High expression of apoptosis-related LMNB2 predicts an unfavorable outcome: A potential prognostic biomarker for liposarcoma
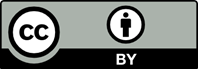
Liposarcoma (LPS) is the most prevalent soft-tissue sarcoma and the second most common malignant mesenchymal sarcoma. Molecular markers have proven instrumental in guiding the diagnosis, prognosis, and treatment strategies for LPS patients. Identifying potential therapeutic targets is essential for developing effective intervention strategies for LPS. LMNB2, an apoptosis-related gene, exhibits associations with various tumors. Therefore, exploring the feasibility of LMNB2 as a prognostic biomarker for LPS is crucial. After screening for differentially expressed genes (DEGs), which were analyzed by GEO2R, 14 apoptosis-related genes were obtained by overlapping DEGs from the GSE21122 and GSE159659 datasets. SPSS software was used for univariate analysis. Receiver operating characteristic curves were constructed by GraphPad software to compare the expression of LMNB2 between LPS and normal tissues. Kaplan–Meier curves were generated to verify the correlation between LMNB2 expression and survival time. GeneMANIA and STRING were used to construct LMNB2-related gene-gene and protein–protein interaction networks. Hiplot software facilitated function and pathway enrichment analysis to determine the potential mechanism of LMNB2-mediated LPS progression. CIBERSORT was used to evaluate the correlation between LMNB2 expression and immune cell infiltration. The expression level of LMNB2 was significantly higher in LPS, and the high expression of LMNB2 was significantly related to poor prognosis in LPS patients. Further analysis indicated that LMNB2 was mainly involved in “senescence” and “apoptosis,” further confirming its role in regulating the occurrence and development of LPS by modulating the cell cycle progression and apoptosis. This study demonstrates that the elevated expression of LMNB2 is significantly associated with poor prognostic outcomes in LPS, suggesting that LMNB2 holds high potential as a new biomarker for LPS. This study is designed to elucidate the potential mechanism of LMNB2-mediated LPS progression, with the prospect of improving therapeutic development by identifying LMNB2 as a promising prognostic biomarker for LPS.
- Cissé MY, Pyrdziak S, Firmin N, et al. Targeting MDM2- dependent serine metabolism as a therapeutic strategy for liposarcoma. Sci Transl Med. 2020;12(547):eaay2163. doi: 10.1126/scitranslmed.aay2163
- Lee ATJ, Thway K, Huang PH, Jones RL. Clinical and molecular spectrum of liposarcoma. J Clin Oncol. 2018;36(2):151-159. doi: 10.1200/jco.2017.74.9598
- Choi JH, Ro JY. The 2020 WHO classification of tumors of soft tissue: Selected changes and new entities. Adv Anat Pathol. 2021;28(1):44-58. doi: 10.1097/pap.0000000000000284
- Haddox CL, Riedel RF. Recent advances in the understanding and management of liposarcoma. Fac Rev. 2021;10:1. doi: 10.12703/r/10-1
- Creytens D, Folpe AL, Koelsche C, et al. Myxoid pleomorphic liposarcoma-a clinicopathologic, immunohistochemical, molecular genetic and epigenetic study of 12 cases, suggesting a possible relationship with conventional pleomorphic liposarcoma. Mod Pathol. 2021;34(11):2043-2049. doi: 10.1038/s41379-021-00862-2
