Cathepsin proteases and cancer progression: A niche for dual-acting anticancer therapeutics
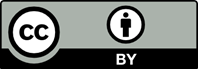
Cathepsin proteases have captivated the scientific community for many years owing to their deregulated expression, activation status, and potential role in the proliferation and progression of specific cancer types. These efforts have led to the development of several therapeutic strategies targeting specific cathepsin proteases. This development has been further driven by the identification of important cathepsin protease-specific target proteins. Despite the limitations of these approaches, primarily due to the lack of known specificity of cathepsin proteases in targeting substrate proteins, an alternative strategy may involve the identification and development of dual-acting therapeutics. We propose that these dual-acting therapeutics can selectively interfere with the protein constituents involved in forming a cathepsin-targeted protein complex while simultaneously inhibiting the cognate cathepsin protease. Considering this novel paradigm, we evaluated the potential of dual-acting therapeutics against other well-documented mimetic therapeutics designed to target intermediates in the apoptosis pathway. Some of these therapeutics may represent promising candidates for further testing as dual-acting anticancer therapeutics, either alone or in combination regimens.
- Cao M, Luo X, Wu K, He X. Targeting lysosomes in human disease: From basic research to clinical applications. Signal Transduct Target Ther. 2021;6(1):379. doi: 10.1038/s41392-021-00778-y
- Eskelinen EL, Tanaka Y, Saftig P. At the acidic edge: Emerging functions for lysosomal membrane proteins. Trends Cell Biol. 2003;13(3):137-145. doi: 10.1016/s0962-8924(03)00005-9
- Olson OC, Joyce JA. Cysteine cathepsin proteases: Regulators of cancer progression and therapeutic response. Nat Rev Cancer. 2015;15(12):712-729. doi: 10.1038/nrc4027
- Soond SM, Kozhevnikova MV, Zamyatnin AA Jr. “Patchiness” and basic cancer research: Unravelling the proteases. Cell Cycle. 2019;18(15):1687-1701. doi: 10.1080/15384101.2019.1632639
- Uhlman A, Folkers K, Liston J, Pancholi H, Hinton A. Effects of vacuolar H+-ATPase inhibition on activation of cathepsin B and cathepsin L secreted from MDA-MB231 breast cancer cells. Cancer Microenviron. 2017;10(1-3):49-56. doi: 10.1007/s12307-017-0196-7
- Rothberg JM, Bailey KM, Wojtkowiak JW, et al. Acid-mediated tumor proteolysis: Contribution of cysteine cathepsins. Neoplasia. 2013;15(10):1125-1137. doi: 10.1593/neo.13946
- Rozhin J, Sameni M, Ziegler G, Sloane BF. Pericellular pH affects distribution and secretion of cathepsin B in malignant cells. Cancer Res. 1994;54(24):6517-6525.
- Soond SM, Kozhevnikova MV, Frolova AS, et al. Lost or Forgotten: The nuclear cathepsin protein isoforms in cancer. Cancer Lett. 2019;462:43-50. doi: 10.1016/j.canlet.2019.07.020
- Jordans S, Jenko-Kokalj S, Kuhl NM, et al. Monitoring compartment-specific substrate cleavage by cathepsins B, K, L, and S at physiological pH and redox conditions. BMC Biochem. 2009;10:23. doi: 10.1186/1471-2091-10-23
- Turk B, Turk D, Turk V. Lysosomal cysteine proteases: More than scavengers. Biochim Biophys Acta. 2000;1477(1-2):98-111. doi: 10.1016/s0167-4838(99)00263-0
- Soond SM, Zamyatnin AA Jr. Helicobacter pylori and gastric cancer: A lysosomal protease perspective. Gastric Cancer. 2022;25(2):306-324. doi: 10.1007/s10120-021-01272-8
- Soond SM, Kozhevnikova MV, Savvateeva LV, Townsend PA, Zamyatnin AA Jr. Intrinsically connected: Therapeutically targeting the cathepsin proteases and the Bcl-2 family of protein substrates as co-regulators of apoptosis. Int J Mol Sci. 2021;22(9):4669. doi: 10.3390/ijms22094669
- De Castro MA, Bunt G, Wouters FS. Cathepsin B launches an apoptotic exit effort upon cell death-associated disruption of lysosomes. Cell Death Discov. 2016;2:16012. doi: 10.1038/cddiscovery.2016.12
- Turk B, Dolenc I, Turk V, Bieth JG, Kinetics of the pH-induced inactivation of human cathepsin L. Biochemistry. 1993;32(1):375-380. doi: 10.1021/bi00052a046
- Turk B, Bieth JG, Bjork I, et al. Regulation of the activity of lysosomal cysteine proteinases by pH-induced inactivation and/or endogenous protein inhibitors, cystatin. Biol Chem Hoppe Seyler. 1995;376(4):225-230. doi: 10.1515/bchm3.1995.376.4.225
