Hydrogen sulfide donors and inhibitors in cancer research: A state-of-the-art review
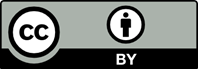
Hydrogen sulfide (H2S), a gaseous biomolecule, is considered a key player in the regulation of various essential cellular events. Normal physiology is determined by the level of endogenous H2S. Any alterations (upregulation and downregulation) to the level of endogenous H2S may lead to illness, including the onset of tumorigenesis. Over the past two decades, extensive research on the role of H2S in cancer development has affirmed the potential pharmacological means to suppress cancer progression by either inhibiting H2S synthesis in cells or exposing exogenously supplied H2S donors to treat different cancers. Some H2S donors and inhibitors release H2S or affect its synthesis. As a result, they have progressed through the development process into widespread clinical use and become increasingly important. The present study draws a detailed discussion on the types of H2S donors and inhibitors and their role in cancer research. We believe that this state-of-the-art review will empower the synthesis of H2S -based chemopreventive drugs and promote the need for further in-depth exploration of the associations between H2S and cancer treatments in clinical settings.
Mishanina TV, Libiad M, Banerjee R, 2015, Biogenesis of reactive sulfur species for signaling by hydrogen sulfide oxidation pathways. Nat Chem Biol, 11(7): 457–464. https://doi.org/10.1038/nchembio.1834
Cao X, Ding L, Xie ZZ, et al., 2019, A review of hydrogen sulfide synthesis, metabolism, and measurement: is modulation of hydrogen sulfide a novel therapeutic for cancer? Antioxid Redox Signal, 31(1): 1–38. https://doi.org/10.1089/ars.2017.7058
Augsburger F, Szabo C, 2020, Potential role of the 3-mercaptopyruvate sulfurtransferase (3-MST)—hydrogen sulfide (H2S) pathway in cancer cells. Pharmacol Res, 154: 104083. https://doi.org/10.1016/j.phrs.2018.11.034
Shi Y, Hussain Z, Zhao Y, 2022, Promising application of D-amino acids toward clinical therapy. Int J Mol Sci, 23(18): 10794. https://doi.org/10.3390/ijms231810794
Myszkowska J, Derevenkov I, Makarov SV, et al., 2021, Biosynthesis, quantification and genetic diseases of the smallest signaling thiol metabolite: Hydrogen sulfide. Antioxidants (Basel), 10(7): 1065. https://doi.org/10.3390/antiox10071065
Kohl JB, Mellis AT, Schwarz G, 2019, Homeostatic impact of sulfite and hydrogen sulfide on cysteine catabolism. Br J Pharmacol, 176(4): 554–570. https://doi.org/10.1111/bph.14464
Khattak S, Rauf MA, Khan NH, et al., 2022, Hydrogen sulfide biology and its role in cancer. Molecules, 27(11): 3389. https://doi.org/10.3390/molecules27113389
Sun HJ, Wu ZY, Nie XW, et al., 2021, Implications of hydrogen sulfide in liver pathophysiology: Mechanistic insights and therapeutic potential. J Adv Res, 27: 127–135. https://doi.org/10.1016/j.jare.2020.05.010
Kumar M, Sandhir R, 2018, Hydrogen sulfide in physiological and pathological mechanisms in brain. CNS Neurol Disord Drug Targets, 17(9): 654–670. https://doi.org/10.2174/1871527317666180605072018
Mir JM, Maurya RC, 2018, Physiological and pathophysiological implications of hydrogen sulfide: A persuasion to change the fate of the dangerous molecule. J Chin Adv Mater Soc, 6(4): 434–458. https://doi.org/10.1080/22243682.2018.1493951
Padilla-Camberos E, Zaitseva G, Padilla C, et al., 2010, Antitumoral activity of allicin in murine lymphoma L5178Y. Asian Pac J Cancer Prev, 11(5): 1241–1244.
Salehi B, Zucca P, Orhan IE, et al., 2019, Allicin and health: A comprehensive review. Trends Food Sci Technol, 86: 502–516. https://doi.org/10.1016/j.tifs.2019.03.003
Haghi A, Azimi H, Rahimi R, 2017, A comprehensive review on pharmacotherapeutics of three phytochemicals, curcumin, quercetin, and allicin, in the treatment of gastric cancer. J Gastroint Cancer, 48(4): 314–320. https://doi.org/10.1007/s12029-017-9997-7
Wang Z, Liu Z, Cao Z, et al., 2012, Allicin induces apoptosis in EL-4 cells in vitro by activation of expression of caspase-3 and-12 and up-regulation of the ratio of Bax/Bcl-2. Nat Prod Res, 26(11): 1033–1037. https://doi.org/10.1080/14786419.2010.550894
Xiang Y, Zhao J, Zhao M, et al., 2018, Allicin activates autophagic cell death to alleviate the malignant development of thyroid cancer. Exp Ther Med, 15(4): 3537–3543. https://doi.org/10.3892/etm.2018.5828
Zhang W, Ha M, Gong Y, et al., 2010, Allicin induces apoptosis in gastric cancer cells through activation of both extrinsic and intrinsic pathways. Oncol Rep, 24(6): 1585– 1592. https://doi.org/10.3892/or_00001021
McGrowder DA, Miller FG, Nwokocha CR, et al., 2020, Medicinal herbs used in traditional management of breast cancer: Mechanisms of action. Medicines (Basel), 7(8): 47. https://doi.org/10.3390/medicines7080047
Lv Q, Xia Q, Li J, et al., 2020, Allicin suppresses growth and metastasis of gastric carcinoma: The key role of microRNA- 383-5p-mediated inhibition of ERBB4 signaling. Biosci Biotechnol Biochem, 84(10): 1997–2004. https://doi.org/10.1080/09168451.2020.1780903
Schultz CR, Gruhlke MCH, Slusarenko AJ, et al., 2020, Allicin, a potent new ornithine decarboxylase inhibitor in neuroblastoma cells. J Nat Prod, 83(8): 2518–2527. https://doi.org/10.1021/acs.jnatprod.0c00613
Jiang W, Huang Y, Wang JP, et al., 2013, The synergistic anticancer effect of artesunate combined with allicin in osteosarcoma cell line in vitro and in vivo. Asian Pac J Cancer Prev, 14(8): 4615–4619. https://doi.org/10.7314/apjcp.2013.14.8.4615
Cai J, Wu X, Li X, et al., 2019, Allicin protects against cisplatin-induced stria vascularis damage: Possible relation to inhibition of Caspase-3 and PARP-1-AIF-mediated apoptotic pathways. ORL J Otorhinolaryngol Relat Spec, 81(4): 202–214.
Wu H, Li X, Zhang T, et al., 2020, Overexpression miR-486-3p promoted by allicin enhances temozolomide sensitivity in glioblastoma via targeting MGMT. Neuromolecular Med, 22(3): 359–369. https://doi.org/10.1007/s12017-020-08592-5
Zou X, Liang J, Sun J, et al., 2016, Allicin sensitizes hepatocellular cancer cells to anti-tumor activity of 5-fluorouracil through ROS-mediated mitochondrial pathway. J Pharmacol Sci, 131(4): 233–240. https://doi.org/10.1016/j.jphs.2016.04.017
Țigu AB, Toma VA, Moț AC, et al., 2020, The synergistic antitumor effect of 5-fluorouracil combined with allicin against lung and colorectal carcinoma cells. Molecules, 25(8): 1947. https://doi.org/10.3390/molecules25081947
Terrasson J, Xu B, Li M, et al., 2007, Activities of Z‐ajoene against tumour and viral spreading in vitro. Fundam Clin Pharmacol, 21(3): 281–289. https://doi.org/10.1111/j.1472-8206.2007.00470.x
He W, Fu Y, Zheng Y, et al., 2021, Diallyl thiosulfinate enhanced the anti-cancer activity of dexamethasone in the side population cells of multiple myeloma by promoting miR-127-3p and deactivating the PI3K/AKT signaling pathway. BMC Cancer, 21(1): 1–10.
Pandey N, Tyagi G, Kaur P, et al., 2020, Allicin overcomes hypoxia mediated cisplatin resistance in lung cancer cells through ROS mediated cell death pathway and by suppressing hypoxia inducible factors. Cell Physiol Biochem, 54(4): 748–766. https://doi.org/10.33594/000000253
Yang Z, Du J, Zhu J, et al., 2020, Allicin inhibits proliferation by decreasing IL-6 and IFN-β in HCMV-infected glioma cells. Cancer Manag Res, 12: 7305–7317. https://doi.org/10.2147/CMAR.S259677
Sultan SA, Khawaji MH, Alsughayyir J, et al., 2020, Antileukemic activity of sulfoxide nutraceutical allicin against THP-1 cells is associated with premature phosphatidylserine exposure in human erythrocytes. Saudi J Biol Sci, 27(12): 3376–3384. https://doi.org/10.1016/j.sjbs.2020.09.005
Ossama M, Hathout RM, Attia DA, et al., 2019, Enhanced allicin cytotoxicity on HEPG-2 cells using glycyrrhetinic acid surface-decorated gelatin nanoparticles. ACS Omega, 4(6): 11293–11300. https://doi.org/10.1021/acsomega.9b01580
Chen X, Li H, Xu W, et al., 2020, Self-assembling cyclodextrin-based nanoparticles enhance the cellular delivery of hydrophobic allicin. J Agric Food Chem, 68(40): 11144–11150. https://doi.org/10.1021/acs.jafc.0c01900
Klinck, J, 2022, Investigations into the Cytotoxic Mechanism of Action for the Garlic Compound Ajoene and its Derivatives in Breast Cancer Cells.