- Crago AM, Dickson MA. Liposarcoma: Multimodality management and future targeted therapies. Surg Oncol Clin N Am. 2016;25(4):761-773. doi: 10.1016/j.soc.2016.05.007
- Yang L, Chen S, Luo P, Yan W, Wang C. Liposarcoma: Advances in cellular and molecular genetics alterations and corresponding clinical treatment. J Cancer. 2020;11(1):100-107. doi: 10.7150/jca.36380
- Nassif NA, Tseng W, Borges C, Chen P, Eisenberg B. Recent advances in the management of liposarcoma. F1000Res. 2016;5:2907. doi: 10.12688/f1000research.10050.1
- Keung EZ, Akdemir KC, Al Sannaa GA, et al. Increased H3K9me3 drives dedifferentiated phenotype via KLF6 repression in liposarcoma. J Clin Invest. 2015;125(8):2965-2978. doi: 10.1172/jci77976
- Damiano JA, Afawi Z, Bahlo M, et al. Mutation of the nuclear lamin gene LMNB2 in progressive myoclonus epilepsy with early ataxia. Hum Mol Genet. 2015;24(16):4483-4490. doi: 10.1093/hmg/ddv171
- Evangelisti C, Rusciano I, Mongiorgi S, et al. The wide and growing range of lamin B-related diseases: From laminopathies to cancer. Cell Mol Life Sci. 2022;79(2):126. doi: 10.1007/s00018-021-04084-2
- Dechat T, Adam SA, Goldman RD. Nuclear lamins and chromatin: When structure meets function. Adv Enzyme Regul. 2009;49(1):157-166. doi: 10.1016/j.advenzreg.2008.12.003
- Capell BC, Collins FS. Human laminopathies: Nuclei gone genetically awry. Nat Rev Genet. 2006;7(12):940-952. doi: 10.1038/nrg1906
- Worman HJ, Bonne G. “Laminopathies”: A wide spectrum of human diseases. Exp Cell Res. 2007;313(10):2121-2133. doi: 10.1016/j.yexcr.2007.03.028
- Guinde J, Frankel D, Perrin S, et al. Lamins in lung cancer: Biomarkers and key factors for disease progression through miR-9 regulation? Cells. 2018;7(7):78. doi: 10.3390/cells7070078
- Kong W, Wu Z, Yang M, Zuo X, Yin G, Chen W. LMNB2 is a prognostic biomarker and correlated with immune infiltrates in hepatocellular carcinoma. IUBMB Life. 2020;72(12):2672-2685. doi: 10.1002/iub.2408
- Prasad S, Soldatenkov VA, Srinivasarao G, Dritschilo A. Intermediate filament proteins during carcinogenesis and apoptosis (Review). Int J Oncol. 1999;14(3):563-570. doi: 10.3892/ijo.14.3.563
- Monier B, Suzanne M. Orchestration of force generation and nuclear collapse in apoptotic cells. Int J Mol Sci. 2021;22(19):10257. doi: 10.3390/ijms221910257
- Mu W, Guo L, Liu Y, Yang H, Ning S, Lv G. Long noncoding RNA SNHG1 regulates LMNB2 expression by sponging miR-326 and promotes cancer growth in hepatocellular carcinoma. Front Oncol. 2021;11:784067. doi: 10.3389/fonc.2021.784067
- Dong CH, Jiang T, Yin H, et al. LMNB2 promotes the progression of colorectal cancer by silencing p21 expression. Cell Death Dis. 2021;12(4):331. doi: 10.1038/s41419-021-03602-1
- Robin JD, Magdinier F. Physiological and pathological aging affects chromatin dynamics, structure and function at the nuclear edge. Front Genet. 2016;7:153. doi: 10.3389/fgene.2016.00153
- Porter AG, Ng P, Jänicke RU. Death substrates come alive. BioEssays. 1997;19(6):501-507. doi: 10.1002/bies.950190609
