A novel signature identified by pyroptosis-related lncRNAs clusters predicts prognosis and tumor immune microenvironment for pediatric acute myeloid leukemia
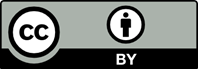
Pyroptosis is a recently discovered programmed cell death that is involved in tumor formation, prognosis, and curative effect. Pyroptosis-related long non-coding ribonucleic acids (PR-lncRNAs) play key roles in tumorigenesis and tumor progression. However, the inherent relationship between PR-lncRNAs and the prognosis of pediatric acute myeloid leukemia (AML) remains unclear. For this reason, the association of PR-lncRNAs with the prognosis and tumor microenvironment features was analyzed using the Therapeutically Applicable Research to Generate Effective Treatment (TARGET) database. We classified three clusters based on PR-lncRNAs expressions and identified 841 differentially expressed PR-lncRNAs related to overall survival time. Seven key lncRNAs were then identified by least absolute shrinkage and selection operator (LASSO)-Cox and multivariate Cox. A signature based on these seven lncRNAs was also established, and the patients were separated into two groups according to their risk score. The high-risk group was characterized by poorer prognosis, lower expression of immune checkpoints, lower microsatellite instability or microsatellite stability (MSI-L/MSS), and lower drug sensitivity. The results demonstrated that PR-lncRNAs have a potential effect on the tumor immune microenvironment, clinicopathological features, and prognosis in pediatric AML. The nomogram and decision curve analysis suggested that the risk score is one of the most accurate of any other and provided a basis for the exploration of the immune microenvironment of the cell pyroptosis-associated subtypes in children with AML and the construction of a prognostic model with seven key PR-lncRNAs, which provides an approach to evaluate the prognosis of pediatric AML patients.
Quessada J, Cuccuini W, Saultier P, et al., 2021, Cytogenetics of pediatric acute myeloid leukemia: A review of the current knowledge (in eng). Genes (Basel), 12(6): 924. https://doi.org/10.3390/genes12060924
Lejman M, Dziatkiewicz I, Jurek M, 2022, Straight to the point-the novel strategies to cure pediatric AML (in eng). Int J Mol Sci, 23(4): 1968. https://doi.org/10.3390/ijms23041968
Egan G, Chopra Y, Mourad S, et al., 2021, Treatment of acute myeloid leukemia in children: A practical perspective (in eng). Pediatr Blood Cancer, 68(7): e28979. https://doi.org/10.1002/pbc.28979
Kuwatsuka Y, Tomizawa D, Kihara R, et al., 2018, Prognostic value of genetic mutations in adolescent and young adults with acute myeloid leukemia (in eng). Int J Hematol, 107(2): 201–210. https://doi.org/10.1007/s12185-017-2340-z
Hsu SK, Li CY, Lin IL, et al., 2021, Inflammation-related pyroptosis, a novel programmed cell death pathway, and its crosstalk with immune therapy in cancer treatment (in eng). Theranostics, 11(18): 8813–8835. https://doi.org/10.7150/thno.62521
Li L, Jiang M, Qi L, et al., 2021, Pyroptosis, a new bridge to tumor immunity (in eng). Cancer Sci, 112(10): 3979–3994. https://doi.org/10.1111/cas.15059
Fang Y, Tian S, Pan Y, et al., 2020, Pyroptosis: A new frontier in cancer (in eng). Biomed Pharmacother, 121: 109595. https://doi.org/10.1016/j.biopha.2019.109595
Xia X, Wang X, Cheng Z, et al., 2019, The role of pyroptosis in cancer: Pro-cancer or pro-“host”? (in eng). Cell Death Dis, 10(9): 650. https://doi.org/10.1038/s41419-019-1883-8
Johnson DC, Taabazuing CY, Okondo MC, et al., 2018, DPP8/DPP9 inhibitor-induced pyroptosis for treatment of acute myeloid leukemia (in eng). Nat Med, 24(8): 1151–1156. https://doi.org/10.1038/s41591-018-0082-y