Kaschula CH, Hunter R, Cotton J, et al., 2016, The garlic compound ajoene targets protein folding in the endoplasmic reticulum of cancer cells. Mol Carcinog, 55(8): 1213–1228. https://doi.org/10.1002/mc.22364
Fang ZJ, Huang WX, Huang MH, et al., 2002, Gene expression profiling of human promyelocytic leukemia HL-60 cell treated by ajoene. Chin J Cancer Res, 14(1): 11–17.
Kash N, Silapunt S, 2021, A review of emerging and non-US FDA-approved topical agents for the treatment of basal cell carcinoma. Future Oncol, 17(23): 3111–3132. https://doi.org/10.2217/fon-2020-1147
Nagini S, 2008, Cancer chemoprevention by garlic and its organosulfur compounds-panacea or promise? Anticancer Agents Med Chem, 8(3): 313–321. https://doi.org/10.2174/187152008783961879
Jung Y, Park H, Zhao HY, et al., 2014, Systemic approaches identify a garlic-derived chemical, Z-ajoene, as a glioblastoma multiforme cancer stem cell-specific targeting agent. Mol Cells, 37(7): 547–553. https://doi.org/10.14348/molcells.2014.0158
Kaschula CH, Tuveri R, Ngarande E, et al., 2019, The garlic compound ajoene covalently binds vimentin, disrupts the vimentin network and exerts anti-metastatic activity in cancer cells. BMC Cancer, 19(1): 248. https://doi.org/10.1186/s12885-019-5388-8
Siyo V, Schäfer G, Hunter R, et al., 2017, The cytotoxicity of the ajoene analogue BisPMB in WHCO1 oesophageal cancer cells is mediated by CHOP/GADD153. Molecules, 22(6): 892. https://doi.org/10.3390/molecules22060892
Lee H, Heo JW, Kim AR, et al., 2019, Z-ajoene from crushed garlic alleviates cancer-induced skeletal muscle atrophy. Nutrients, 11(11): 2724. https://doi.org/10.3390/nu11112724
Huang YS, Xie N, Su Q, et al., 2011, Diallyl disulfide inhibits the proliferation of HT-29 human colon cancer cells by inducing differentially expressed genes. Mol Med Rep, 4(3): 553–559. https://doi.org/10.3892/mmr.2011.453
Shan Y, Wei Z, Tao L, et al., 2016, Prophylaxis of diallyl disulfide on skin carcinogenic model via p21-dependent Nrf2 stabilization. Sci Rep, 6(1): 35676. https://doi.org/10.1038/srep35676
Khan A, Shukla Y, Kalra N, et al. 2007, Potential of diallyl sulfide bearing pH-sensitive liposomes in chemoprevention against DMBA-induced skin papilloma. Mol Med. 13(7-8): 443–451. https://doi.org/10.2119/2006–00111.Khan
McCaskill ML, Rogan E, Thomas RD, 2014, Diallyl sulfide inhibits diethylstilbestrol induced DNA damage in human breast epithelial cells (MCF-10A). Steroids, 92: 96–100. https://doi.org/100.10.1016/j.steroids.2014.09.005
Prasad S, Kalra N, Shukla Y, 2006, Modulatory effects of diallyl sulfide against testosterone‐induced oxidative stress in Swiss albino mice. Asian J Androl, 8(6): p. 719-723. https://doi.org/10.1111/j.1745-7262.2006.00201.x
Hwang JS, et al., 2017, DATS sensitizes glioma cells to TRAIL-mediated apoptosis by up-regulation of death receptor 5 via ROS. Food Chem Toxicol, 106(Pt A): 514–521. https://doi.org/10.1016/j.fct.2017.05.056
Suman S, Shukla Y, 2016, Diallyl sulfide and its role in chronic diseases prevention. Adv Exp Med Biol, 929: 127–144. https://doi.org/10.1007/978-3-319-41342-6_6
Arora A, Seth K, Shukla Y, 2004, Reversal of P-glycoprotein-mediated multidrug resistance by diallyl sulfide in K562 leukemic cells and in mouse liver. Carcinogenesis, 25(6): 941–949. https://doi.org/10.1093/carcin/bgh060
Wu PP, Chung HW, Liu KC, et al., 2011, Diallyl sulfide induces cell cycle arrest and apoptosis in HeLa human cervical cancer cells through the p53, caspase-and mitochondria-dependent pathways. Int J Oncol, 38(6): 1605–1613. https://doi.org/10.3892/ijo.2011.973
Ansari IA, Ahmad A, Imran MA, et al., 2021, Organosulphur compounds induce apoptosis and cell cycle arrest in cervical cancer cells via downregulation of HPV E6 and E7 oncogenes. Anticancer Agents Med Chem, 21(3): 393–405. https://doi.org/10.2174/1871520620999200818154456
Aquilano K, Filomeni G, Baldelli S, et al., 2007, Neuronal nitric oxide synthase protects neuroblastoma cells from oxidative stress mediated by garlic derivatives. J Neurochem, 101(5): 1327–1337. https://doi.org/10.1111/j.1471-4159.2006.04431.x
Khan A, Alhumaydhi FA, Alwashmi ASS, et al., 2020, Diallyl sulfide-mediated modulation of the fatty acid synthase (FASN) leads to cancer cell death in BaP-induced lung carcinogenesis in Swiss mice. J Inflamm Res, 13: 1075–1087. https://doi.org/10.2147/JIR.S284279
Muninathan N, 2021, Amelioration of combination of paclitaxel and di allyl sulfide on the alterations of Bcl2, P53 and apoptosis changes against 7, 12 di methyl benz (A) anthracene induced skin cancer in experimental animals. Indian J Clin Biochem, 36(2): 143–150. https://doi.org/10.1007/s12291-019-0817-7
Wargovich MJ, Imada O, Stephens LC, 1992, Initiation and post-initiation chemopreventive effects of diallyl sulfide in esophageal carcinogenesis. Cancer Lett, 64(1): 39–42. https://doi.org/10.1016/0304-3835(92)90019-r
Yin X, Feng C, Han L, et al., 2018, Diallyl disulfide inhibits the metastasis of type II esophageal-gastric junction adenocarcinoma cells via NF-κB and PI3K/AKT signaling pathways in vitro. Oncol Rep, 39(2): 784–794. https://doi.org/10.3892/or.2017.6113
Yi L, Su Q, 2013, Molecular mechanisms for the anti-cancer effects of diallyl disulfide. Food Chem Toxicol, 57: 362–370. https://doi.org/10.1016/j.fct.2013.04.001
Druesne N, Pagniez A, Mayeur C, et al., 2004, Repetitive treatments of colon HT‐29 cells with diallyl disulfide induce a prolonged hyperacetylation of histone H3 K14. Ann N Y Acad Sci, 1030(1): 612–621. https://doi.org/10.1196/annals.1329.071
Yang JS, Chen GW, Hsia TC, et al., 2009, Diallyl disulfide induces apoptosis in human colon cancer cell line (COLO 205) through the induction of reactive oxygen species, endoplasmic reticulum stress, caspases casade and mitochondrial-dependent pathways. Food Chem Toxicol, 47(1): 171–179.
Su J, Zhou Y, Pan Z, et al., 2017, Downregulation of LIMK1– ADF/cofilin by DADS inhibits the migration and invasion of colon cancer. Scientific Reports, 7(1): 1–12.
Yi L, Ji XX, Lin M, et al., 2010, Diallyl disulfide induces apoptosis in human leukemia HL-60 cells through activation of JNK mediated by reactive oxygen. Pharmazie, 65(9): 693–698.