- Méndez-López I, Blanco-Luquin I, Sánchez-Ruiz de Gordoa J, et al. Hippocampal LMNA gene expression is increased in late-stage Alzheimer’s disease. Int J Mol Sci. 2019;20(4):878. doi: 10.3390/ijms20040878
- Stephens AD, Banigan EJ, Marko JF. Chromatin’s physical properties shape the nucleus and its functions. Curr Opin Cell Biol. 2019;58:76-84. doi: 10.1016/j.ceb.2019.02.006
- Parry DA, Martin CA, Greene P, et al. Heterozygous lamin B1 and lamin B2 variants cause primary microcephaly and define a novel laminopathy. Genet Med. 2021;23(2):408-414. doi: 10.1038/s41436-020-00980-3
- Ma Y, Fei L, Zhang M, et al. Lamin B2 binding to minichromosome maintenance complex component 7 promotes non-small cell lung carcinogenesis. Oncotarget. 2017;8(62):104813-104830. doi: 10.18632/oncotarget.20338
- Zhao CC, Chen J, Zhang LY, Liu H, Zhang CG, Liu Y. Lamin B2 promotes the progression of triple negative breast cancer via mediating cell proliferation and apoptosis. Biosci Rep. 2021;41(1):BSR20203874. doi: 10.1042/BSR20203874
- Ji J, Li H, Chen J, Wang W. Lamin B2 contributes to the proliferation of bladder cancer cells via activating the expression of cell division cycle-associated protein 3. Int J Mol Med. 2022;50(3):111. doi: 10.3892/ijmm.2022.5168
- Bu D, Luo H, Huo P, et al. KOBAS-i: Intelligent prioritization and exploratory visualization of biological functions for gene enrichment analysis. Nucleic Acids Res. 2021;49(W1):W317-W325. doi: 10.1093/nar/gkab447
- Huang DW, Sherman BT, Tan Q, et al. The DAVID gene functional classification tool: A novel biological module-centric algorithm to functionally analyze large gene lists. Genome Biol. 2007;8(9):R183. doi: 10.1186/gb-2007-8-9-r183
- Xie C, Mao X, Huang J, et al. KOBAS 2.0: A web server for annotation and identification of enriched pathways and diseases. Nucleic Acids Res. 2011;39:W316-W322. doi: 10.1093/nar/gkr483
- Xu JH, Hu SL, Shen GD, Shen G. Tumor suppressor genes and their underlying interactions in paclitaxel resistance in cancer therapy. Cancer Cell Int. 2016;16:13. doi: 10.1186/s12935-016-0290-9
- Franz M, Rodriguez H, Lopes C, et al. GeneMANIA update 2018. Nucleic Acids Res. 2018;46(W1):W60-W64. doi: 10.1093/nar/gky311
- Warde-Farley D, Donaldson SL, Comes O, et al. The GeneMANIA prediction server: Biological network integration for gene prioritization and predicting gene function. Nucleic Acids Res. 2010;38:W214-W220. doi: 10.1093/nar/gkq537
- Szklarczyk D, Morris JH, Cook H, et al. The STRING database in 2017: Quality-controlled protein-protein association networks, made broadly accessible. Nucleic Acids Res. 2017;45(D1):D362-D368.doi: 10.1093/nar/gkw937
- Szklarczyk D, Gable AL, Nastou KC, et al. The STRING database in 2021: Customizable protein-protein networks, and functional characterization of user-uploaded gene/measurement sets. Nucleic Acids Res. 2021;49(D1):D605-D612. doi: 10.1093/nar/gkaa1074
- Chen B, Khodadoust MS, Liu CL, Newman AM, Alizadeh AA. Profiling tumor infiltrating immune cells with CIBERSORT. Methods Mol Biol. 2018;1711:243-259. doi: 10.1007/978-1-4939-7493-1_12
- Craven KE, Gökmen-Polar Y, Badve SS. CIBERSORT analysis of TCGA and METABRIC identifies subgroups with better outcomes in triple negative breast cancer. Sci Rep. 2021;11(1):4691. doi: 10.1038/s41598-021-83913-7
