3D-printed degradable hair follicular hanging-drop scaffolds integrated with tissue-engineered skin promote hair follicle regeneration in vitro
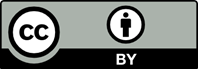
Currently, a substantial number of patients suffer from the distress of skin defects. Tissue-engineered skin represents a promising alternative therapeutic option for these patients. However, the currently available tissue-engineered skin products still exhibit significant limitations, including poor long-term viability and a lack of appendages. The advent of three-dimensional (3D) printing technology and organoid formation techniques provides a promising avenue for addressing these challenges. In this study, we constructed a scaffold using 3D printing with sodium alginate (NaAlg)/gelatin (GEL)/alginate lyase hydrogel, which has controllable pore size and degradation rate. This scaffold provides a favorable microenvironment for the colonization and functional maturation of hair follicular hanging drops within artificially organized skin. In vitro observations revealed the regeneration of hair follicles and the high expression of hair follicle-specific markers, LIM homeobox 2 (LHX2), and cytokeratin 17 (CK17). In addition, we studied the follicular polarization of the engineered skin compared to normal skin and attempted to identify possible underlying mechanisms. In conclusion, our findings present a novel strategy for establishing artificial skin with appendages.

- Singer AJ, Dagum AB. Current management of acute cutaneous wounds. N Engl J Med. 2008;359(10):1037-1046. doi: 10.1056/NEJMra0707253
- Hall J, Buckley HL, Lamb KA, et al. Point prevalence of complex wounds in a defined United Kingdom population. Wound Repair Regen. 2014;22(6):694-700. doi: 10.1111/wrr.12230
- Jiang Y, Huang S, Fu X, et al. Epidemiology of chronic cutaneous wounds in China. Wound Repair Regen. 2011;19(2):181-188. doi: 10.1111/j.1524-475X.2010.00666.x
- Kabashima K, Honda T, Ginhoux F, et al. The immunological anatomy of the skin. Nat Rev Immunol. 2019; 19(1):19-30. doi: 10.1038/s41577-018-0084-5
- Baker LB. Physiology of sweat gland function: the roles of sweating and sweat composition in human health. Temperature (Austin). 2019;6(3):211-259. doi: 10.1080/23328940.2019.1632145
- Sierra-Sánchez Á, Kim KH, Blasco-Morente G, et al. Cellular human tissue-engineered skin substitutes investigated for deep and difficult to heal injuries. NPJ Regen Med. 2021;6(1):1-23. doi: 10.1038/s41536-021-00144-0
- Sierra-Sánchez Á, Fernández-González A, Lizana-Moreno A, et al. Hyaluronic acid biomaterial for human tissue-engineered skin substitutes: preclinical comparative in vivo study of wound healing. J Eur Acad Dermatol Venereol. 2020;34(10):2414-2427. doi: 10.1111/jdv.16342
- Mansbridge JN. Tissue-engineered skin substitutes in regenerative medicine. Curr Opin Biotechnol. 2009;20(5):563-567. doi: 10.1016/j.copbio.2009.08.008
- Nourian Dehkordi A, Mirahmadi Babaheydari F, Chehelgerdi M, et al. Skin tissue engineering: wound healing based on stem-cell-based therapeutic strategies. Stem Cell Res Ther. 2019;10(1):111. doi: 10.1186/s13287-019-1212-2
- Costello L, Dicolandrea T, Tasseff R, et al. Tissue engineering strategies to bioengineer the ageing skin phenotype in vitro. Aging Cell. 2022;21(2):e13550. doi: 10.1111/acel.13550
- Chen J, Fan Y, Dong G, et al. Designing biomimetic scaffolds for skin tissue engineering. Biomater Sci. 2023;11(9):3051-3076. doi: 10.1039/d3bm00046j
- Amirsadeghi A, Jafari A, Eggermont LJ, et al. Vascularization strategies for skin tissue engineering. Biomater Sci. 2020;8(15):4073-4094. doi: 10.1039/d0bm00266f
- Bock O, Schmid-Ott G, Malewski P, et al. Quality of life of patients with keloid and hypertrophic scarring. Arch Dermatol Res. 2006;297(10):433-438. doi: 10.1007/s00403-006-0651-7
- Lei M, Schumacher LJ, Lai Y-C, et al. Self-organization process in newborn skin organoid formation inspires strategy to restore hair regeneration of adult cells. Proc Natl Acad Sci U S A. 2017;114(34):E7101-E7110. doi: 10.1073/pnas.1700475114
- Abaci HE, Coffman A, Doucet Y, et al. Tissue engineering of human hair follicles using a biomimetic developmental approach. Nat Commun. 2018;9(1):5301. doi: 10.1038/s41467-018-07579-y
- Huang S, Xu Y, Wu C, et al. In vitro constitution and in vivo implantation of engineered skin constructs with sweat glands. Biomaterials. 2010;31(21):5520-5525. doi: 10.1016/j.biomaterials.2010.03.060
- Huang S, Yao B, Xie J, et al. 3D bioprinted extracellular matrix mimics facilitate directed differentiation of epithelial progenitors for sweat gland regeneration. Acta Biomater. 2016;32:170-177. doi: 10.1016/j.actbio.2015.12.039