Ling H, He J, Tan H, et al., 2017, Identification of potential targets for differentiation in human leukemia cells induced by diallyl disulfide. Int J Oncol, 50(2): 697–707. https://doi.org/10.3892/ijo.2017.3839
Liu R, Yang YN, Yi L, et al., 2018, Diallyl disulfide effect on the invasion and migration ability of HL-60 cells with a high expression of DJ-1 in the nucleus through the suppression of the Src signaling pathway. Oncol Lett, 15(5): 6377–6385. https://doi.org/10.3892/ol.2018.8139
Arunkumar A, Vijayababu MR, Venkataraman P, et al., 2006, Chemoprevention of rat prostate carcinogenesis by diallyl disulfide, an organosulfur compound of garlic. Biol Pharm Bull, 29(2): 375–379. https://doi.org/10.1248/bpb.29.375
Arunkumar R, Sharmila G, Elumalai P, et al., 2012, Effect of diallyl disulfide on insulin-like growth factor signaling molecules involved in cell survival and proliferation of human prostate cancer cells in vitro and in silico approach through docking analysis. Phytomedicine, 19(10): 912–923. https://doi.org/10.1016/j.phymed.2012.04.009
Wang HC, Yang JH, Hsieh SC, et al., 2010, Allyl sulfides inhibit cell growth of skin cancer cells through induction of DNA damage mediated G2/M arrest and apoptosis. J Agric Food Chem, 58(11): 7096–7103. https://doi.org/10.1021/jf100613x
Lei XY, Yao SQ, Zu XY, et al., 2008, Apoptosis induced by diallyl disulfide in human breast cancer cell line MCF‐7 1. Acta Pharmacol Sin, 29(10): 1233–1239. https://doi.org/10.1111/j.1745-7254.2008.00851.x
Xiao X, Chen B, Liu X, et al., 2014, Diallyl disulfide suppresses SRC/Ras/ERK signaling-mediated proliferation and metastasis in human breast cancer by up-regulating miR-34a. PLoS One, 9(11): e112720. https://doi.org/10.1371/journal.pone.0112720
Xiong T, Liu XW, Huang XL, et al., 2018, Tristetraprolin: A novel target of diallyl disulfide that inhibits the progression of breast cancer. Oncol Lett, 15(5): 7817–7827. https://doi.org/10.3892/ol.2018.8299
Nkrumah-Elie YM, Reuben JS, Hudson AM, et al., 2012, The attenuation of early benzo (a) pyrene-induced carcinogenic insults by diallyl disulfide (DADS) in MCF-10A cells. Nutr Cancer, 64(7): 1112–1121. https://doi.org/10.1080/01635581.2012.712738
Altonsy MO, Habib TN, Andrews SC, Diallyl disulfide-induced apoptosis in a breast-cancer cell line (MCF-7) may be caused by inhibition of histone deacetylation. Nutr Cancer, 64(8): 1251–1260. https://doi.org/10.1080/01635581.2012.721156
Ciocci M, Iorio E, Carotenuto F, et al., 2016, H2S-releasing nanoemulsions: A new formulation to inhibit tumor cells proliferation and improve tissue repair. Oncotarget, 7(51): 84338–84358. https://doi.org/10.18632/oncotarget.12609
Siddhartha VT, Pindiprolu SKS, Chintamaneni PK, et al., 2018, RAGE receptor targeted bioconjuguate lipid nanoparticles of diallyl disulfide for improved apoptotic activity in triple negative breast cancer: In vitro studies. Artif Cells Nanomed Biotechnol, 46(2): 387–397. https://doi.org/10.1080/21691401.2017.1313267
Ji C, Ren F, Ma H, et al., 2010, The roles of p38MAPK and caspase-3 in DADS-induced apoptosis in human HepG2 cells. J Exp Clin Cancer Res, 29(1): 50. https://doi.org/10.1186/1756-9966-29-50
Hong YS, Ham YA, Choi JH, et al., 2000, Effects of allyl sulfur compounds and garlic extract on the expression of Bcl-2, Bax, and p53 in non small cell lung cancer cell lines. Exp Mol Med, 32(3): 127–134. https://doi.org/10.1038/emm.2000.22
Das B, Sinha D, 2019, Diallyl disulphide suppresses the cannonical Wnt signaling pathway and reverses the fibronectin-induced epithelial mesenchymal transition of A549 lung cancer cells. Food Funct, 10(1): 191–202. https://doi.org/10.1039/c8fo00246k
Thejass P, Kuttan G, 2007, Antiangiogenic activity of diallyl sulfide (DAS). Int Immunopharmacol, 7(3): 295–305. https://doi.org/10.1016/j.intimp.2006.10.011
Su B, Su J, Zeng Y, et al., 2018, Diallyl disulfide inhibits TGF-β1-induced upregulation of Rac1 and β-catenin in epithelial-mesenchymal transition and tumor growth of gastric cancer. Oncol Rep, 39(6): 2797–2806. https://doi.org/10.3892/or.2018.6345
Filomeni G, Aquilano K, Rotilio G, et al., 2005, Glutathione-related systems and modulation of extracellular signal– regulated kinases are involved in the resistance of AGS adenocarcinoma gastric cells to diallyl disulfide–induced apoptosis. Cancer Res, 65(24): 11735–11742. https://doi.org/10.1158/0008-5472.CAN-05-3067
Das A, Banik NL, Ray SK, 2007, Garlic compounds generate reactive oxygen species leading to activation of stress kinases and cysteine proteases for apoptosis in human glioblastoma T98G and U87MG cells. Cancer, 110(5): 1083–1095. https://doi.org/10.1002/cncr.22888
Di C, Sun C, Li H, et al., 2015, Diallyl disulfide enhances carbon ion beams–induced apoptotic cell death in cervical cancer cells through regulating Tap73/ΔNp73. Cell Cycle, 14(23): 3725–3733. https://doi.org/10.1080/15384101.2015.1104438
Lu HF, Sue CC, Yu CS, et al., 2004, Diallyl disulfide (DADS) induced apoptosis undergo caspase-3 activity in human bladder cancer T24 cells. Food Chem Toxicol, 42(10): 1543–1552. https://doi.org/10.1016/j.fct.2003.06.001
Yue Z, Guan X, Chao R, et al., 2019, Diallyl disulfide induces apoptosis and autophagy in human osteosarcoma MG-63 cells through the PI3K/Akt/mTOR pathway. Molecules, 24(14): 2665. https://doi.org/10.3390/molecules24142665
Borkowska A, Sielicka-Dudzin A, Herman-Antosiewicz A, et al., 2012, Diallyl trisulfide-induced prostate cancer cell death is associated with Akt/PKB dephosphorylation mediated by P-p66shc. Eur J Nutr, 51(7): 817–825. https://doi.org/10.1007/s00394-011-0260-x
Kim SH, Hahm ER, Singh KB, et al., 2020, Diallyl trisulfide inhibits leptin-induced oncogenic signaling in human breast cancer cells but fails to prevent chemically-induced luminal-type cancer in rats. J Cancer Prev, 25(1): 1–12. https://doi.org/10.15430/JCP.2020.25.1.1
Elsherbiny NM, El-Sherbiny M, Zaitone SA, 2020, Diallyl trisulfide potentiates chemotherapeutic efficacy of doxorubicin in experimentally induced mammary carcinoma: Role of Notch signaling. Pathol Res Pract, 216(10): 153139. https://doi.org/10.1016/j.prp.2020.153139
Shin DY, Kim GY, Hwang HJ, et al., 2014, Diallyl trisulfide-induced apoptosis of bladder cancer cells is caspase-dependent and regulated by PI3K/Akt and JNK pathways. Environ Toxicol Pharmacol, 37(1): 74–83. https://doi.org/10.1016/j.etap.2013.11.002
Geng H, Guo W, Feng L, et al., 2021, Diallyl trisulfide inhibited tobacco smoke-mediated bladder EMT and cancer stem cell marker expression via the NF-κB pathway in vivo. J Int Med Res, 49(3): 0300060521992900. https://doi.org/10.1177/0300060521992900
Wang J, Si L, Wang G, et al., 2019, Increased sulfiredoxin expression in gastric cancer cells may be a molecular target of the anticancer component diallyl trisulfide. Biomed Res Int, 2019: 4636804. https://doi.org/10.1155/2019/4636804
Jiang XY, Zhu XS, Xu HY, et al., 2017, Diallyl trisulfide suppresses tumor growth through the attenuation of Nrf2/ Akt and activation of p38/JNK and potentiates cisplatin efficacy in gastric cancer treatment. Acta Pharmacol Sin, 38(7): 1048–1058. https://doi.org/10.1038/aps.2016.176
Wang H, Sun N, Li X, et al., 2016, Diallyl trisulfide induces osteosarcoma cell apoptosis through reactive oxygen species-mediated downregulation of the PI3K/Akt pathway. Oncol Rep, 35(6): 3648–3658.https://doi.org/10.3892/or.2016.4722
Xie WP, Zhang Y, Zhang YK, et al., 2018, Treatment of Saos-2 osteosarcoma cells with diallyl trisulfide is associated with an increase in calreticulin expression. Exp Ther Med, 15(6): 4737–4742. https://doi.org/10.3892/etm.2018.6037
He P, Wang Z, Sheng B, et al., 2020, Diallyl trisulfide regulates cell apoptosis and invasion in human Osteosarcoma U2OS cells through regulating PI3K/AKT/GSK3β signaling pathway. Histol Histopathol, 35(12): 1511–1520. https://doi.org/10.14670/HH-18-299
Jiang X, Zhu X, Liu N, et al., 2017, Diallyl trisulfide inhibits growth of NCI-H460 in vitro and in vivo, and ameliorates cisplatin-induced oxidative injury in the treatment of lung carcinoma in xenograft mice. Int J Biol Sci, 13(2): 167–178. https://doi.org/10.7150/ijbs.16828
Liu Y, Fu R, Tu S, et al., 2021, Extracellular microparticles encapsulated with Diallyl Trisulfide interfere with the inflammatory tumor microenvironment and lung metastasis of invasive melanoma. Mol Pharmaceutics, 18(3): 822–835. https://doi.org/10.1021/acs.molpharmaceut.0c00696