- Kawada JI, Takeuchi S, Imai H, et al. Immune cell infiltration landscapes in pediatric acute myocarditis analyzed by CIBERSORT. J Cardiol. 2021;77(2):174-178. doi: 10.1016/j.jjcc.2020.08.004
- Gouda MA. Common pitfalls in reporting the use of SPSS software. Med Princ Pract. 2015;24(3):300. doi: 10.1159/000381953
- Willcox CR, Vantourout P, Salim M, et al. Butyrophilin-like 3 directly binds a human Vγ4+ T Cell receptor using a modality distinct from clonally-restricted antigen. Immunity. 2019;51(5):813-825.e4. doi: 10.1016/j.immuni.2019.09.006
- Yeo J, Ko M, Lee DH, Park Y, Jin HS. TIGIT/CD226 axis regulates anti-tumor immunity. Pharmaceuticals (Basel). 2021;14(3):200. doi: 10.3390/ph14030200
- Starzer AM, Berghoff AS. New emerging targets in cancer immunotherapy: CD27 (TNFRSF7). ESMO Open. 2020;4(Suppl 3):e000629. doi: 10.1136/esmoopen-2019-000629
- Tang T, Cheng X, Truong B, Sun L, Yang X, Wang H. Molecular basis and therapeutic implications of CD40/CD40L immune checkpoint. Pharmacol Ther. 2021;219:107709. doi: 10.1016/j.pharmthera.2020.107709
- Odobasic D, Kitching AR, Tipping PG, Holdsworth SR. CD80 and CD86 costimulatory molecules regulate crescentic glomerulonephritis by different mechanisms. Kidney Int. 2005;68(2):584-594. doi: 10.1111/j.1523-1755.2005.00436.x
- Solinas C, Gu-Trantien C, Willard-Gallo K. The rationale behind targeting the ICOS-ICOS ligand costimulatory pathway in cancer immunotherapy. ESMO Open. 2020;5(1):e000544. doi: 10.1136/esmoopen-2019-000544
- Chen L, Flies DB. Molecular mechanisms of T cell co-stimulation and co-inhibition. Nat Rev Immunol. 2013;13(4):227-242. doi: 10.1038/nri3405
- Tian J, Zhang B, Rui K, Wang S. The role of GITR/GITRL interaction in autoimmune diseases. Front Immunol. 2020;11:588682. doi: 10.3389/fimmu.2020.588682
- Song X, Zhou Z, Li H, et al. Pharmacologic suppression of B7-H4 glycosylation restores antitumor immunity in immune-cold breast cancers. Cancer Discov. 2020;10(12):1872-1893. doi: 10.1158/2159-8290.Cd-20-0402
- Bock S, Hoffmann DG, Jiang Y, Chen H, Il’yasova D. Increasing incidence of liposarcoma: A population-based study of national surveillance databases, 2001-2016. Int J Environ Res Public Health. 2020;17(8):2710. doi: 10.3390/ijerph17082710
- Bartlett EK, Curtin CE, Seier K, et al. Histologic subtype defines the risk and kinetics of recurrence and death for primary extremity/truncal liposarcoma. Ann Surg. 2021;273(6):1189-1196. doi: 10.1097/sla.0000000000003453
- Zheng K, Yu XC, Xu M, Yang Y. Surgical outcomes and prognostic factors of myxoid liposarcoma in extremities: A retrospective study. Orthop Surg. 2019;11(6):1020-1028. doi: 10.1111/os.12566
- Bill KL, Casadei L, Prudner BC, Iwenofu H, Strohecker AM, Pollock RE. Liposarcoma: Molecular targets and therapeutic implications. Cell Mol Life Sci. 2016;73(19):3711-3718. doi: 10.1007/s00018-016-2266-2
- Thway K. Well-differentiated liposarcoma and dedifferentiated liposarcoma: An updated review. Semin Diagn Pathol. 2019;36(2):112-121. doi: 10.1053/j.semdp.2019.02.006
- Schöffski P. Established and experimental systemic treatment options for advanced liposarcoma. Oncol Res Treat. 2022;45(9):525-543. doi: 10.1159/000524939