- Edgington LE, Verdoes M, Bogyo M. Functional imaging of proteases: Recent advances in the design and application of substrate-based and activity-based probes. Curr Opin Chem Biol. 2011;15(6):798-805. doi: 10.1016/j.cbpa.2011.10.012
- Jain M, Bouilloux J, Borrego I, et al. Cathepsin B-cleavable polymeric photosensitizer prodrug for selective photodynamic therapy: In vitro studies. Pharmaceuticals (Basel). 2022;15(5):564. doi: 10.3390/ph15050564
- Chowdhury MA, Moya IA, Bhilocha S, et al. Prodrug-inspired probes selective to cathepsin B over other cysteine cathepsins. J Med Chem. 2014;57(14):6092-6104. doi: 10.1021/jm500544p
- Soond SM, Kozhevnikova MV, Townsend PA, Zamyatnin AA Jr. Cysteine cathepsin protease inhibition: An update on its diagnostic, prognostic and therapeutic potential in cancer. Pharmaceuticals (Basel). 2019;12(2):87. doi: 10.3390/ph12020087
- Podobnik M, Kuhelj R, Turk V, Turk D. Crystal structure of the wild-type human procathepsin B at 2.5 A resolution reveals the native active site of a papain-like cysteine protease zymogen. J Mol Biol. 1997;271(5):774-788. doi: 10.1006/jmbi.1997.1218
- Renko M, Pozgan U, Majera D, Turk D. Stefin A displaces the occluding loop of cathepsin B only by as much as required to bind to the active site cleft. FEBS J. 2010;277(20):4338-4345. doi: 10.1111/j.1742-4658.2010.07824.x
- Cory S, Adams JM. The Bcl2 family: Regulators of the cellular life-or-death switch. Nat Rev Cancer. 2002;2(9):647-656. doi: 10.1038/nrc883
- Ola MS, Nawaz M, Ahsan H. Role of Bcl-2 family proteins and caspases in the regulation of apoptosis. Mol Cell Biochem. 2011;351(1-2):41-58. doi: 10.1007/s11010-010-0709-x
- Garcia-Saez AJ. The secrets of the Bcl-2 family. Cell Death Differ. 2012;19(11):1733-1740. doi: 10.1038/cdd.2012.105
- Youle RJ, Strasser A. The BCL-2 protein family: Opposing activities that mediate cell death. Nat Rev Mol Cell Biol. 2008;9(1):47-59. doi: 10.1038/nrm2308
- Huang DC, Adams JM, Cory S. The conserved N-terminal BH4 domain of Bcl-2 homologues is essential for inhibition of apoptosis and interaction with CED-4. EMBO J. 1998;17(4):1029-1039. doi: 10.1093/emboj/17.4.1029
- Kvansakul M, Yang H, Fairlie WD, et al. Vaccinia virus anti-apoptotic F1L is a novel Bcl-2-like domain-swapped dimer that binds a highly selective subset of BH3-containing death ligands. Cell Death Differ. 2008;15(10):1564-1571. doi: 10.1038/cdd.2008.83
- Townsend PA, Kozhevnikova MV, Cexus ONF, Zamyatnin AA Jr., Soond SM. BH3-mimetics: Recent developments in cancer therapy. J Exp Clin Cancer Res. 2021;40(1):355. doi: 10.1186/s13046-021-02157-5
- Lomonosova E, Chinnadurai G. BH3-only proteins in apoptosis and beyond: An overview. Oncogene. 2008;27(Suppl 1):S2-S19. doi: 10.1038/onc.2009.39
- Flores-Romero H, Ros U, Garcia-Saez AJ. Pore formation in regulated cell death. EMBO J. 2020;39(23):e105753. doi: 10.15252/embj.2020105753
- Li P, Nijhawan D, Budihardjo I, et al. Cytochrome c and dATP-dependent formation of Apaf-1/caspase-9 complex initiates an apoptotic protease cascade. Cell. 1997;91(4):479-489. doi: 10.1016/s0092-8674(00)80434-1
- Delbridge AR, Strasser A. The BCL-2 protein family, BH3-mimetics and cancer therapy. Cell Death Differ. 2015;22(7):1071-1080. doi: 10.1038/cdd.2015.50
- Merino D, Kelly GL, Lessene G, Wei AH, Roberts AW, Strasser A. BH3-mimetic drugs: Blazing the trail for new cancer medicines. Cancer Cell. 2018;34(6):879-891. doi: 10.1016/j.ccell.2018.11.004
- Turk V, Stoka V, Vasiljeva O, et al. Cysteine cathepsins: From structure, function and regulation to new frontiers. Biochim Biophys Acta. 2012;1824(1):68-88. doi: 10.1016/j.bbapap.2011.10.002
- Pogorzelska A, Zolnowska B, Bartoszewski R. Cysteine cathepsins as a prospective target for anticancer therapies-current progress and prospects. Biochimie. 2018;51:85-106. doi: 10.1016/j.biochi.2018.05.023
- Kozlowski L, Rucinska M, Wojtukiewicz MZ. Metastases of malignant melanoma of unknown primary site: An important diagnostic and therapeutic problem. Pol Merkur Lekarski. 2000;8(49):486-488.
- Sloane BF, Dunn JR, Honn KV. Lysosomal cathepsin B: Correlation with metastatic potential. Science. 1981;212(4499):1151-1153. doi: 10.1126/science.7233209
- Dian D, Vrekoussis T, Shabani N, et al. Expression of cathepsin-D in primary breast cancer and corresponding local recurrence or metastasis: An immunohistochemical study. Anticancer Res. 2012;32(3):901-905.