Xu S, Pan J, Cheng X, et al., 2020, Diallyl trisulfide, a H2S donor, inhibits cell growth of human papillary thyroid carcinoma KTC‐1 cells through a positive feedback loop between H2S and cystathionine‐gamma‐lyase. Phytother Res, 34(5): 1154–1165. https://doi.org/10.1002/ptr.6586
Lai KC, Hsu SC, Yang JS, et al., 2015, Diallyl trisulfide inhibits migration, invasion and angiogenesis of human colon cancer HT‐29 cells and umbilical vein endothelial cells, and suppresses murine xenograft tumour growth. J Cell Mol Med, 19(2): 474–484. https://doi.org/10.1111/jcmm.12486
Nakagawa C, Suzuki-Karasaki M, Suzuki-Karasaki M, et al., 2020, The mitochondrial Ca2+ overload via voltage-gated Ca2+ entry contributes to an anti-melanoma effect of diallyl trisulfide. Int J Mol Sci, 21(2): 491. https://doi.org/10.3390/ijms21020491
Wan HF, Yu LH, Wu JL, et al., 2013, Effect of diallyl trisulfide on human ovarian cancer SKOV-3/DDP cell apoptosis. Asian Pac J Cancer Prev, 14(12): 7197–7201. https://doi.org/10.7314/apjcp.2013.14.12.7197
Agassi SFT, Yeh TM, Chang CD, et al., 2020, Potentiation of differentiation and apoptosis in a human promyelocytic leukemia cell line by garlic essential oil and its organosulfur compounds. Anticancer Res, 40(11): 6345–6354. https://doi.org/10.21873/anticanres.14655
Das A, Henderson F Jr, Lowe S, et al., 2018, Single agent efficacy of the HDAC inhibitor DATS in preclinical models of glioblastoma. Cancer Chemother Pharmacol, 82(6): 945–952. https://doi.org/10.1007/s00280-018-3684-7
Shigemi Z, Furukawa Y, Hosokawa K, et al., 2016, Diallyl trisulfide induces apoptosis by suppressing NF-κB signaling through destabilization of TRAF6 in primary effusion lymphoma. Int J Oncol, 48(1): 293–304. https://doi.org/10.3892/ijo.2015.3247
Abbaoui B, Riedl KM, Ralston RA, et al., 2012, Inhibition of bladder cancer by broccoli isothiocyanates sulforaphane and erucin: Characterization, metabolism, and interconversion. Mol Nutr Food Res, 56(11): 1675–1687. https://doi.org/10.1002/mnfr.201200276
Abbaoui B, Telu KH, Lucas CR, et al., 2017, The impact of cruciferous vegetable isothiocyanates on histone acetylation and histone phosphorylation in bladder cancer. J Proteomics, 156: 94–103. https://doi.org/10.1016/j.jprot.2017.01.013
Shan Y, Zhang L, Bao Y, et al., 2013, Epithelial-mesenchymal transition, a novel target of sulforaphane via COX-2/MMP2, 9/Snail, ZEB1 and miR-200c/ZEB1 pathways in human bladder cancer cells. J Nutr Biochem, 24(6): 1062–1069. https://doi.org/10.1016/j.jnutbio.2012.08.004
Wang F, Liu P, An H, et al., 2020, Sulforaphane suppresses the viability and metastasis, and promotes the apoptosis of bladder cancer cells by inhibiting the expression of FAT1. Int J Mol Med, 46(3): 1085–1095. https://doi.org/10.3892/ijmm.2020.4665
Justin S, Rutz J, Maxeiner S, et al., 2020, Bladder cancer metastasis induced by chronic Everolimus application can be counteracted by sulforaphane in vitro. Int J Mol Sci, 21(15): 5582. https://doi.org/10.3390/ijms21155582
Byun S, Shin SH, Park J, et al., 2016, Sulforaphene suppresses growth of colon cancer‐derived tumors via induction of glutathione depletion and microtubule depolymerization. Mol Nutr Food Res, 60(5): 1068–1078. https://doi.org/10.1002/mnfr.201501011
Zhou JW, Wang M, Sun NX, et al., 2019, Sulforaphane-induced epigenetic regulation of Nrf2 expression by DNA methyltransferase in human Caco-2 cells. Oncol Lett, 18(3): 2639–2647. https://doi.org/10.3892/ol.2019.10569
Yasuda S, Horinaka M, Sakai T, 2019, Sulforaphane enhances apoptosis induced by Lactobacillus pentosus strain S-PT84 via the TNFα pathway in human colon cancer cells. Oncol Lett, 18(4): 4253–4261. https://doi.org/10.3892/ol.2019.10739
Cheng AC, Shen CJ, Hung CM, et al., 2019, Sulforaphane decrease of SERTAD1 expression triggers G1/S arrest in breast cancer cells. J Med Food, 22(5): 444–450. https://doi.org/10.1089/jmf.2018.4195
Castro NP, Rangel MC, Merchant AS, et al., 2019, Sulforaphane suppresses the growth of triple-negative breast cancer stem-like cells in vitro and in vivo. Cancer Prev Res (Phila), 12(3): 147–158. https://doi.org/10.1158/1940-6207.CAPR-18-0241
Choi YH, 2018, ROS-mediated activation of AMPK plays a critical role in sulforaphane-induced apoptosis and mitotic arrest in AGS human gastric cancer cells. Gen Physiol Biophys, 37(2): 129–140. https://doi.org/10.4149/gpb_2017026
Wang Y, Wu H, Dong N, et al., 2021, Sulforaphane induces S-phase arrest and apoptosis via p53-dependent manner in gastric cancer cells. Sci Rep, 11(1): 2504. https://doi.org/10.1038/s41598-021-81815-2
Han S, Wang Z, Liu J, et al., 2021, miR-29a-3p-dependent COL3A1 and COL5A1 expression reduction assists sulforaphane to inhibit gastric cancer progression. Biochem Pharmacol, 188: 114539. https://doi.org/10.1016/j.bcp.2021.114539
Zhang C, Su ZY, Khor TO, et al., 2013, Sulforaphane enhances Nrf2 expression in prostate cancer TRAMP C1 cells through epigenetic regulation. Biochem Pharmacol, 85(9): 1398–1404. https://doi.org/10.1016/j.bcp.2013.02.010
Vyas AR, Moura MB, Hahm ER, et al., 2016, Sulforaphane inhibits c‐myc‐mediated prostate cancer stem‐like traits. J Cell Biochem, 117(11): 2482–2495. https://doi.org/10.1002/jcb.25541
Singh KB, Kim SH, Hahm ER, et al., 2018, Prostate cancer chemoprevention by sulforaphane in a preclinical mouse model is associated with inhibition of fatty acid metabolism. Carcinogenesis, 39(6): 826–837. https://doi.org/10.1093/carcin/bgy051
Rutz J, Thaler S, Maxeiner S, et al., 2020, Sulforaphane reduces prostate cancer cell growth and proliferation in vitro by modulating the cdk-cyclin axis and expression of the CD44 Variants 4, 5, and 7. Int J Mol Sci, 21(22): 8724. https://doi.org/10.3390/ijms21228724
Bryant CS, Kumar S, Chamala S, et al., 2010, Sulforaphane induces cell cycle arrest by protecting RB-E2F-1 complex in epithelial ovarian cancer cells. Molecular Cancer, 9(1): 1–9.
Chang CC, Hung CM, Yang YR, et al., 2013, Sulforaphane induced cell cycle arrest in the G2/M phase via the blockade of cyclin B1/CDC2 in human ovarian cancer cells. J Ovarian Res, 6(1): 41. https://doi.org/10.1186/1757-2215-6-41
Gong TT, Liu XD, Zhan ZP, et al., 2020, Sulforaphane enhances the cisplatin sensitivity through regulating DNA repair and accumulation of intracellular cisplatin in ovarian cancer cells. Exp Cell Res, 393(2): 112061. https://doi.org/10.1016/j.yexcr.2020.112061
Hsu YC, Chang SJ, Wang MY, et al., 2013, Growth inhibition and apoptosis of neuroblastoma cells through ROS-independent MEK/ERK activation by sulforaphane. Cell Biochem Biophys, 66(3): 765–774. https://doi.org/10.1007/s12013-013-9522-y
Yeh CT, Yen GC, 2005, Effect of sulforaphane on metallothionein expression and induction of apoptosis in human hepatoma HepG2 cells. Carcinogenesis, 26(12): 2138–2148. https://doi.org/10.1093/carcin/bgi185
Saha K, Fisher ML, Adhikary G, et al., 2017, Sulforaphane suppresses PRMT5/MEP50 function in epidermal squamous cell carcinoma leading to reduced tumor formation. Carcinogenesis, 38(8): 827–836. https://doi.org/10.1093/carcin/bgx044
Żuryń A, Krajewski A, Klimaszewska-Wiśniewska A, et al., 2019, Expression of cyclin B1, D1 and K in non-small cell lung cancer H1299 cells following treatment with sulforaphane. Oncol Rep, 41(2): 1313–1323. https://doi.org/10.3892/or.2018.6919
Xie C, Zhu J, Jiang Y, et al., 2019, Sulforaphane inhibits the acquisition of tobacco smoke-induced lung cancer stem cell-like properties via the IL-6/ΔNp63α/Notch Axis. Theranostics, 9(16): 4827–4840. https://doi.org/10.7150/thno.33812
Chen L, Chan LS, Lung HL, et al., 2019, Crucifera sulforaphane (SFN) inhibits the growth of nasopharyngeal carcinoma through DNA methyltransferase 1 (DNMT1)/ Wnt inhibitory factor 1 (WIF1) axis. Phytomedicine, 63: 153058. https://doi.org/10.1016/j.phymed.2019.153058
Chu WF, Wu DM, Liu W, et al., 2009, Sulforaphane induces G2–M arrest and apoptosis in high metastasis cell line of salivary gland adenoid cystic carcinoma. Oral Oncol, 45(11): 998–1004. https://doi.org/10.1016/j.oraloncology.2009.05.641
Gründemann C, Garcia-Käufer M, Lamy E, et al., 2015, 4-Methylthiobutyl isothiocyanate (Erucin) from rocket plant dichotomously affects the activity of human immunocompetent cells. Phytomedicine, 22(3): 369–378.