Razmara M, Srinivasula SM, Wang L, et al., 2002, CARD-8 protein, a new CARD family member that regulates caspase-1 activation and apoptosis (in eng). J Biol Chem, 277(16): 13952–13958. https://doi.org/10.1074/jbc.M107811200
Luo X, Zhang X, Gan L, et al., 2018, The outer membrane protein Tp92 of Treponema pallidum induces human mononuclear cell death and IL-8 secretion (in eng). J Cell Mol Med, 22(12): 6039–6054. https://doi.org/10.1111/jcmm.13879
Bhan A, Soleimani M, Mandal SS, 2017, Long noncoding RNA and cancer: A new paradigm (in eng). Cancer Res, 77(15): 3965–3981. https://doi.org/10.1158/0008-5472.Can-16-2634
Bridges MC, Daulagala AC, Kourtidis A, 2021, LNCcation: lncRNA localization and function (in eng). J Cell Biol, 220(2): e202009045. https://doi.org/10.1083/jcb.202009045
He D, Zheng J, Hu J, et al., 2020, Long non-coding RNAs and pyroptosis (in eng). Clin Chim Acta, 504: 201–208. https://doi.org/10.1016/j.cca.2019.11.035
Weber CE, Kuo PC, 2012, The tumor microenvironment (in eng). Surg Oncol, 21(3): 172–177. https://doi.org/10.1016/j.suronc.2011.09.001
Newman AM, Liu CL, Green MR, et al., 2015, Robust enumeration of cell subsets from tissue expression profiles (in eng). Nat Methods, 12(5): 453–457. https://doi.org/10.1038/nmeth.3337
Ohaegbulam KC, Assal A, Lazar-Molnar E, et al., 2015, Human cancer immunotherapy with antibodies to the PD-1 and PD-L1 pathway (in eng). Trends Mol Med, 21(1): 24–33. https://doi.org/10.1016/j.molmed.2014.10.009
Rowshanravan B, Halliday N, Sansom DM, 2018, CTLA-4: A moving target in immunotherapy (in eng). Blood, 131(1): 58–67. https://doi.org/10.1182/blood-2017-06-741033
Maruhashi T, Sugiura D, Okazaki IM, et al., 2020, LAG-3: From molecular functions to clinical applications (in eng). J Immunother Cancer, 8(2): e001014. https://doi.org/10.1136/jitc-2020-001014
Zang K, Hui L, Wang M, et al., 2021, TIM-3 as a prognostic marker and a potential immunotherapy target in human malignant tumors: A meta-analysis and bioinformatics validation (in eng). Front Oncol, 11: 579351. https://doi.org/10.3389/fonc.2021.579351
Li L, Feng Q, Wang X, 2020, PreMSIm: An R package for predicting microsatellite instability from the expression profiling of a gene panel in cancer (in eng). Comput Struct Biotechnol J, 18: 668–675. https://doi.org/10.1016/j.csbj.2020.03.007
Garzon R, Volinia S, Papaioannou D, et al., 2014, Expression and prognostic impact of lncRNAs in acute myeloid leukemia (in eng). Proc Natl Acad Sci U S A, 111(52): 18679– 18684. https://doi.org/10.1073/pnas.1422050112
Liu J, Geng R, Ni S, et al., 2022, Pyroptosis-related lncRNAs are potential biomarkers for predicting prognoses and immune responses in patients with UCEC (in eng). Mol Ther Nucleic Acids, 27: 1036–1055. https://doi.org/10.1016/j.omtn.2022.01.018
Ping L, Zhang K, Ou X, et al., 2021, A novel pyroptosis-associated long non-coding RNA signature predicts prognosis and tumor immune microenvironment of patients with breast cancer (in eng). Front Cell Dev Biol, 9: 727183. https://doi.org/10.3389/fcell.2021.727183
Guo M, Xiao ZD, Dai Z, et al., 2020, The landscape of long noncoding RNA-involved and tumor-specific fusions across various cancers (in eng). Nucleic Acids Res, 48(22): 12618–12631. https://doi.org/10.1093/nar/gkaa1119
Lu Z, Tang F, Li Z, et al., 2022, Prognosis risk model based on pyroptosis-related lncRNAs for bladder cancer (in eng). Dis Markers, 2022: 7931393. https://doi.org/10.1155/2022/7931393
Aran D, Sirota M, Butte AJ, 2015, Systematic pan-cancer analysis of tumour purity (in eng). Nat Commun, 6: 8971. https://doi.org/10.1038/ncomms9971
Abril-Rodriguez G, RibasA, 2017, SnapShot: Immune checkpoint inhibitors (in eng). Cancer Cell, 31(6): 848–848.e1.https://doi.org/10.1016/j.ccell.2017.05.010
Vago L, Gojo I, 2020, Immune escape and immunotherapy of acute myeloid leukemia (in eng). J Clin Invest, 130(4): 1552–1564. https://doi.org/10.1172/jci129204