- Fujise N, Nanashim A, Taniguchi Y, et al. Prognostic impact of cathepsin B and matrix metalloproteinase-9 in pulmonary adenocarcinomas by immunohistochemical study. Lung Cancer. 2000;27(1):19-26. doi: 10.1016/s0169-5002(99)00088-4
- Pruitt FL, He Y, Franco OE, Jiang M, Cates JM, Hayward SW. Cathepsin D acts as an essential mediator to promote malignancy of benign prostatic epithelium. Prostate. 2013;73(5):476-488. doi: 10.1002/pros.22589
- Krueger S, Kalinski T, Wolf H, Kellner U, Roessner A. Interactions between human colon carcinoma cells, fibroblasts and monocytic cells in coculture--regulation of cathepsin B expression and invasiveness. Cancer Lett. 2005;223(2):313-322. doi: 10.1016/j.canlet.2004.09.050
- Sivaparvathi M, Sawaya R, Gokaslan ZL, Chintala SK, Rao JS. Expression and the role of cathepsin H in human glioma progression and invasion. Cancer Lett. 1996;104(1):121-126. doi: 10.1016/0304-3835(96)04242-5
- Kleer CG, Bloushtain-Qimron N, Chen YH, et al. Epithelial and stromal cathepsin K and CXCL14 expression in breast tumor progression. Clin Cancer Res. 2008;14(17):5357-5367. doi: 10.1158/1078-0432.CCR-08-0732
- Gocheva V, Zeng W, Ke D, et al. Distinct roles for cysteine cathepsin genes in multistage tumorigenesis. Genes Dev. 2006;20(5):543-556. doi: 10.1101/gad.1407406
- Jedeszko C, Sloane BF. Cysteine cathepsins in human cancer. Biol Chem. 2004;385(11):1017-1027. doi: 10.1515/BC.2004.132
- Ebert MP, Kruger S, Fogeron ML, et al. Overexpression of cathepsin B in gastric cancer identified by proteome analysis. Proteomics. 2005;5(6):1693-1704. doi: 10.1002/pmic.200401030
- Bengsch F, Buck A, Gunther SC, et al. Cell type-dependent pathogenic functions of overexpressed human cathepsin B in murine breast cancer progression. Oncogene. 2014;33(36):4474-4484. doi: 10.1038/onc.2013.395
- Qin G, Cai Y, Long J, et al. Cathepsin L is involved in proliferation and invasion of breast cancer cells. Neoplasma. 2016;63(1):30-36. doi: 10.4149/neo_2016_004
- Wang W, Long L, Wang L, et al. Knockdown of Cathepsin L promotes radiosensitivity of glioma stem cells both in vivo and in vitro. Cancer Lett. 2016;71(2):274-284. doi: 10.1016/j.canlet.2015.12.012
- Mehrotra S, Wickremesekera SK, Brasch HD, et al. Expression and localization of cathepsins B, D and G in cancer stem cells in liver metastasis from colon adenocarcinoma. Front Surg. 2018;5:40. doi: 10.3389/fsurg.2018.00040
- Featherston T, Marsh RW, van Schaijik B, Brasch HD, Tan ST, Itinteang T. Expression and localization of cathepsins B, D, and G in two cancer stem cell subpopulations in moderately differentiated oral tongue squamous cell carcinoma. Front Med (Lausanne). 2017;4:100. doi: 10.3389/fmed.2017.00100
- Breznik B, Limbaeck Stokin C, Kos J, et al. Cysteine cathepsins B, X and K expression in peri-arteriolar glioblastoma stem cell niches. J Mol Histol. 2018;9(5):481-497. doi: 10.1007/s10735-018-9787-y
- Sui H, Shi C, Yan Z, Wu M. Overexpression of Cathepsin L is associated with chemoresistance and invasion of epithelial ovarian cancer. Oncotarget. 2016;(29):45995-46001. doi: 10.18632/oncotarget.10276
- Zhang H, Zhang L, Wei L, et al. Knockdown of cathepsin L sensitizes ovarian cancer cells to chemotherapy. Oncol Lett. 2016;11(6):4235-4239. doi: 10.3892/ol.2016.4494
- Han ML, Zhao YF, Tan CH, et al. Cathepsin L upregulation-induced EMT phenotype is associated with the acquisition of cisplatin or paclitaxel resistance in A549 cells. Acta Pharmacol Sin. 2016;37(12):1606-1622. doi: 10.1038/aps.2016.93
- Reiser J, Adair B, Reinheckel T. Specialized roles for cysteine cathepsins in health and disease. J Clin Invest. 2010;120(10):3421-3431. doi: 10.1172/JCI42918
- Sukhova GK, Shi GP, Simon DI, Chapman HA, Libby P. Expression of the elastolytic cathepsins S and K in human atheroma and regulation of their production in smooth muscle cells. J Clin Invest. 1998;102(3):576-583. doi: 10.1172/JCI181
- Godat E, Herve-Grvepinet V, Veillard F, et al. Regulation of cathepsin K activity by hydrogen peroxide. Biol Chem. 2008;389(8):1123-1126. doi: 10.1515/BC.2008.109
- Vizovisek M, Fonovic M, Turk B. Cysteine cathepsins in extracellular matrix remodeling: Extracellular matrix degradation and beyond. Matrix Biol. 2019;75-76:141-159. doi: 10.1016/j.matbio.2018.01.024
- Gocheva V, Joyce JA. Cysteine cathepsins and the cutting edge of cancer invasion. Cell Cycle. 2007;6(1):60-64. doi: 10.4161/cc.6.1.3669
- Fonovic M, Turk B. Cysteine cathepsins and extracellular matrix degradation. Biochim Biophys Acta. 2014;1840(8):2560-25670. doi: 10.1016/j.bbagen.2014.03.017