Melchini A, Traka MH, Catania S, et al., 2013, Antiproliferative activity of the dietary isothiocyanate erucin, a bioactive compound from cruciferous vegetables, on human prostate cancer cells. Nutr Cancer, 65(1): 132–138. https://doi.org/10.1080/01635581.2013.741747
Citi V, Piragine E, Pagnotta E, et al., 2019 Anticancer properties of erucin, an H2S‐releasing isothiocyanate, on human pancreatic adenocarcinoma cells (AsPC‐1). Phytother Res, 33(3): 845–855. https://doi.org/10.1002/ptr.6278
Lamy E, Oey D, Eißmann F, et al., 2013, Erucin and benzyl isothiocyanate suppress growth of late stage primary human ovarian carcinoma cells and telomerase activity in vitro. Phytother Res, 27(7): 1036–1041. https://doi.org/10.1002/ptr.4798
Li G, Zhou J, Budhraja A, et al., 2015, Mitochondrial translocation and interaction of cofilin and Drp1 are required for erucin-induced mitochondrial fission and apoptosis. Oncotarget, 6(3): 1834. https://doi.org/10.18632/oncotarget.2795
Tsai SC, Huang WW, Huang WC, et al., 2012, ERK-modulated intrinsic signaling and G2/M phase arrest contribute to the induction of apoptotic death by allyl isothiocyanate in MDA-MB-468 human breast adenocarcinoma cells. Int J Oncol, 41(6): 2065–2072. https://doi.org/10.3892/ijo.2012.1640
Sayeed MA, Bracci M, Ciarapica V, et al., 2018, Allyl isothiocyanate exhibits no anticancer activity in MDA-MB-231 breast cancer cells. Int J Mol Sci, 19(1): 145. https://doi.org/10.3390/ijms19010145
Qin G, Li P, Xue Z, 2018, Effect of allyl isothiocyanate on the viability and apoptosis of the human cervical cancer HeLa cell line in vitro. Oncol Lett, 15(6): 8756–8760. https://doi.org/10.3892/ol.2018.8428
Chang PY, Tsai FJ, Bau DT, et al., 2021, Potential effects of allyl isothiocyanate on inhibiting cellular proliferation and inducing apoptotic pathway in human cisplatin-resistant oral cancer cells. J Formos Med Assoc, 120(1): 515–523. https://doi.org/10.1016/j.jfma.2020.06.025
Hwang ES, Kim GH, 2009, Allyl isothiocyanate influences cell adhesion, migration and metalloproteinase gene expression in SK-Hep1 cells. Exp Biol Med (Maywood), 234(1): 105–111. https://doi.org/10.3181/0806-RM-190
Lai KC, Lu CC, Tang YJ, et al., 2014, Allyl isothiocyanate inhibits cell metastasis through suppression of the MAPK pathways in epidermal growth factor-stimulated HT29 human colorectal adenocarcinoma cells. Oncol Rep, 31(1): 189–196. https://doi.org/10.3892/or.2013.2865
Chiang JH, Tsai FJ, Hsu YM, et al., 2020, Sensitivity of allyl isothiocyanate to induce apoptosis via ER stress and the mitochondrial pathway upon ROS production in colorectal adenocarcinoma cells. Oncol Rep, 44(4): 1415–1424. https://doi.org/10.3892/or.2020.7700
Tsai TF, Chen PC, Lin YC, et al., 2020, Benzyl isothiocyanate promotes miR‐99a expression through ERK/AP‐1‐dependent pathway in bladder cancer cells. Environ Toxicol, 35(1): 47–54. https://doi.org/10.1002/tox.22841
Lin JF, Tsai TF, Yang SC, et al., 2017, Benzyl isothiocyanate induces reactive oxygen species-initiated autophagy and apoptosis in human prostate cancer cells. Oncotarget, 8(12): 20220–20234. https://doi.org/10.18632/oncotarget.15643
Kim SH, Singh SV, 2019, Role of krüppel-like factor 4-p21CIP1 axis in breast cancer stem-like cell inhibition by benzyl isothiocyanaterole of KLF4 in bCSC suppression by BITC. Cancer Prev Res (Phila), 12(3): 125–134. https://doi.org/10.1158/1940-6207.CAPR-18-0393
Kim SH, Singh SV, 2010, p53-independent apoptosis by benzyl isothiocyanate in human breast cancer cells is mediated by suppression of XIAP expressionxiap suppression in BITC-induced apoptosis. Cancer Prev Res(Phila), 3(6): 718–726. https://doi.org/10.1158/1940-6207.CAPR-10-0048
Huang YP, Jiang YW, Chen HY, et al., 2018, Benzyl isothiocyanate induces apoptotic cell death through mitochondria-dependent pathway in gefitinib-resistant NCI-H460 human lung cancer cells in vitro. Anticancer Res, 38(9): 5165–5176. https://doi.org/10.21873/anticanres.12839
Ma L, Chen Y, Han R, et al., 2019, Benzyl isothiocyanate inhibits invasion and induces apoptosis via reducing S100A4 expression and increases PUMA expression in oral squamous cell carcinoma cells. Braz J Med Biol Res, 52(4): e8409. https://doi.org/10.1590/1414-431X20198409
Wolf MA, Claudio PP, 2014, Benzyl isothiocyanate inhibits HNSCC cell migration and invasion, and sensitizes HNSCC cells to cisplatin. Nutr Cancer, 66(2): 285–294. https://doi.org/10.1080/01635581.2014.868912
Yue T, Zuo S, Bu D, et al., 2020, Aminooxyacetic acid (AOAA) sensitizes colon cancer cells to oxaliplatin via exaggerating apoptosis induced by ROS. J Cancer, 11(7): 1828–1838. https://doi.org/10.7150/jca.35375
Zhu M, Li W, Dong X, et al., 2017, Benzyl-isothiocyanate induces apoptosis and inhibits migration and invasion of hepatocellular carcinoma cells in vitro. J Cancer, 8(2): 240–248. https://doi.org/10.7150/jca.16402
Ohara M, Kimura S, Tanaka A, et al., 2011, Benzyl isothiocyanate sensitizes human pancreatic cancer cells to radiation by inducing apoptosis. Int J Mol Med, 28(6): 1043–1047. https://doi.org/10.3892/ijmm.2011.770
Kasiappan R, Jutooru I, Karki K, et al., 2016, Benzyl isothiocyanate (BITC) induces reactive oxygen species-dependent repression of STAT3 protein by down-regulation of specificity proteins in pancreatic cancer. J Biol Chem, 291(53): 27122–27133. https://doi.org/10.1074/jbc.M116.746339
Han KWW, Po WW, Sohn UD, et al., 2019, Benzyl isothiocyanate induces apoptosis via reactive oxygen species-initiated mitochondrial dysfunction and DR4 and DR5 death receptor activation in gastric adenocarcinoma cells. Biomolecules, 9(12): 839. https://doi.org/10.3390/biom9120839
Chen PY, Lin KC, Lin JP, et al., 2012, Phenethyl isothiocyanate (PEITC) inhibits the growth of human oral squamous carcinoma HSC-3 cells through G0/G1 phase arrest and mitochondria-mediated apoptotic cell death. Evid Based Complement Alternat Med, 2012: 718320. https://doi.org/10.1155/2012/718320
Lam-Ubol A, Fitzgerald AL, Ritdej A, et al., 2018, Sensory acceptable equivalent doses of β-phenylethyl isothiocyanate (PEITC) induce cell cycle arrest and retard the growth of p53 mutated oral cancer in vitro and in vivo. Food Funct, 9(7): 3640–3656. https://doi.org/10.1039/c8fo00865e
Chou YC, Chang MY, Lee HT, et al., 2018, Phenethyl isothiocyanate inhibits in vivo growth of xenograft tumors of human glioblastoma cells. Molecules, 23(9): 2305. https://doi.org/10.3390/molecules23092305
Wang D, Upadhyaya B, Liu Y, et al., 2014, Phenethyl isothiocyanate upregulates death receptors 4 and 5 and inhibits proliferation in human cancer stem-like cells. BMC Cancer, 14(1): 591. https://doi.org/10.1186/1471-2407-14-591
Yang MD, Lai KC, Lai TY, et al., 2010, Phenethyl isothiocyanate inhibits migration and invasion of human gastric cancer AGS cells through suppressing MAPK and NF-κB signal pathways. Anticancer Res, 30(6): 2135–2143.
Liu Y, Dey M, 2017, Dietary phenethyl isothiocyanate protects mice from colitis associated colon cancer. Int J Mol Sci, 18(9): 1908. https://doi.org/10.3390/ijms18091908
Shao WY, Yang YL, Yan H, et al., 2017, Phenethyl isothiocyanate suppresses the metastasis of ovarian cancer associated with the inhibition of CRM1-mediated nuclear export and mTOR-STAT3 pathway. Cancer Biol Ther, 18(1): 26–35. https://doi.org/10.1080/15384047.2016.1264540
Hsia TC, Huang YP, Jiang YW, et al., 2018, Phenethyl isothiocyanate induces apoptotic cell death through the mitochondria-dependent pathway in gefitinib-resistant NCI-H460 human lung cancer cells in vitro. Anticancer Res, 38(4): 2137–2147. https://doi.org/10.21873/anticanres.12454
Huang SH, Hsu MH, Hsu SC, et al., 2014, Phenethyl isothiocyanate triggers apoptosis in human malignant melanoma A375. S2 cells through reactive oxygen species and the mitochondria-dependent pathways. Hum Exp Toxicol, 33(3): 270–283. https://doi.org/10.1177/0960327113491508
Chen Y, Li Y, Wang XQ, et al., 2018, Phenethyl isothiocyanate inhibits colorectal cancer stem cells by suppressing Wnt/β‐catenin pathway. Phytother Res, 32(12): 2447–2455. https://doi.org/10.1002/ptr.6183
Gupta P, Srivastava SK, 2012, Antitumor activity of phenethyl isothiocyanate in HER2-positive breast cancer models. BMC Med, 10(1): 80. https://doi.org/10.1186/1741-7015-10-80
Ezeriņa D, Takano Y, Hanaoka K, et al., 2018, N-acetyl cysteine functions as a fast-acting antioxidant by triggering intracellular H2S and sulfane sulfur production. Cell Chem Biol, 25(4): 447–459.e4. https://doi.org/10.1016/j.chembiol.2018.01.011
Geng YD, Zhang L, Wang GY, et al., 2020, Xanthatin mediates G2/M cell cycle arrest, autophagy and apoptosis via ROS/XIAP signaling in human colon cancer cells. Nat Prod Res, 34(18): 2616–2620. https://doi.org/10.1080/14786419.2018.1544976
Zuhra K, Tomé CS, Masi L, et al., 2019, N-acetylcysteine serves as substrate of 3-mercaptopyruvate sulfurtransferase and stimulates sulfide metabolism in colon cancer cells. Cells, 8(8): 828. https://doi.org/10.3390/cells8080828