Jiang F, Wang XY, WangMY, et al., 2021, An immune checkpoint-related gene signature for predicting survival of pediatric acute myeloid leukemia (in eng). J Oncol, 2021: 5550116. https://doi.org/10.1155/2021/55501106
He R, Wu S, Gao R, et al., 2021, Identification of a long noncoding RNA TRAF3IP2-AS1 as key regulator of IL-17 signaling through the SRSF10-IRF1-Act1 axis in autoimmune diseases (in eng). J Immunol, 206(10): 2353–2365. https://doi.org/10.4049/jimmunol.2001223
Han Y, Ye A, Bi L, et al., 2014, Th17 cells and interleukin-17 increase with poor prognosis in patients with acute myeloid leukemia (in eng). Cancer Sci, 105(8): 933–942. https://doi.org/10.1111/cas.12459
Wróbel T, Mazur G, Jazwiec B, et al., 2003, Interleukin-17 in acute myeloid leukemia (in eng). J Cell Mol Med, 7(4): 472–474. https://doi.org/10.1111/j.1582-4934.2003.tb00250.x
Li J, Zhang J, Tao S, et al., 2022, Prognostication of pancreatic cancer using the cancer genome atlas based ferroptosis-related long non-coding RNAs (in eng). Front Genet, 13: 838021. https://doi.org/10.3389/fgene.2022.838021
Zhong F, Yao F, Cheng Y, et al., 2022, m6A-related lncRNAs predict prognosis and indicate immune microenvironment in acute myeloid leukemia (in eng). Sci Rep, 12(1): 1759. https://doi.org/10.1038/s41598-022-05797-5
Ni W, Mo H, Liu Y, et al., 2021, Targeting cholesterol biosynthesis promotes anti-tumor immunity by inhibiting long noncoding RNA SNHG29-mediated YAP activation (in eng). Mol Ther, 29(10): 2995–3010. https://doi.org/10.1016/j.ymthe.2021.05.012
Chen C, Liang C, Wang S, et al., 2020, Expression patterns of immune checkpoints in acute myeloid leukemia (in eng). J Hematol Oncol, 13(1): 28. https://doi.org/10.1186/s13045-020-00853-x
Han L, Li Z, Jiang Y, et al., 2019, SNHG29 regulates miR- 223-3p/CTNND1 axis to promote glioblastoma progression via Wnt/β-catenin signaling pathway (in eng). Cancer Cell Int, 19: 345. https://doi.org/10.1186/s12935-019-1057-x
Li S, Wu D, Jia H, et al., 2020, Long non-coding RNA LRRC75A-AS1 facilitates triple negative breast cancer cell proliferation and invasion via functioning as a ceRNA to modulate BAALC (in eng). Cell Death Dis, 11(8): 643. https://doi.org/10.1038/s41419-020-02821-2
Wu L, Liao W, Wang X, et al., 2021, Expression, prognosis value, and immune infiltration of lncRNA ASB16-AS1 identified by pan-cancer analysis (in eng). Bioengineered, 12(2): 10302–10318. https://doi.org/10.1080/21655979.2021.1996054
Bosman MC, Schepers H, Jaques J, et al., 2014, The TAK1- NF-κB axis as a therapeutic target for AML (in eng). Blood, 124(20): 3130–3140. https://doi.org/10.1182/blood-2014-04-569780
Fu T, Ji K, Jin L, et al., 2021, ASB16-AS1 up-regulated and phosphorylated TRIM37 to activate NF-κB pathway and promote proliferation, stemness, and cisplatin resistance of gastric cancer (in eng). Gastric Cancer, 24(1): 45–59. https://doi.org/10.1007/s10120-020-01096-y
Zhou JG, Liang B, Liu JG, et al., 2021, Identification of 15 lncRNAs signature for predicting survival benefit of advanced melanoma patients treated with Anti-PD-1 monotherapy (in eng). Cells, 10(5): 977. https://doi.org/10.3390/cells10050977
Rezaei M, Tan J, Zeng C, et al., 2021, TIM-3 in leukemia; Immune response and beyond (in eng). Front Oncol, 11: 753677. https://doi.org/10.3389/fonc.2021.753677
Kikushige Y, Akashi K, 2012, TIM-3 as a therapeutic target for malignant stem cells in acute myelogenous leukemia (in eng). Ann N Y Acad Sci, 1266: 118–123. https://doi.org/10.1111/j.1749-6632.2012.06550.x
Du T, Gao J, Li P, et al., 2021, Pyroptosis, metabolism, and tumor immune microenvironment (in eng) Clin Transl Med, 11(8): e492. https://doi.org/10.1002/ctm2.492
Park EG, Pyo SJ, Cui Y, et al., 2022, Tumor immune microenvironment lncRNAs (in eng). Brief Bioinform, 23(1): bbab504. https://doi.org/10.1093/bib/bbab504