- Li Z, Yasuda Y, Li W, et al. Regulation of collagenase activities of human cathepsins by glycosaminoglycans. J Biol Chem. 2004;279(7):5470-5479. doi: 10.1074/jbc.M310349200
- Repnik U, Starr AE, Overall CM, Turk B. Cysteine cathepsins activate ELR chemokines and inactivate Non- ELR chemokines. J Biol Chem. 2015;290(22):13800-13811. doi: 10.1074/jbc.M115.638395
- Breznik B, Motaln H, Lah Turnsek T. Proteases and cytokines as mediators of interactions between cancer and stromal cells in tumours. Biol Chem. 2017;98(7):709-719. doi: 10.1515/hsz-2016-0283
- Ohashi K, Naruto M, Nakaki T, Sano E. Identification of interleukin-8 converting enzyme as cathepsin L. Biochim Biophys Acta. 2003;1649(1):30-39. doi: 10.1016/s1570-9639(03)00152-3
- Hira VV, Verbovsek U, Breznik B, et al. Cathepsin K cleavage of SDF-1alpha inhibits its chemotactic activity towards glioblastoma stem-like cells. Biochim Biophys Acta Mol Cell Res. 2017;1864(3):594-603. doi: 10.1016/j.bbamcr.2016.12.021
- Robotic B, Vizovisek M, Vidmar R, et al. Proteomic identification of cysteine cathepsin substrates shed from the surface of cancer cells. Mol Cell Proteomics. 2015;4(8): 2213-2228. doi: 10.1074/mcp.M114.044628
- Clark AK, Yip PK, Malcangio M. The liberation of fractalkine in the dorsal horn requires microglial cathepsin S. J Neurosci. 2009;29(21):6945-6954. doi: 10.1523/JNEUROSCI.0828-09.2009
- Mohamed MM, Sloane BF. Cysteine cathepsins: Multifunctional enzymes in cancer. Nat Rev Cancer. 2006;6(10):764-775. doi: 10.1038/nrc1949
- Caglic D, Pungercar JR, Pejler G, Turk V, Turk B. Glycosaminoglycans facilitate procathepsin B activation through disruption of propeptide-mature enzyme interactions. J Biol Chem. 2007;282(45):33076-33085. doi: 10.1074/jbc.M705761200
- Ruettger A, Schueler S, Mollenhauer JA, Wiederanders B. Cathepsins B, K, and L are regulated by a defined collagen type II peptide via activation of classical protein kinase C and p38 MAP kinase in articular chondrocytes. J Biol Chem. 2008;283(2):1043-1051. doi: 10.1074/jbc.M704915200
- Rampino N, Yamamoto H, Ionov Y, et al. Somatic frameshift mutations in the BAX gene in colon cancers of the microsatellite mutator phenotype. Science. 1997;275(5302):967-969. doi: 10.1126/science.275.5302.967
- Zhang M, Zheng J, Nussinov R, Ma B. Oncogenic mutations differentially affect bax monomer, dimer, and oligomeric pore formation in the membrane. Sci Rep. 2016;6:33340. doi: 10.1038/srep33340
- Zhang Z, Zhu W, Lapolla SM, et al. Bax forms an oligomer via separate, yet interdependent, surfaces. J Biol Chem. 2010;285(23):17614-17627. doi: 10.1074/jbc.M110.113456
- Shibata MA, Liu ML, Knudson MC, et al. Haploid loss of bax leads to accelerated mammary tumor development in C3(1)/SV40-TAg transgenic mice: Reduction in protective apoptotic response at the preneoplastic stage. EMBO J. 1999;18(10):2692-2701. doi: 10.1093/emboj/18.10.2692
- Knudson CM, Tung KS, Tourtellotte WG, Brown GA, Korsmeyer SJ. Bax-deficient mice with lymphoid hyperplasia and male germ cell death. Science. 1995;270(5233):96-99. doi: 10.1126/science.270.5233.96
- Lindsten T, Ross AJ, King A, et al. The combined functions of proapoptotic Bcl-2 family members bak and bax are essential for normal development of multiple tissues. Mol Cell. 2000;6(6):1389-1399. doi: 10.1016/s1097-2765(00)00136-2
- Fannjiang Y, Kim CH, Huganir RL, et al. BAK alters neuronal excitability and can switch from anti-to pro-death function during postnatal development. Dev Cell. 2003;4(4):575-585. doi: 10.1016/s1534-5807(03)00091-1
- Wei MC, Zong WX, Cheng EH, et al. Proapoptotic BAX and BAK: A requisite gateway to mitochondrial dysfunction and death. Science. 2001;292(5517):727-730. doi: 10.1126/science.1059108
- Jaaskelainen M, Nieminen A, Pokkyla RM, et al. Regulation of cell death in human fetal and adult ovaries--role of Bok and Bcl-X(L). Mol Cell Endocrinol. 2010;330(1-2):17-24. doi: 10.1016/j.mce.2010.07.020
- Ke F, Voss A, Kerr JB, et al. BCL-2 family member BOK is widely expressed but its loss has only minimal impact in mice. Cell Death Differ. 2012;19(6):915-925. doi: 10.1038/cdd.2011.210
- Suominen JS, Yan W, Toppari J, Kaipia A. The expression and regulation of Bcl-2-related ovarian killer (Bok) mRNA in the developing and adult rat testis. Eur J Endocrinol. 2001;145(6):771-778. doi: 10.1530/eje.0.1450771
- Cao X, Deng X, May WS. Cleavage of Bax to p18 Bax accelerates stress-induced apoptosis, and a cathepsin-like protease may rapidly degrade p18 Bax. Blood. 2003;102(7):2605-2614. doi: 10.1182/blood-2003-01-0211