Jurkowska H, Wróbel M, 2018, Inhibition of human neuroblastoma cell proliferation by N-acetyl-L-cysteine as a result of increased sulfane sulfur level. Anticancer Res, 38(9): 5109–5113. https://doi.org/10.21873/anticanres.12831
Li J, Tu HJ, Li J, et al., 2007, N-acetyl cysteine inhibits human signet ring cell gastric cancer cell line (SJ-89) cell growth by inducing apoptosis and DNA synthesis arrest. Eur J Gastroenterol Hepatol, 19(9): 769–774. https://doi.org/10.1097/MEG.0b013e3282202bda
Qin W, Li C, Zheng W, et al., 2015, Inhibition of autophagy promotes metastasis and glycolysis by inducing ROS in gastric cancer cells. Oncotarget, 6(37): 39839–39854. https://doi.org/10.18632/oncotarget.5674
Duan C, Zhang B, Deng C, et al., 2016, Piperlongumine induces gastric cancer cell apoptosis and G2/M cell cycle arrest both in vitro and in vivo. Tumour Biol, 37(8): 10793–10804. https://doi.org/10.1007/s13277-016-4792-9
Bondad N, Boostani R, Barri A, et al., 2020, Protective effect of N-acetylcysteine on oxaliplatin-induced neurotoxicity in patients with colorectal and gastric cancers: A randomized, double blind, placebo-controlled, clinical trial. J Oncol Pharm Pract, 26(7): 1575–1582. https://doi.org/10.1177/1078155219900788
Sayin VI, Ibrahim MX, Larsson E, et al., 2014, Antioxidants accelerate lung cancer progression in mice. Sci Transl Med, 6(221): 221ra15. https://doi.org/10.1126/scitranslmed.3007653
Li J, Wang XH, Hu J, et al., 2020, Combined treatment with N‐acetylcysteine and gefitinib overcomes drug resistance to gefitinib in NSCLC cell line. Cancer Med, 9(4): 1495–1502. https://doi.org/10.1002/cam4.2610
Gersey ZC, Rodriguez GA, Barbarite E, et al., 2017, Curcumin decreases malignant characteristics of glioblastoma stem cells via induction of reactive oxygen species. BMC Cancer, 17(1): 99. https://doi.org/10.1186/s12885-017-3058-2
Agarwal A, Muñoz-Nájar U, Klueh U, et al., 2004, N-acetyl-cysteine promotes angiostatin production and vascular collapse in an orthotopic model of breast cancer. Am J Pathol, 164(5): 1683–1696. https://doi.org/10.1016/S0002-9440(10)63727-3
Monti D, Sotgia F, Whitaker-Menezes D, et al. 2017, Pilot study demonstrating metabolic and anti-proliferative effects of in vivo anti-oxidant supplementation with N-Acetylcysteine in Breast Cancer. Semin Oncol. 44(3):226-232. https://doi.org/10.1053/j.seminoncol.2017.10.001
Panjehpour M, Alaie S, 2010, N-acetylcysteine inhibits cadmium-induced cytotoxicity in human breast cancer cell line (MDA-MB468). Toxicol Lett, 196: S308. https://doi.org/10.1016/j.toxlet.2010.03.972
Huang Z, Hu H, 2021, Arginine deiminase induces immunogenic cell death and is enhanced by n-acetylcysteine in murine MC38 colorectal cancer cells and MDA-MB-231 human breast cancer cells in vitro. Molecules, 26(2): 511. https://doi.org/10.3390/molecules26020511
Supabphol A, Muangman V, Chavasiri W, et al., 2009, N-acetylcysteine inhibits proliferation, adhesion, migration and invasion of human bladder cancer cells. J Med Assoc Thai, 92(9): 1171–1177.
Tang L, Li G, Song L, et al., 2006, The principal urinary metabolites of dietary isothiocyanates, N-acetylcysteine conjugates, elicit the same anti-proliferative response as their parent compounds in human bladder cancer cells. Anticancer Drugs, 17(3): 297–305. https://doi.org/10.1097/00001813-200603000-00008
Miyajima A, Nakashima J, Tachibana M, et al., 1999, N‐Acetylcysteine modifies cis‐dichlorodiammineplatinum‐induced effects in bladder cancer cells. Jpn J Cancer Res, 90(5): 565–570.https://doi.org/10.1111/j.1349-7006.1999.tb00784.x
Supabphol MA, 2012, Antimetastatic potential of N-acetylcysteine on human prostate cancer cells. J Med Assoc Thai, 95(12): S56–S62.
Tozawa K, Okamoto T, Hayashi Y, et al., 2002, N-acetyl- L-cysteine enhances chemotherapeutic effect on prostate cancer cells. Urol Res, 30(1): 53–58. https://doi.org/10.1007/s00240-001-0226-1
Brum G, Carbone T, Still E, et al., 2013, N-acetylcysteine potentiates doxorubicin-induced ATM and p53 activation in ovarian cancer cells. Int J Oncol, 42(1): 211–218. https://doi.org/10.3892/ijo.2012.1680
Mantawy EM, Said RS, Kassem DH, et al., 2020, Novel molecular mechanisms underlying the ameliorative effect of N-acetyl-L-cysteine against ϒ-radiation-induced premature ovarian failure in rats. Ecotoxicol Environ Saf, 206: 111190. https://doi.org/10.1016/j.ecoenv.2020.111190
Mezencev R, Wang L, Xu W, et al., 2013, Molecular analysis of the inhibitory effect of N-acetyl-L-cysteine on the proliferation and invasiveness of pancreatic cancer cells. Anticancer Drugs, 24(5): 504–518. https://doi.org/10.1097/CAD.0b013e32836009d7
Pillai K, Mekkawy AH, Akhter J, et al., 2020, Enhancing the potency of chemotherapeutic agents by combination with bromelain and N-acetylcysteine-an in vitro study with pancreatic and hepatic cancer cells. Am J Transl Res, 12(11): 7404–7419.
Tsuyuki S, Yamauchi A, Nakamura H, et al., 1998, Possible availability of N-acetylcysteine as an adjunct to cytokine therapy for hepatocellular carcinoma. Clin Immunol Immunopathol, 88(2): 192–198. https://doi.org/10.1006/clin.1998.4574
Dagnino S, Bodinier B, Grigoryan H, et al., 2020, Agnostic cys34‐albumin adductomics and DNA methylation: Implication of N‐acetylcysteine in lung carcinogenesis years before diagnosis. Int J Cancer, 146(12): 3294–3303. https://doi.org/10.1002/ijc.32680
Breau M, Houssaini A, Lipskaia L, et al., 2019, The antioxidant N-acetylcysteine protects from lung emphysema but induces lung adenocarcinoma in mice. JCI Insight, 4(19): e127647. https://doi.org/10.1172/jci.insight.127647
De Preter G, Deriemaeker C, Danhier P, et al., 2016, A fast hydrogen sulfide–releasing donor increases the tumor response to radiotherapyhydrogen sulfide donor potentiates radiotherapy. Mol Cancer Ther, 15(1): 154–161. https://doi.org/10.1158/1535-7163.MCT-15-0691-T
Zhen Y, Zhang W, Liu C, et al., 2015, Exogenous hydrogen sulfide promotes C6 glioma cell growth through activation of the p38 MAPK/ERK1/2-COX-2 pathways. Oncol Rep, 34(5): 2413–2422. https://doi.org/10.3892/or.2015.4248
Zhao L, Wang Y, Yan Q, et al., 2015, Exogenous hydrogen sulfide exhibits anti-cancer effects though p38 MAPK signaling pathway in C6 glioma cells. Biol Chem, 396(11): 1247–1253. https://doi.org/10.1515/hsz-2015-0148
Cai WJ, Wang MJ, Ju LH, et al., 2010, Hydrogen sulfide induces human colon cancer cell proliferation: Role of Akt, ERK and p21. Cell Biol Int, 34(6): 565–572. https://doi.org/10.1042/CBI20090368
Faris P, Ferulli F, Vismara M, et al., 2020, Hydrogen sulfide-evoked intracellular Ca2+ signals in primary cultures of metastatic colorectal cancer cells. Cancers (Basel), 12(11): 3338. https://doi.org/10.3390/cancers12113338
Ma Z, Bi Q, Wang Y, 2015, Hydrogen sulfide accelerates cell cycle progression in oral squamous cell carcinoma cell lines. Oral Dis, 21(2): 156–162. https://doi.org/10.1111/odi.12223
Liu H, Chang J, Zhao Z, et al., 2017, Effects of exogenous hydrogen sulfide on the proliferation and invasion of human Bladder cancer cells. J Cancer Res Ther, 13(5): 829–832. https://doi.org/10.4103/jcrt.JCRT_423_17
Zhen Y, Wu Q, Ding Y, et al., 2018, Exogenous hydrogen sulfide promotes hepatocellular carcinoma cell growth by activating the STAT3-COX-2 signaling pathway. Oncol Lett, 15(5): 6562–6570. https://doi.org/10.3892/ol.2018.8154
Ye M, Yu M, Yang D, et al., 2020, Exogenous hydrogen sulfide donor NaHS alleviates nickel-induced epithelial-mesenchymal transition and the migration of A549 cells by regulating TGF-β1/Smad2/Smad3 signaling. Ecotoxicol Environ Saf, 195: 110464. https://doi.org/10.1016/j.ecoenv.2020.110464
Nagpure BV, Bian JS, 2014, Hydrogen sulfide inhibits A2A adenosine receptor agonist induced β-amyloid production in SH-SY5Y neuroblastoma cells via a cAMP dependent pathway. PLoS One, 9(2): e88508. https://doi.org/10.1371/journal.pone.0088508
Qiao P, Zhao F, Liu M, et al., 2017, Hydrogen sulfide inhibits mitochondrial fission in neuroblastoma N2a cells through the Drp1/ERK1/2 signaling pathway. Mol Med Rep, 16(1): 971–977. https://doi.org/10.3892/mmr.2017.6627
Hassan AY, Maulood IM, Salihi A, 2021, The vasodilatory mechanism of nitric oxide and hydrogen sulfide in the human mesenteric artery in patients with colorectal cancer. Exp Ther Med, 21(3): 214. https://doi.org/10.3892/etm.2021.9646
Xiao AY, Maynard MR, Piett CG, et al., 2019, Sodium sulfide selectively induces oxidative stress, DNA damage, and mitochondrial dysfunction and radiosensitizes glioblastoma (GBM) cells. Redox Biol, 26: 101220. https://doi.org/10.1016/j.redox.2019.101220
Bhattacharyya S, Saha S, Giri K, et al., 2013, Cystathionine beta-synthase (CBS) contributes to advanced ovarian cancer progression and drug resistance. PloS One, 8(11): e79167. https://doi.org/10.1371/journal.pone.0079167
Forti KM, Figueroa M, Torres B, et al., 2016, Calcium sulfide (CaS) nanostructure treatment on non‐small cell lung cancer. FASEB J, 30: 1099.4–1099.4.