- Noguchi R, Yoshimatsu Y, Ono T, et al. Establishment and characterization of NCC-PLPS1-C1, a novel patient-derived cell line of pleomorphic liposarcoma. Human Cell. 2021;34(2):688-697. doi: 10.1007/s13577-020-00457-0
- Zhou MY, Bui NQ, Charville GW, Ganjoo KN, Pan M. Treatment of de-differentiated liposarcoma in the era of immunotherapy. Int J Mol Sci. 2023;24(11):9571. doi: 10.3390/ijms24119571
- Qin H, Lu Y, Du L, et al. Pan-cancer analysis identifies LMNB1 as a target to redress Th1/Th2 imbalance and enhance PARP inhibitor response in human cancers. Cancer Cell Int. 2022;22(1):101. doi: 10.1186/s12935-022-02467-4
- Endo-Munoz L, Dahler A, Teakle N, et al. E2F7 can regulate proliferation, differentiation, and apoptotic responses in human keratinocytes: Implications for cutaneous squamous cell carcinoma formation. Cancer Res. 2009;69(5):1800-1808. doi: 10.1158/0008-5472.Can-08-2725
- Zong S, Liu X, Zhou N, Yue Y. E2F7, EREG, miR-451a and miR-106b-5p are associated with the cervical cancer development. Arch Gynecol Obstet. 2019;299(4):1089-1098. doi: 10.1007/s00404-018-5007-y
- Kim LK, Park SA, Eoh KJ, Heo TH, Kim YT, Kim HJ. E2F8 regulates the proliferation and invasion through epithelial-mesenchymal transition in cervical cancer. Int J Biol Sci. 2020;16(2):320-329. doi: 10.7150/ijbs.37686
- Iino K, Mitobe Y, Ikeda K, et al. RNA-binding protein NONO promotes breast cancer proliferation by post-transcriptional regulation of SKP2 and E2F8. Cancer Sci. 2020;111(1):148-159. doi: 10.1111/cas.14240
- Yang R, Wang M, Zhang G, et al. E2F7-EZH2 axis regulates PTEN/AKT/mTOR signalling and glioblastoma progression. Br J Cancer. 2020;123(9):1445-1455. doi: 10.1038/s41416-020-01032-y
- Wajant H. Therapeutic targeting of CD70 and CD27. Expert Opin Ther Targets. 2016;20(8):959-973. doi: 10.1517/14728222.2016.1158812
- Chen DS, Mellman I. Oncology meets immunology: The cancer-immunity cycle. Immunity. 2013;39(1):1-10. doi: 10.1016/j.immuni.2013.07.012
- Rotte A. Combination of CTLA-4 and PD-1 blockers for treatment of cancer. J Exp Clin Cancer Res. 2019;38(1):255. doi: 10.1186/s13046-019-1259-z
- Shimi T, Pfleghaar K, Kojima S, et al. The A- and B-type nuclear lamin networks: Microdomains involved in chromatin organization and transcription. Genes Dev. 2008;22(24):3409-3421. doi: 10.1101/gad.1735208
- Audia JE, Campbell RM. Histone modifications and cancer. Cold Spring Harb Perspect Biol. 2016;8(4):a019521. doi: 10.1101/cshperspect.a019521
- Kelleher FC, O’Sullivan H. FOXM1 in sarcoma: Role in cell cycle, pluripotency genes and stem cell pathways. Oncotarget. 2016;7(27):42792-42804. doi: 10.18632/oncotarget.8669
- Ishibashi Y, Miyoshi H, Hiraoka K, et al. Anaplastic lymphoma kinase protein expression, genetic abnormalities, and phosphorylation in soft tissue tumors: Phosphorylation is associated with recurrent metastasis. Oncol Rep. 2015;33(4):1667-1674. doi: 10.3892/or.2015.3806
- VanArsdale T, Boshoff C, Arndt KT, Abraham RT. Molecular pathways: Targeting the cyclin D-CDK4/6 axis for cancer treatment. Clin Cancer Res. 2015;21(13):2905-2910. doi: 10.1158/1078-0432.Ccr-14-0816