- Jurgensmeier JM, Xie Z, Deveraux Q, Ellerby L, Bredesen D, Reed JC. Bax directly induces release of cytochrome c from isolated mitochondria. Proc Natl Acad Sci U S A. 1998;95(9):4997-5002. doi: 10.1073/pnas.95.9.4997
- Droga-Mazovec G, Bojic L, Petelin A, et al. Cysteine cathepsins trigger caspase-dependent cell death through cleavage of bid and antiapoptotic Bcl-2 homologs. J Biol Chem. 2008;283(27):19140-19150. doi: 10.1074/jbc.M802513200
- Soond SM, Savvateeva LV, Makarov VA, Gorokhovets NV, Townsend PA, Zamyatnin AA Jr. Cathepsin S cleaves BAX as a novel and therapeutically important regulatory mechanism for apoptosis. Pharmaceutics. 2021;13(3):339. doi: 10.3390/pharmaceutics13030339
- Bruncko M, Oost TK, Belli BA, et al. Studies leading to potent, dual inhibitors of Bcl-2 and Bcl-xL. J Med Chem. 2007;50(4):641-662. doi: 10.1021/jm061152t
- Oltersdorf T, Elmore SW, Shoemaker AR, et al. An inhibitor of Bcl-2 family proteins induces regression of solid tumours. Nature. 2005;435(7042):677-681. doi: 10.1038/nature03579
- Delbridge AR, Grabow S, Strasser A, Vaux DL. Thirty years of BCL-2: Translating cell death discoveries into novel cancer therapies. Nat Rev Cancer. 2016;16(2):99-109. doi: 10.1038/nrc.2015.17
- Gaussian N, Frontczak A, El Kaddissi A, et al. Systemic treatment of locally advanced or metastatic penile cancer. Bull Cancer. 2020;107(5S):S17-S23. doi: 10.1016/S0007-4551(20)30274-5
- Kaiser U, Schilli M, Haag U, et al. Expression of bcl-2--protein in small cell lung cancer. Lung Cancer. 1996;15(1):31-40. doi: 10.1016/0169-5002(96)00568-5
- Beroukhim R, Mermel CH, Porter D, et al. The landscape of somatic copy-number alteration across human cancers. Nature. 2010;463(7283):899-905. doi: 10.1038/nature08822
- Leverson JD, Phillips DC, Mitten MJ, et al. Exploiting selective BCL-2 family inhibitors to dissect cell survival dependencies and define improved strategies for cancer therapy. Sci Transl Med. 2015;7(279):279ra40. doi: 10.1126/scitranslmed.aaa4642
- Punnoose EA, Leverson JD, Peale F, et al. Expression profile of BCL-2, BCL-XL, and MCL-1 predicts pharmacological response to the BCL-2 selective antagonist venetoclax in multiple myeloma models. Mol Cancer Ther. 2016;15(5):1132-1144. doi: 10.1158/1535-7163.MCT-15-0730
- Motoyama N, Wang F, Roth KA, et al. Massive cell death of immature hematopoietic cells and neurons in Bcl-x-deficient mice. Science. 1995;267(5203):1506-1510. doi: 10.1126/science.7878471
- Veis DJ, Sorenson CM, Shutter JR, Korsmeyer SJ. Bcl-2- deficient mice demonstrate fulminant lymphoid apoptosis, polycystic kidneys, and hypopigmented hair. Cell. 1993;75(2):229-240. doi: 10.1016/0092-8674(93)80065-m
- Appelqvist H, Johansson AC, Linderoth E, et al. Lysosome-mediated apoptosis is associated with cathepsin D-specific processing of bid at Phe24, Trp48, and Phe183. Ann Clin Lab Sci. 2012;42(3):231-242.
- Cirman T, Oresic K, Mazovec GD, et al. Selective disruption of lysosomes in HeLa cells triggers apoptosis mediated by cleavage of Bid by multiple papain-like lysosomal cathepsins. J Biol Chem. 2004;279(5):3578-3587. doi: 10.1074/jbc.M308347200
- Li H, Zhu H, Xu CJ, Yuan J. Cleavage of BID by caspase 8 mediates the mitochondrial damage in the Fas pathway of apoptosis. Cell. 1998;94(4):491-501. doi: 10.1016/s0092-8674(00)81590-1
- Luo X, Budihardjo I, Zou H, Slaughter C, Wang X. Bid, a Bcl2 interacting protein, mediates cytochrome c release from mitochondria in response to activation of cell surface death receptors. Cell. 1998;94(4):481-490. doi: 10.1016/s0092-8674(00)81589-5
- Gross A, Yin XM, Wang K, et al. Caspase cleaved BID targets mitochondria and is required for cytochrome c release, while BCL-XL prevents this release but not tumor necrosis factor-R1/Fas death. J Biol Chem. 1999;274(2):1156-1163. doi: 10.1074/jbc.274.2.1156
- Slee EA, Keogh SA, Martin SJ. Cleavage of BID during cytotoxic drug and UV radiation-induced apoptosis occurs downstream of the point of Bcl-2 action and is catalyzed by caspase-3: A potential feedback loop for amplification of apoptosis-associated mitochondrial cytochrome c release. Cell Death Differ. 2000;7(6):556-565. doi: 10.1038/sj.cdd.4400689
- Eskes R, Desagher S, Antonsson B, Martinou JC. Bid induces the oligomerization and insertion of Bax into the outer mitochondrial membrane. Mol Cell Biol. 2000;20(3):929-935. doi: 10.1128/MCB.20.3.929-935.2000
- Wei MC, Lindsten T, Mootha VK, et al. tBID, a membrane-targeted death ligand, oligomerizes BAK to release cytochrome c. Genes Dev. 2000;14(16):2060-2071.