Li N, Sun Q, Yu Z, et al., 2018, Nuclear-targeted photothermal therapy prevents cancer recurrence with near-infrared triggered copper sulfide nanoparticles. ACS Nano, 12(6): 5197–5206. https://doi.org/10.1021/acsnano.7b06870
Li Y, Lu W, Huang Q, et al., 2010, Copper sulfide nanoparticles for photothermal ablation of tumor cells. Nanomedicine (Lond), 5(8): 1161–1171. https://doi.org/10.2217/nnm.10.85
Lee ZW, Teo XY, Song ZJ, et al., 2017, Intracellular hyper-acidification potentiated by hydrogen sulfide mediates invasive and therapy resistant cancer cell death. Front Pharmacol, 8: 763. https://doi.org/10.3389/fphar.2017.00763
Sakuma S, Minamino S, Takase M, et al., 2019, Hydrogen sulfide donor GYY4137 suppresses proliferation of human colorectal cancer Caco-2 cells by inducing both cell cycle arrest and cell death. Heliyon, 5(8): e02244. https://doi.org/10.1016/j.heliyon.2019.e02244
Szadvari I, Hudecova S, Chovancova B, et al., 2019, Sodium/ calcium exchanger is involved in apoptosis induced by H2S in tumor cells through decreased levels of intracellular pH. Nitric Oxide, 87: 1–9. https://doi.org/10.1016/j.niox.2019.02.011
Lu S, Gao Y, Huang X, et al., 2014, GYY4137, a hydrogen sulfide (H2S) donor, shows potent anti-hepatocellular carcinoma activity through blocking the STAT3 pathway. Int J Oncol, 44(4): 1259–1267. https://doi.org/10.3892/ijo.2014.2305
Yurinskaya MM, Garbuz DG, Afanasiev VN, et al., 2020, Effects of the hydrogen sulfide donor GYY4137 and HSP70 protein on the activation of SH-SY5Y cells by lipopolysaccharide. Mol Biol (Mosk), 54(6): 1018–1028. https://doi.org/10.31857/S0026898420060142
Hasegawa U, Tateishi N, Uyama H, et al., 2015, Hydrolysis‐sensitive dithiolethione prodrug micelles. Macromol Biosci, 15(11): 1512–1522. https://doi.org/10.1002/mabi.201500156
Cai F, Xu H, Cao N, et al., 2020, ADT-OH, a hydrogen sulfide-releasing donor, induces apoptosis and inhibits the development of melanoma in vivo by upregulating FADD. Cell Death Dis, 11(1): 33. https://doi.org/10.1038/s41419-020-2222-9
Dong Q, Yang B, Han JG, et al., 2019, A novel hydrogen sulfide-releasing donor, HA-ADT, suppresses the growth of human breast cancer cells through inhibiting the PI3K/ AKT/mTOR and Ras/Raf/MEK/ERK signaling pathways. Cancer Lett, 455: 60–72. https://doi.org/10.1016/j.canlet.2019.04.031
Ma K, Liu Y, Zhu Q, et al., 2011, H2S donor, S-propargyl-cysteine, increases CSE in SGC-7901 and cancer-induced mice: Evidence for a novel anti-cancer effect of endogenous H2S? PLoS One, 6(6): e20525. https://doi.org/10.1371/journal.pone.0020525
Wang W, Cheng J, Zhu Y, 2015, The JNK signaling pathway is a novel molecular target for S-propargyl-L-cysteine, a naturally-occurring garlic derivatives: Link to its anticancer activity in pancreatic cancer in vitro and in vivo. Curr Cancer Drug Targets, 15(7): 613–623. https://doi.org/10.2174/1568009615666150602143943
Covarrubias AE, Lecarpentier E, Lo A, et al., 2019, AP39, a modulator of mitochondrial bioenergetics, reduces antiangiogenic response and oxidative stress in hypoxia-exposed trophoblasts: Relevance for preeclampsia pathogenesis. Am J Pathol, 189(1): 104–114. https://doi.org/10.1016/j.ajpath.2018.09.007
Szczesny B, Módis K, Yanagi K, et al., 2014, AP39, a novel mitochondria-targeted hydrogen sulfide donor, stimulates cellular bioenergetics, exerts cytoprotective effects and protects against the loss of mitochondrial DNA integrity in oxidatively stressed endothelial cells in vitro. Nitric Oxide, 41: 120–130. https://doi.org/10.1016/j.niox.2014.04.008
Xu S, Yang CT, Meng FH, et al., 2016, Ammonium tetrathiomolybdate as a water-soluble and slow-release hydrogen sulfide donor. Bioorg Med Chem Lett, 26(6): 1585–1588. https://doi.org/10.1016/j.bmcl.2016.02.005
Zhao YN, Chen LH, Yang XL, et al., 2020, Inhibition of copper transporter-1 by ammonium tetrathiocarbolybdate in the treatment of pancreatic cancer. Sichuan Da Xue Xue Bao Yi Xue Ban, 51(5): 643–649. https://doi.org/10.12182/20200960101
Ryumon S, Okui T, Kunisada Y, et al., 2019, Ammonium tetrathiomolybdate enhances the antitumor effect of cisplatin via the suppression of ATPase copper transporting beta in head and neck squamous cell carcinoma. Oncol Rep, 42(6): 2611–2621. https://doi.org/10.3892/or.2019.7367
Chisholm CL, Wang H, Wong AHH, et al., 2016, Ammonium tetrathiomolybdate treatment targets the copper transporter ATP7A and enhances sensitivity of breast cancer to cisplatin. Oncotarget, 7(51): 84439–84452. https://doi.org/10.18632/oncotarget.12992
Chan N, Willis A, Kornhauser N, et al., 2017, Influencing the tumor microenvironment: A phase II study of copper-depletion using tetrathiomolybdate (TM) in patients with breast cancer at high risk for recurrence and in preclinical models of lung metastases. Clin Cancer Res, 23(3): 666–676. https://doi.org/10.1158/1078-0432.CCR-16-1326
Li X, Li N, Huang L, et al., 2020, Is hydrogen sulfide a concern during treatment of lung adenocarcinoma with ammonium tetrathiomolybdate? Front Oncol, 10: 234. https://doi.org/10.3389/fonc.2020.00234
Elsheikh W, Blackler RW, Flannigan KL, et al., 2014, Enhanced chemopreventive effects of a hydrogen sulfide-releasing anti-inflammatory drug (ATB-346) in experimental colorectal cancer. Nitric Oxide, 41: 131–137. https://doi.org/10.1016/j.niox.2014.04.006
De Cicco P, Panza E, Ercolano G, et al., 2016, ATB-346, a novel hydrogen sulfide-releasing anti-inflammatory drug, induces apoptosis of human melanoma cells and inhibits melanoma development in vivo. Pharmacol Res, 114: 67–73. https://doi.org/10.1016/j.phrs.2016.10.019
Kodela R, Chattopadhyay M, Velázquez-Martínez CA, et al., 2015, NOSH-aspirin (NBS-1120), a novel nitric oxide-and hydrogen sulfide-releasing hybrid has enhanced chemo-preventive properties compared to aspirin, is gastrointestinal safe with all the classic therapeutic indications. Biochem Pharmacol, 98(4): 564–572. https://doi.org/10.1016/j.bcp.2015.09.014
Chattopadhyay M, Kodela R, Kashfi K, 2013, P09 therapeutic potential of NOSH-aspirin, a dual nitric oxide-and hydrogen sulfide-donating hybrid in colon cancer. Nitric Oxide, 31: S37–S38. https://doi.org/10.1016/j.niox.2013.06.071
Kashfi K, Chattopadhyay M, Kodela R, et al., 2013 NOSH‐aspirin a Dual Nitric Oxide and Hydrogen Sulfide‐Releasing Hybrid Reciprocally Regulates NF‐κB: S‐Nitrosylation vs S‐Sulfhydration. Hoboken, New Jersey: Wiley Online Library.
