Compressive properties and fatigue performance of NiTi lattice structures optimized by TPMS
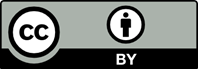
Nickel titanium (NiTi) lattice structures prepared by laser powder bed fusion (LPBF) have great application potential, due to their unique shape memory effect, superelasticity, and controlled geometry characteristics. In this study, the NiTi I-graph-wrapped package (I-WP) and NiTi body-centered cubic (BCC) lattice structure samples were prepared by the LPBF process. The uniaxial compression properties and cyclic compression properties of NiTi lattice structure samples prepared by LPBF process were studied. The results showed that the surfaces of NiTi lattice structures were very rough and adhered with many powder particles. The sample optimization design through the minimal surface could effectively improve the mechanical properties and deformation resistance and change the surface morphology of fracture surfaces in high cycle fatigue. The I-WP lattice structure had a higher Young’s modulus and yield strength. The fatigue strength improved from 1.88 MPa (BCC structure lattice) to 2.08 MPa (I-WP structure lattice). The simulation was performed to investigate the mechanism underlying the improvement in fatigue strength, revealing that optimization of surface stress distribution could be the plausible reason. In general, this study provides valuable guidance for the preparation and design of NiTi lattice structure by the LPBF process.
Chunze Yan serves as the Editorial Board Member of the journal, but did not in any way involve in the editorial and peer-review process conducted for this paper, directly or indirectly.
- Bagheri A, Mahtabi MJ, Shamsaei N. Fatigue behavior and cyclic deformation of additive manufactured NiTi. J Mater Process Technol. 2018;252:440-453. doi: 10.1016/j.jmatprotec.2017.10.006
- Gu H, Bumke L, Chluba C, Quandt E, James RD. Phase engineering and supercompatibility of shape memory alloys. Mater Today. 2018;21(3):265-277. doi: 10.1016/j.mattod.2017.10.002
- Molod MA, Spyridis P, Barthold FJ. Applications of shape memory alloys in structural engineering with a focus on concrete construction - A comprehensive review. Constr Build Mater. 2022;337:127565. doi: 10.1016/j.conbuildmat.2022.127565
- Fang C, Liang D, Zheng Y, Yam MCH, Sun R. Rocking bridge piers equipped with shape memory alloy (SMA) washer springs. Eng Struct. 2020;214:110651. doi: 10.1016/j.engstruct.2020.110651
- Xie W, Quinn J, Zhang J, Carson L, Chan CW. Control of laser-gas-material interactions to enhance the surface properties of NiTi for orthopaedic applications. Surf Coat Technol. 2021;421:127403. doi: 10.1016/j.surfcoat.2021.127403
- Yang X, Yang Q, Shi Y, et al. Effect of volume fraction and unit cell size on manufacturability and compressive behaviors of Ni-Ti triply periodic minimal surface lattices. Addit Manuf. 2022;54:102737. doi: 10.1016/j.addma.2022.102737
- Zhang C, Jin J, He M, Yang L. Compressive mechanics and hyperelasticity of Ni-Ti lattice structures fabricated by
- Sefene EM. State-of-the-art of selective laser melting process: A comprehensive review. J Manuf Syst. 2022;63:250-274. doi: 10.1016/j.jmsy.2022.04.002
- Liu C, Roux LL, Ji Z, Kerfriden P, Lacan F, Bigot S. Machine learning-enabled feedback loops for metal powder bed fusion additive manufacturing. Procedia Comput Sci. 2020;176:2586-2595. doi: 10.1016/j.procs.2020.09.314
- Han Q, Gu Y, Huang J, et al. Selective laser melting of hastelloy X nanocomposite: Effects of TiC reinforcement on crack elimination and strength improvement. Compos B Eng. 2020;202:108442. doi: 10.1016/j.compositesb.2020.108442
- Zhou SY, Su Y, Wang H, Enz J, Ebel T, Yan M. Selective laser melting additive manufacturing of 7xxx series Al-Zn- Mg-Cu alloy: Cracking elimination by co-incorporation of Si and TiB2. Addit Manuf. 2020;36:101458. doi: 10.1016/j.addma.2020.101458
- Hussain S, Alagha AN, Haidemenopoulos GN, Zaki W. Microstructural and surface analysis of NiTi TPMS lattice sections fabricated by laser powder bed fusion. J Manuf Process. 2023;102:375-386. doi: 10.1016/j.jmapro.2023.07.055