- Reiners JJ Jr., Caruso JA, Mathieu P, Chelladurai B, Yin XM, Kessel D. Release of cytochrome c and activation of pro-caspase-9 following lysosomal photodamage involves Bid cleavage. Cell Death Differ. 2002;9(9):934-944. doi: 10.1038/sj.cdd.4401048
- Stoka V, Turk B, Schendel SL, et al. Lysosomal protease pathways to apoptosis. Cleavage of bid, not pro-caspases, is the most likely route. J Biol Chem. 2001;276(5):3149-3157. doi: 10.1074/jbc.M008944200
- Blomgran R, Zheng L, Stendahl O. Cathepsin-cleaved Bid promotes apoptosis in human neutrophils via oxidative stress-induced lysosomal membrane permeabilization. J Leukoc Biol. 2007;81(5):1213-1223. doi: 10.1189/jlb.0506359
- Egle A, Harris AW, Bouillet P, Cory S. Bim is a suppressor of Myc-induced mouse B cell leukemia. Proc Natl Acad Sci U S A. 2004;101(16):6164-6169. doi: 10.1073/pnas.0401471101
- Tan TT, Degenhardt K, Nelson DA, et al. Key roles of BIM-driven apoptosis in epithelial tumors and rational chemotherapy. Cancer Cell. 2005;7(3):227-238. doi: 10.1016/j.ccr.2005.02.008
- Sattler M, Liang H, Nettesheim D, et al. Structure of Bcl-xL-Bak peptide complex: recognition between regulators of apoptosis. Science. 1997;275(5302):983-986. doi: 10.1126/science.275.5302.983
- Lessene G, Czabotar PE, Colman PM. BCL-2 family antagonists for cancer therapy. Nat Rev Drug Discov. 2008;7(12):989-1000. doi: 10.1038/nrd2658
- Wendt MD, Shen W, Kunzer A, et al. Discovery and structure-activity relationship of antagonists of B-cell lymphoma 2 family proteins with chemopotentiation activity in vitro and in vivo. J Med Chem. 2006;49(3):1165-1181. doi: 10.1021/jm050754u
- Tse C, Shoemaker AR, Adickes J, et al. ABT-263: A potent and orally bioavailable Bcl-2 family inhibitor. Cancer Res. 2008;68(9):3421-3428. doi: 10.1158/0008-5472.CAN-07-5836
- Wilson WH, O’Connor OA, Czuczman MS, et al. Navitoclax, a targeted high-affinity inhibitor of BCL-2, in lymphoid malignancies: A phase 1 dose-escalation study of safety, pharmacokinetics, pharmacodynamics, and antitumour activity. Lancet Oncol. 2010;11(12):1149-1159. doi: 10.1016/S1470-2045(10)70261-8
- Roberts AW, Seymour JF, Brown JR, et al. Substantial susceptibility of chronic lymphocytic leukemia to BCL2 inhibition: Results of a phase I study of navitoclax in patients with relapsed or refractory disease. J Clin Oncol. 2012;30(5):488-496. doi: 10.1200/JCO.2011.34.7898
- Rudin CM, Hann CL, Garon EB, et al. Phase II study of single-agent navitoclax (ABT-263) and biomarker correlates in patients with relapsed small cell lung cancer. Clin Cancer Res. 2012;18(11):3163-3169. doi: 10.1158/1078-0432.CCR-11-3090
- Kipps TJ, Eradat H, Grosicki S, et al. A phase 2 study of the BH3 mimetic BCL2 inhibitor navitoclax (ABT- 263) with or without rituximab, in previously untreated B-cell chronic lymphocytic leukemia. Leuk Lymphoma. 2015;56(10):2826-2833. doi: 10.3109/10428194.2015.1030638
- Debrincat MA, Pleines I, Lebois M, et al. BCL-2 is dispensable for thrombopoiesis and platelet survival. Cell Death Dis. 2015;6(4):e1721. doi: 10.1038/cddis.2015.97
- Mason KD, Carpinelli MR, Fletcher JI, et al. Programmed anuclear cell death delimits platelet life span. Cell. 2007;128(6):1173-1186. doi: 10.1016/j.cell.2007.01.037
- Roberts AW, Davids MS, Pagel JM, et al. Targeting BCL2 with venetoclax in relapsed chronic lymphocytic leukemia. N Engl J Med. 2016;374(4):311-322. doi: 10.1056/NEJMoa1513257
- Souers AJ, Leverson JD, Boghaert ER, et al. ABT-199, a potent and selective BCL-2 inhibitor, achieves antitumor activity while sparing platelets. Nat Med. 2013;19(2):202-208. doi: 10.1038/nm.3048
- Stilgenbauer S, Eichhorst B, Schetelig J, et al. Venetoclax in relapsed or refractory chronic lymphocytic leukaemia with 17p deletion: A multicentre, open-label, phase 2 study. Lancet Oncol. 2016;17(6):768-778. doi: 10.1016/S1470-2045(16)30019-5