Kashfi K, Borgo S, Williams JL, et al., 2005, Positional isomerism markedly affects the growth inhibition of colon cancer cells by nitric oxide-donating aspirin in vitro and in vivo. J Pharmacol Exp Ther, 312(3): 978–988. https://doi.org/10.1124/jpet.104.075994
Togashi A, Katagiri T, Ashida S, et al., 2005, Hypoxia-inducible protein 2 (HIG2), a novel diagnostic marker for renal cell carcinoma and potential target for molecular therapy. Cancer Res, 65(11): 4817–4826. https://doi.org/10.1158/0008-5472.CAN-05-0120
Huang Z, Fu J, Zhang Y, 2017, Nitric oxide donor-based cancer therapy: Advances and prospects. J Med Chem, 60(18): 7617–7635. https://doi.org/10.1021/acs.jmedchem.6b01672
Jeong CH, Ryu H, Kim DH, et al., 2019, Piperlongumine induces cell cycle arrest via reactive oxygen species accumulation and IKKβ suppression in human breast cancer cells. Antioxidants (Basel), 8(11): 553. https://doi.org/10.3390/antiox8110553
Chattopadhyay M, Kodela R, Santiago G, et al., 2020, NOSH-aspirin (NBS-1120) inhibits pancreatic cancer cell growth in a xenograft mouse model: Modulation of FoxM1, p53, NF-κB, iNOS, caspase-3 and ROS. Biochem Pharmacol, 176: 113857. https://doi.org/10.1016/j.bcp.2020.113857
Kodela R, Chattopadhyay M, Kashfi K, 2013, Synthesis and biological activity of NOSH-naproxen (AVT-219) and NOSH-sulindac (AVT-18A) as potent anti-inflammatory agents with chemotherapeutic potential. Medchemcomm, 4(11): 1472–1481. https://doi.org/10.1039/C3MD00185G
Jia H, Ye J, You J, et al., 2017, Role of the cystathionine β-synthase/H2S system in liver cancer cells and the inhibitory effect of quinolone-indolone conjugate QIC2 on the system. Oncol Rep, 37(5): 3001–3009. https://doi.org/10.3892/or.2017.5513
Kashfi K, Chattopadhyay M, Kodela R, 2015, NOSH-sulindac (AVT-18A) is a novel nitric oxide-and hydrogen sulfide-releasing hybrid that is gastrointestinal safe and has potent anti-inflammatory, analgesic, antipyretic, anti-platelet, and anti-cancer properties. Redox Biol, 6: 287–296. https://doi.org/10.1016/j.redox.2015.08.012
You J, Shi X, Liang H, et al., 2017, Cystathionine-γ-lyase promotes process of breast cancer in association with STAT3 signaling pathway. Oncotarget, 8(39): 65677–65686. https://doi.org/10.18632/oncotarget.20057
You J, Ma M, Ye J, et al., 2017, Down-Regulation of Cystathionine-γ-lyase/H2S System Inhibits Cell Growth in Human Breast Cancer MDA-MB-231 Cells. In: BIO Web of Conferences. EDP Sciences.
Chakraborty PK, Xiong X, Mustafi SB, et al., 2015, Role of cystathionine beta synthase in lipid metabolism in ovarian cancer. Oncotarget, 6(35): 37367–37384. https://doi.org/10.18632/oncotarget.5424 242.
Liu Y, Wang L, Zhang X, et al., 2021, A novel cystathionine γ-lyase inhibitor, I194496, inhibits the growth and metastasis of human TNBC via downregulating multiple signaling pathways. Sci Rep, 11(1): 1–13.
Ye F, Li X, Sun K, et al., 2020, Inhibition of endogenous hydrogen sulfide biosynthesis enhances the anti-cancer effect of 3, 3’-diindolylmethane in human gastric cancer cells. Life Sci, 261: 118348. https://doi.org/10.1016/j.lfs.2020.118348
Yin P, Zhao C, Li Z, et al., 2012, Sp1 is involved in regulation of cystathionine γ-lyase gene expression and biological function by PI3K/Akt pathway in human hepatocellular carcinoma cell lines. Cell Signal, 24(6): 1229–1240. https://doi.org/10.1016/j.cellsig.2012.02.003
Pan Y, Ye S, Yuan D, et al., 2014, Hydrogen sulfide (H2S)/ cystathionine γ-lyase (CSE) pathway contributes to the proliferation of hepatoma cells. Mutat Res, 763–764: 10–18. https://doi.org/10.1016/j.mrfmmm.2014.03.002
Pan Y, Zhou C, Yuan D, et al., 2016, Radiation exposure promotes hepatocarcinoma cell invasion through epithelial mesenchymal transition mediated by H2S/CSE pathway. Radiat Res, 185(1): 96–105. https://doi.org/10.1667/RR14177.1
Yang D, Li T, Li Y, et al., 2019, H2S suppresses indoleamine 2, 3-dioxygenase 1 and exhibits immunotherapeutic efficacy in murine hepatocellular carcinoma. J Exp Clin Cancer Res, 38(1): 88. https://doi.org/10.1186/s13046-019-1083-5
Fan K, Li N, Qi J, et al., 2014, Wnt/β-catenin signaling induces the transcription of cystathionine-γ-lyase, a stimulator of tumor in colon cancer. Cell Signal, 26(12): 2801–2808. https://doi.org/10.1016/j.cellsig.2014.08.023
Wang YH, Huang JT, Chen WL, et al., 2019, Dysregulation of cystathionine γ‐lyase promotes prostate cancer progression and metastasis. EMBO Rep, 20(10): e45986. https://doi.org/10.15252/embr.201845986
Kawahara B, Moller T, Hu-Moore K, et al., 2017, Attenuation of antioxidant capacity in human breast cancer cells by carbon monoxide through inhibition of cystathionine β-synthase activity: implications in chemotherapeutic drug sensitivity. J Med Chem, 60(19): 8000–8010. https://doi.org/10.1021/acs.jmedchem.7b00476
Zhang L, Qi Q, Yang J, et al., 2015, An anticancer role of hydrogen sulfide in human gastric cancer cells. Oxid Med Cell Longev, 2015: 636410. https://doi.org/10.1155/2015/636410
Wang L, Cai H, Hu Y, et al., 2018, A pharmacological probe identifies cystathionine β-synthase as a new negative regulator for ferroptosis. Cell Death Dis, 9(10): 1005. https://doi.org/10.1038/s41419-018-1063-2
Sanokawa-Akakura R, Ostrakhovitch EA, Akakura S, et al., 2014, A H2S-Nampt dependent energetic circuit is critical to survival and cytoprotection from damage in cancer cells. PloS One, 9(9): e108537. https://doi.org/10.1371/journal.pone.0108537
Kim J, Hong SJ, Park JH, et al., 2009, Expression of cystathionine β-synthase is downregulated in hepatocellular carcinoma and associated with poor prognosis. Oncol Rep, 21(6): 1449–1454. https://doi.org/10.3892/or_00000373
Zhang J, Xie Y, Xu Y, et al., 2011, Hydrogen sulfide contributes to hypoxia-induced radioresistance on hepatoma cells. J Radiat Res, 52(5): 622–628. https://doi.org/10.1269/jrr.11004
Wang L, Han H, Liu Y, et al., 2018, Cystathionine β-synthase induces multidrug resistance and metastasis in hepatocellular carcinoma. Curr Mol Med, 18(7): 496–506. https://doi.org/10.2174/1566524019666181211162754
Wang L, Yang Z, Wu Z, et al., 2020, Increased expression of cystathionine beta-synthase and chemokine ligand 21 is closely associated with poor prognosis in extrahepatic cholangiocarcinoma. Medicine (Baltimore), 99(38): e22255. https://doi.org/10.1097/MD.0000000000022255
Liu N, Lin X, Huang C, 2020, Activation of the reverse transsulfuration pathway through NRF2/CBS confers erastin-induced ferroptosis resistance. Br J Cancer, 122(2): 279–292.
Santos I, Ramos C, Mendes C, et al., 2019, Targeting glutathione and cystathionine β-synthase in ovarian cancer treatment by selenium–chrysin polyurea dendrimer nanoformulation. Nutrients, 11(10): 2523. https://doi.org/10.3390/nu11102523
Chao C, Zatarain JR, Ding Y, et al., 2016, Cystathionine-β- synthase inhibition for colon cancer: Enhancement of the efficacy of aminooxyacetic acid via the prodrug approach. Mol Med, 22(1): 361–379. https://doi.org/10.2119/molmed.2016.00102
Szabo C, Coletta C, Chao C, et al., 2013, Tumor-derived hydrogen sulfide, produced by cystathionine-β- synthase, stimulates bioenergetics, cell proliferation, and angiogenesis in colon cancer. Proc Natl Acad Sci, 110(30): 12474–12479. https://doi.org/10.1073/pnas.1306241110
Ascenção K, Dilek N, Augsburger F, et al., 2021, Pharmacological induction of mesenchymal-epithelial transition via inhibition of H2S biosynthesis and consequent suppression of ACLY activity in colon cancer cells. Pharmacol Res, 165: 105393. https://doi.org/10.1016/j.phrs.2020.105393
Niu W, Chen F, Wang J, et al., 2018, Antitumor effect of sikokianin C, a selective cystathionine β-synthase inhibitor, against human colon cancer in vitro and in vivo. Medchemcomm, 9(1): 113–120. https://doi.org/10.1039/c7md00484b
Zhang M, Li J, Huang B, et al., 2020, Cystathionine β synthase/hydrogen sulfide signaling in multiple myeloma regulates cell proliferation and apoptosis. J Environ Pathol Toxicol Oncol, 39(3): 281–290. https://doi.org/10.1615/JEnvironPatholToxicolOncol.2020034851
Govar AA, Törő G, Szaniszlo P, et al., 2020, 3‐Mercaptopyruvate sulfurtransferase supports endothelial cell angiogenesis and bioenergetics. Br J Pharmacol, 177(4): 866–883. https://doi.org/10.1111/bph.14574
Augsburger F, Randi EB, Jendly M, et al., 2020, Role of 3-mercaptopyruvate sulfurtransferase in the regulation of proliferation, migration, and bioenergetics in murine colon cancer cells. Biomolecules, 10(3): 447. https://doi.org/10.3390/biom10030447
Bantzi M, Augsburger F, Loup J, et al., 2021, Novel aryl-substituted pyrimidones as inhibitors of 3-mercaptopyruvate sulfurtransferase with antiproliferative efficacy in colon cancer. J Med Chem, 64(9): 6221–6240. https://doi.org/10.1021/acs.jmedchem.1c00260
Bronowicka-Adamska P, Bentke A, Lasota M, et al., 2020, Effect of S-allyl–l-cysteine on MCF-7 cell line 3-mercaptopyruvate sulfurtransferase/sulfane sulfur system, viability and apoptosis. Int J Mol Sci, 21(3): 1090.