389/fbioe.2022.913579
- Wang J, Cui Z, Maniruzzaman M. Bioprinting: A focus on improving bioink printability and cell performance based on different process parameters. Int J Pharm. 2023:640: 123020. doi: 10.1016/j.ijpharm.2023.123020
- Kupfer ME, Lin WH, Ravikumar V, et al. In situ expansion, differentiation, and electromechanical coupling of human cardiac muscle in a 3D bioprinted, chambered organoid. Circ Res. 2020;127(2):207-224. doi: 10.1161/CIRCRESAHA.119.316155
- Banerjee D, Singh YP, Datta P, et al. Strategies for 3D bioprinting of spheroids: A comprehensive review. Biomaterials. 2022;291: 121881. doi: 10.1016/j.biomaterials.2022.121881
- Asim S, Tabish TA, Liaqat U, et al. Advances in gelatin bioinks to optimize bioprinted cell functions. Adv Healthc Mater. 2023;12(17):e2203148. doi: 10.1002/adhm.202203148