- Tahir SK, Smith ML, Hessler P, et al. Potential mechanisms of resistance to venetoclax and strategies to circumvent it. BMC Cancer. 2017;17(1):399. doi: 10.1186/s12885-017-3383-5
- Koehler MF, Bergeron P, Choo EF, et al. Structure-guided rescaffolding of selective antagonists of BCL-XL. ACS Med Chem Lett. 2014;5(6):662-667. doi: 10.1021/ml500030p
- Lessene G, Czabotar PE, Sleebs BE, et al. Structure-guided design of a selective BCL-X(L) inhibitor. Nat Chem Biol. 2013;9(6):390-397. doi: 10.1038/nchembio.1246
- Tao ZF, Hasvold L, Wang L, et al. Discovery of a potent and selective BCL-XL inhibitor with in vivo activity. ACS Med Chem Lett. 2014;5(10):1088-1093. doi: 10.1021/ml5001867
- Wei G, Margolin AA, Haery L, et al. Chemical genomics identifies small-molecule MCL1 repressors and Bcl-xL as a predictor of MCL1 dependency. Cancer Cell. 2012;21(4): 547-562. doi: 10.1016/j.ccr.2012.02.028
- Wertz IE, Kusam S, Lam C, et al. Sensitivity to antitubulin chemotherapeutics is regulated by MCL1 and FBW7. Nature. 2011;471(7336):110-114. doi: 10.1038/nature09779
- Lee EF, Czabotar PE, van Delft MF, et al. A novel BH3 ligand that selectively targets Mcl-1 reveals that apoptosis can proceed without Mcl-1 degradation. J Cell Biol. 2008;180(2):341-355. doi: 10.1083/jcb.200708096
- Lee EF, Czabotar PE, Yang H, et al. Conformational changes in Bcl-2 pro-survival proteins determine their capacity to bind ligands. J Biol Chem. 2009;284(44):30508-30517. doi: 10.1074/jbc.M109.040725
- Nakao S, Zandi S, Sun D,Hafezi-Moghadam A. Cathepsin B-mediated CD18 shedding regulates leukocyte recruitment from angiogenic vessels. FASEB J. 2018;32(1):143-154. doi: 10.1096/fj.201601229R
- Oskarsson T. Extracellular matrix components in breast cancer progression and metastasis. Breast. 2013;22(Suppl 2):S66-S72. doi: 10.1016/j.breast.2013.07.012
- Wang B, Sun J, Kitamoto S, et al. Cathepsin S controls angiogenesis and tumor growth via matrix-derived angiogenic factors. J Biol Chem. 2006;281(9):6020-6029. doi: 10.1074/jbc.M509134200
- Guinec N, Dalet-Fumeron V, Pagano M. “In vitro” study of basement membrane degradation by the cysteine proteinases, cathepsins B, B-like and L. Digestion of collagen IV, laminin, fibronectin, and release of gelatinase activities from basement membrane fibronectin. Biol Chem Hoppe Seyler. 1993;374(12):1135-1146. doi: 10.1515/bchm3.1993.374.7-12.1135
- Mai J, Sameni M, Mikkelsen T, Sloane BF. Degradation of extracellular matrix protein tenascin-C by cathepsin B: An interaction involved in the progression of gliomas. Biol Chem. 2002;383(9):1407-1413. doi: 10.1515/BC.2002.159
- Felbor U, Dreier L, Bryant RA, Ploegh HL, Olsen BR, Mothes W. Secreted cathepsin L generates endostatin from collagen XVIII. EMBO J. 2000;19(6):1187-1194. doi: 10.1093/emboj/19.6.1187
- Veillard F, Saidi A, Burden RE et al. Cysteine cathepsins S and L modulate anti-angiogenic activities of human endostatin. J Biol Chem. 2011;286(43):37158-37167. doi: 10.1074/jbc.M111.284869
- Gineyts E, Bonnet N, Bertholon C, et al. The C-terminal intact forms of periostin (iPTN) are surrogate markers for osteolytic lesions in experimental breast cancer bone metastasis. Calcif Tissue Int. 2018;103(5):567-580. doi: 10.1007/s00223-018-0444-y
- Podgorski I, Linebaugh BE, Koblinski JE, et al. Bone marrow-derived cathepsin K cleaves SPARC in bone metastasis. Am J Pathol. 2009;175(3):1255-1269. doi: 10.2353/ajpath.2009.080906
- Park CM, Oie T, Petros AM, et al. Design, synthesis, and computational studies of inhibitors of Bcl-xL. J Am Chem Soc. 2006;128(50):16206-16212. doi: 10.1021/ja0650347
- Wang G, Nikolovska-Coleska Z, Yang CY, et al. Structure-based design of potent small-molecule inhibitors of anti-apoptotic Bcl-2 proteins. J Med Chem. 2006;49(21):6139-6142. doi: 10.1021/jm060460o
- Nguyen M, Marcellus RC, Roulston A, et al. Small molecule obatoclax (GX15-070) antagonizes MCL-1 and overcomes MCL-1-mediated resistance to apoptosis. Proc Natl Acad Sci U S A. 2007;104(49):19512-19517. doi: 10.1073/pnas.0709443104