- Finazzi V, Berti F, Petrini L, Previtali B, Demir AG. Additive manufacturing and post-processing of superelastic NiTi micro struts as building blocks for cardiovascular stents. Addit Manuf. 2023;70:103561. doi: 10.1016/j.addma.2023.103561
- Wang J, Huang B, Gu X, Zhu J, Zhang W. Actuation performance of machined helical springs from NiTi shape memory alloy. Int J Mech Sci. 2022;236:107744. doi: 10.1016/j.ijmecsci.2022.107744
- Luo J, Xu K, Li C, Li M, Lin Y. The evolution of dynamic recrystallization and recrystallization texture during isothermal compression of NiTi shape memory alloy. Mater Sci Eng A. 2021;820:141424. doi: 10.1016/j.msea.2021.141424
- Kan Q, Zhang Y, Xu Y, Kang G, Yu C. Tension-compression asymmetric functional degeneration of super-elastic NiTi shape memory alloy: Experimental observation and multiscale constitutive model. Int J Solids Struct. 2023;280:112384. doi: 10.1016/j.ijsolstr.2023.112384
- Safdel A, Zaker N, Botton GA, Elbestawi MA. The role of texture and restoration mechanisms in defining the tension-compression asymmetry behavior of aged NiTi alloys fabricated by laser powder bed fusion. Mater Sci Eng A. 2023;864:144592. doi: 10.1016/j.msea.2023.144592
- Wu Y, Ertekin E, Sehitoglu H. Elastocaloric cooling capacity of shape memory alloys - Role of deformation temperatures, mechanical cycling, stress hysteresis and inhomogeneity of transformation. Acta Mater. 2017;135:158-176. doi: 10.1016/j.actamat.2017.06.012
- Zhang K, Kang G, Sun Q. High fatigue life and cooling efficiency of NiTi shape memory alloy under cyclic compression. Scr Mater. 2019;159:62-67. doi: 10.1016/j.scriptamat.2018.09.012
- Zhao T, Kang G. Experimental study and life prediction on fatigue failure of NiTi shape memory alloy under multi-axial one-way shape memory cyclic loadings. Int J Fatigue. 2022;155:106609. doi: 10.1016/j.ijfatigue.2021.106609
- Jin J, Wu S, Yang L, et al. Ni-Ti multicell interlacing Gyroid lattice structures with ultra-high hyperelastic response fabricated by laser powder bed fusion. Int J Mach Tools Manuf. 2024;195:104099. doi: 10.1016/j.ijmachtools.2023.104099
- Song W, Mu K, Feng G, et al. Mechanical properties of 3D printed interpenetrating phase composites with TPMS architectures. Thin Walled Struct. 2023;193:111210. doi: 10.1016/j.tws.2023.111210
- Gado MG, Ookawara S. 3D-printed Triply Periodic Minimal Surface (TPMS) structures: Towards potential application of adsorption-based atmospheric water harvesting. Energy Convers Manag. 2023;297:117729. doi: 10.1016/j.enconman.2023.117729
- Yang L, Li Y, Chen Y, Yan C, Liu B, Shi Y. Topologically optimized lattice structures with superior fatigue performance. Int J Fatigue. 2022;165:107188. doi: 10.1016/j.ijfatigue.2022.107188
- Zhang C, Qiao H, Yang L, et al. Vibration characteristics of additive manufactured IWP-type TPMS lattice structures. Compos Struct. 2024;327:117642. doi: 10.1016/j.compstruct.2023.117642
- Fu H, Kaewunruen S. Experimental and DEM investigation of axially-loaded behaviours of IWP-based structures. Int J Mech Sci. 2022;235:107738. doi: 10.1016/j.ijmecsci.2022.107738
- Ge J, Yuan B, Chen H, et al. Anisotropy in microstructural features and tensile performance of laser powder bed fusion NiTi alloys. J Mater Res Technol. 2023;24:8656-8668. doi: 10.1016/j.jmrt.2023.05.046
- Qi Y, Sha P, Yang K, et al. Construction and parameter optimization of LPBF-NiTi alloy bionic superhydrophobic surface based on laser processing. J Mater Res Technol. 2023;24:9462-9475. doi: 10.1016/j.jmrt.2023.05.162
- Yang L, Yan C, Fan H, et al. Investigation on the orientation dependence of elastic response in Gyroid cellular structures. J Mech Behav Biomed Mater. 2019;90:73-85. doi: 10.1016/j.jmbbm.2018.09.042
- International Organization for Standardization. ISO 13314:2011, Mechanical Testing of Metals--Ductility Testing- -Compression Test for Porous and Cellular Metals. Geneva: International Organization for Standardization; 2011. p. 1-7.