- Li L, Thomas RM, Suzuki H, De Brabander JK, Wang X, Harran PG. A small molecule Smac mimic potentiates TRAIL- and TNFalpha-mediated cell death. Science. 2004;305(5689):1471-1474. doi: 10.1126/science.1098231
- Bai L, Chen W, Chen W, Wang X, Tang H, Lin Y. IKKbeta-mediated nuclear factor-kappaB activation attenuates smac mimetic-induced apoptosis in cancer cells. Mol Cancer Ther. 2009;8(6):1636-1645. doi: 10.1158/1535-7163.MCT-09-0068
- Lu J, Bai L, Sun H, et al. SM-164: A novel, bivalent Smac mimetic that induces apoptosis and tumor regression by concurrent removal of the blockade of cIAP-1/2 and XIAP. Cancer Res. 2008;68(22):9384-9393. doi: 10.1158/0008-5472.CAN-08-2655
- Cai Q, Sun H, Peng Y, et al. A potent and orally active antagonist (SM-406/AT-406) of multiple inhibitor of apoptosis proteins (IAPs) in clinical development for cancer treatment. J Med Chem. 2011;54(8):2714-2726. doi: 10.1021/jm101505d
- Weisberg E, Ray A, Barrett R, et al. Smac mimetics: Implications for enhancement of targeted therapies in leukemia. Leukemia. 2010;24(12):2100-2109. doi: 10.1038/leu.2010.212
- Sun H, Nikolovska-Coleska Z, Lu J, et al. Design, synthesis, and characterization of a potent, nonpeptide, cell-permeable, bivalent Smac mimetic that concurrently targets both the BIR2 and BIR3 domains in XIAP. J Am Chem Soc. 2007;129(49):15279-15294. doi: 10.1021/ja074725f
- Allensworth JL, Sauer SJ, Lyerly HK, Morse MA, Devi GR. Smac mimetic Birinapant induces apoptosis and enhances TRAIL potency in inflammatory breast cancer cells in an IAP-dependent and TNF-alpha-independent mechanism. Breast Cancer Res Treat. 2013;137(2):359-371. doi: 10.1007/s10549-012-2352-6
- Leverson JD, Zhang H, Chen J, et al. Potent and selective small-molecule MCL-1 inhibitors demonstrate on-target cancer cell killing activity as single agents and in combination with ABT-263 (navitoclax). Cell Death Dis. 2015;6(1):e1590. doi: 10.1038/cddis.2014.561
- Bruncko M, Wang L, Sheppard GS, et al. Structure-guided design of a series of MCL-1 inhibitors with high affinity and selectivity. J Med Chem. 2015;58(5):2180-2194. doi: 10.1021/jm501258m
- Caenepeel S, Brown SP, Belmontes B, et al. AMG 176, a selective MCL1 inhibitor, is effective in hematologic cancer models alone and in combination with established therapies. Cancer Discov. 2018;8(12):1582-1597. doi: 10.1158/2159-8290.CD-18-0387
- Tron AE, Belmonte MA, Adam A, et al. Discovery of Mcl-1-specific inhibitor AZD5991 and preclinical activity in multiple myeloma and acute myeloid leukemia. Nat Commun. 2018;9(1):5341. doi: 10.1038/s41467-018-07551-w
- Kotschy A, Szlavik Z, Murray J, et al. The MCL1 inhibitor S63845 is tolerable and effective in diverse cancer models. Nature. 2016;538(7626):477-482. doi: 10.1038/nature19830
- Stewart ML, Fire E, Keating AE, Walensky LD. The MCL-1 BH3 helix is an exclusive MCL-1 inhibitor and apoptosis sensitizer. Nat Chem Biol. 2010;6(8):595-601. doi: 10.1038/nchembio.391
- Ramsey HE, Fischer MA, Lee T, et al. A novel MCL1 inhibitor combined with venetoclax rescues venetoclax-resistant acute myelogenous leukemia. Cancer Discov. 2018;8(12):1566-1581. doi: 10.1158/2159-8290.CD-18-0140
- Lee T, Bian Z, Zhao B, et al. Discovery and biological characterization of potent myeloid cell leukemia-1 inhibitors. FEBS Lett. 2017;591(1):240-251. doi: 10.1002/1873-3468.12497
- Folkes AJ, Ahmadi K, Alderton WK, et al. The identification of 2-(1H-indazol-4-yl)-6-(4-methanesulfonyl-piperazin- 1-ylmethyl)-4-morpholin-4-yl-thieno[3,2-d]pyrimidine (GDC-0941) as a potent, selective, orally bioavailable inhibitor of class I PI3 kinase for the treatment of cancer. J Med Chem. 2008;51(18):5522-5532. doi: 10.1021/jm800295d
- Zamyatnin AA Jr., Gregory LC, Townsend PA, Soond SM. Beyond basic research: The contribution of cathepsin B to cancer development, diagnosis and therapy. Expert Opin Ther Targets. 2022;26(11):963-977. doi: 10.1080/14728222.2022.2161888