- Chen W, Yang Q, Huang S, Kruzic JJ, Li X. Compression behavior of graded NiTi gyroid-structures fabricated by laser powder bed fusion additive manufacturing under monotonic and cyclic loading. JOM. 2021;73(12):4154-4165. doi: 10.1007/s11837-021-04938-x
- Yang L, Wu S, Yan C, et al. Fatigue properties of Ti-6Al-4V Gyroid graded lattice structures fabricated by laser powder bed fusion with lateral loading. Addit Manuf. 2021;46:102214. doi: 10.1016/j.addma.2021.102214
- Jiang H, Wang X, Xi R, et al. Size effect on the microstructure, phase transformation behavior, and mechanical properties of NiTi shape memory alloys fabricated by laser powder bed fusion. J Mater Sci Technol. 2023;157:200-212. doi: 10.1016/j.jmst.2023.02.026
- Zheng N, Zhai X, Chen F. Topology optimization of Self-supporting porous structures based on triply periodic minimal surfaces. Comput Aided Des. 2023;161:103542. doi: 10.1016/j.cad.2023.103542
- Hu J, Wang S, Wang Y, Li F, Luo Z. A lightweight methodology of 3D printed objects utilizing multi-scale porous structures. Vis Comput. 2019;35:949-959. doi: 10.1007/s00371-019-01672-z
- Chen W, Gu D, Yang J, Yang Q, Chen J, Shen X. Compressive mechanical properties and shape memory effect of NiTi gradient lattice structures fabricated by laser powder bed fusion. Int J Extrem Manuf. 2022;4(4):045002. doi: 10.1088/2631-7990/ac8ef3
- Sun L, Chen K, Geng P, Zhou Y, Wen S, Shi Y. Mechanical and shape memory properties of NiTi triply periodic minimal surface structures fabricated by laser powder bed fusion. J Manuf Process. 2023;101:1091-1100. doi: 10.1016/j.jmapro.2023.06.034
- Yang L, Mertens R, Ferrucci M, Yan C, Shi Y, Yang S. Continuous graded Gyroid cellular structures fabricated by selective laser melting: Design, manufacturing and mechanical properties. Mater Des. 2019;162:394-404. doi: 10.1016/j.matdes.2018.12.007
- Loginov YN, Koptyug A, Popov VV Jr., et al. Compression deformation and fracture behavior of additively manufactured Ti-6Al-4V cellular structures. Int J Lightweight Mater Manuf. 2022;5(1):126-135. doi: 10.1016/j.ijlmm.2021.11.003
- Al-Saedi DSJ, Masood SH, Faizan-Ur-Rab M, Alomarah A, Ponnusamy P. Mechanical properties and energy absorption capability of functionally graded F2BCC lattice fabricated by SLM. Mater Des. 2018;144:32-44. doi: 10.1016/j.matdes.2018.01.059
- Bian Y, Li P, Yang F, Wang P, Li W, Fan H. Deformation mode and energy absorption of polycrystal-inspired square-cell lattice structures. Appl Math Mech. 2020;41(10):1561-1582. doi: 10.1007/s10483-020-2648-8
- Li QM, Magkiriadis I, Harrigan JJ. Compressive strain at the onset of densification of cellular solids. J Cell Plast. 2006;42(5):371-392. doi: 10.1177/0021955x06063519
- Prevéy PS, Cammett JT. The influence of surface enhancement by low plasticity burnishing on the corrosion fatigue performance of AA7075-T6. Int J Fatigue. 2004;26(9):975-982. doi: 10.1016/j.ijfatigue.2004.01.010
- Nemat-Nasser S, Guo WG. Superelastic and cyclic response of NiTi SMA at various strain rates and temperatures. Mech Mater. 2006;38(5):463-474. doi: 10.1016/j.mechmat.2005.07.004
- Gall K, Maier HJ. Cyclic deformation mechanisms in precipitated NiTi shape memory alloys. Acta Mater. 2002;50(18):4643-4657. doi: 10.1016/S1359-6454(02)00315-4
- Sun ZP, Guo YB, Shim VPW. Deformation and energy absorption characteristics of additively-manufactured polymeric lattice structures - Effects of cell topology and material anisotropy. Thin-Walled Struct. 2021;169:108420. doi: 10.1016/j.tws.2021.108420
- Otsuka K, Ren X. Physical metallurgy of Ti-Ni-based shape memory alloys. Prog Mater Sci. 2005;50(5):511-678. doi: 10.1016/j.pmatsci.2004.10.001
- Yang L, Yan C, Cao W, et al. Compression-compression fatigue behaviour of gyroid-type triply periodic minimal surface porous structures fabricated by selective laser melting. Acta Mater. 2019;181:49-66. doi: 10.1016/j.actamat.2019.09.042
- Zhao S, Li SJ, Wang SG, et al. Compressive and fatigue behavior of functionally graded Ti-6Al-4V meshes fabricated by electron beam melting. Acta Mater. 2018;150:1-15. doi: 10.1016/j.actamat.2018.02.060
- International Organization for Standardization. Metallic Materials--Fatigue Testing--Axial Force-controlled Method. Geneva: International Organization for Standardization; 2006.
- Li SJ, Murr LE, Cheng XY, et al. Compression fatigue behavior of Ti-6Al-4V mesh arrays fabricated by electron beam melting. Acta Mater. 2012;60(3):793-802. doi: 10.1016/j.actamat.2011.10.051
- Speirs M, Van Hooreweder B, Van Humbeeck J, Kruth JP. Fatigue behaviour of NiTi shape memory alloy scaffolds produced by SLM, a unit cell design comparison. J Mech Behav Biomed Mater. 2017;70:53-59. doi: 10.1016/j.jmbbm.2017.01.016
- Zhu ML, Jin L, Xuan FZ. Fatigue life and mechanistic modeling of interior micro-defect induced cracking in high cycle and very high cycle regimes. Acta Mater. 2018;157:259-275. doi: 10.1016/j.actamat.2018.07.036
- Swain MV, Lawn BR, Burns SJ. Cleavage step deformation in brittle solids. J Mater Sci. 1974;9(2):175-183. doi: 10.1007/BF00550939