- Kang MS, Kwon M, Park R, et al. Harnessing the intradermal delivery of hair follicle dermal papilla cell spheroids for hair follicle regeneration in nude mice. Biomater Res. 2025;29:0129. doi: 10.34133/bmr.0129
- Zheng Y, Du X, Wang W, et al. Organogenesis from dissociated cells: generation of mature cycling hair follicles from skin-derived cells. J Invest Dermatol. 2005;124(5):867-876. doi: 10.1111/j.0022-202X.2005.23716.x
- Wang Y, Liu T, Guo Y, et al. Integrating melt electrospinning writing and microfluidics to engineer a human cardiac microenvironment for high-fidelity drug screening. Bioact Mater. 2025;45:551-566. doi: 10.1016/j.bioactmat.2024.11.037
- Carlson MW, Alt-Holland A, Egles C, et al. Three-dimensional tissue models of normal and diseased skin. Curr Protoc Cell Biol. 2008, Chapter 19: Unit 19.9. doi: 10.1002/0471143030.cb1909s41
- Gupta K, Levinsohn J, Linderman G, et al. Single-cell analysis reveals a hair follicle dermal niche molecular differentiation trajectory that begins prior to morphogenesis. Dev Cell. 2019;48(1):17-31.e6. doi: 10.1016/j.devcel.2018.11.032
- Lin B, Miao Y, Wang J, et al. Surface tension guided hanging-drop: producing controllable 3D spheroid of high-passaged human dermal papilla cells and forming inductive microtissues for hair-follicle regeneration. ACS Appl Mater Interfaces. 2016;8(9):5906-5916. doi: 10.1021/acsami.6b00202
- Kalabusheva E, Terskikh V, Vorotelyak E. Hair germ model in vitro via human postnatal keratinocyte-dermal papilla interactions: impact of hyaluronic acid. Stem Cells Int. 2017;2017:9271869. doi: 10.1155/2017/9271869
- Wang J, Liu S, Huang J, et al. Alginate: microbial production, functionalization, and biomedical applications. Int J Biol Macromol. 2023;242:125048. doi: 10.1016/j.ijbiomac.2023.125048
- Hecht H, Srebnik S. Structural characterization of sodium alginate and calcium alginate. Biomacromolecules. 2016;17(6):2160-2167. doi: 10.1021/acs.biomac.6b00378
- Mallakpour S, Mohammadi N. Development of sodium alginate-pectin/TiO2 nanocomposites: antibacterial and bioactivity investigations. Carbohydr Polym. 2022;285:119226. doi: 10.1016/j.carbpol.2022.119226
- Sanchez-Ballester NM, Bataille B, Soulairol I. Sodium alginate and alginic acid as pharmaceutical excipients for tablet formulation: structure-function relationship. Carbohydr Polym. 2021;270:118399. doi: 10.1016/j.carbpol.2021.118399
- Do UT, Kim J, Luu QS, et al. Accurate detection of enzymatic degradation processes of gelatin–alginate microcapsule by 1H NMR spectroscopy: probing biodegradation mechanism and kinetics. Carbohydr Polym. 2023;304:120490. doi: 10.1016/j.carbpol.2022.120490
- Chen C, Li X, Lu C, et al. Advances in alginate lyases and the potential application of enzymatic prepared alginate oligosaccharides: a mini review. Int J Biol Macromol. 2024;260:129506. doi: 10.1016/j.ijbiomac.2024.129506
- Ma J, Qin C, Wu J, et al. 3D multicellular micropatterning biomaterials for hair regeneration and vascularization. Mater Horiz. 2023;10(9):3773-3784. doi: 10.1039/d3mh00528c
- Motter Catarino C, Cigaran Schuck D, Dechiario L, et al. Incorporation of hair follicles in 3D bioprinted models of human skin. Sci Adv. 2023;9(41):eadg0297. doi: 10.1126/sciadv.adg0297
- Yao B, Hu T, Cui X, et al. Enzymatically degradable alginate/ gelatin bioink promotes cellular behavior and degradation in vitro and in vivo. Biofabrication. 2019;11(4):045020. doi: 10.1088/1758-5090/ab38ef
- Lee JJ, Lee S-G, Park JC, et al. Investigation on biodegradable PLGA scaffold with various pore size structure for skin tissue engineering. Curr Appl Phys. 2007;7:e37-e40. doi: 10.1016/j.cap.2006.11.011
- Zhang Z, Feng Y, Wang L, et al. A review of preparation methods of porous skin tissue engineering scaffolds. Mater Today Commun. 2022;32:104109. doi: 10.1016/j.mtcomm.2022.104109
- Han J, Wu J, Xiang X, et al. Biodegradable BBG/PCL composite scaffolds fabricated by selective laser sintering for directed regeneration of critical-sized bone defects. Mater Design. 2023;225:111543. doi: 10.1016/j.matdes.2022.111543
- Cao M, Zhao J, Du L, et al. The combination of hair follicle-specific marker LHX2 and co-expressed marker can distinguish between sweat gland placodes and hair placodes in rat. J Mol Histol. 2021;52(6):1225-1232. doi: 10.1007/s10735-021-10023-z
- Tomann P, Paus R, Millar SE, et al. Lhx2 is a direct NF-κB target gene that promotes primary hair follicle placode down-growth. Development. 2016; 143(9):1512-1522. doi: 10.1242/dev.130898
- Rhee H, Polak L, Fuchs E. Lhx2 maintains stem cell character in hair follicles. Science. 2006;312(5782):1946-1949. doi: 10.1126/science.1128004
- Villeneuve C, Hashmi A, Ylivinkka I, et al. Mechanical forces across compartments coordinate cell shape and fate transitions to generate tissue architecture. Nature Cell Biol. 2024;26(2):207-218. doi: 10.1038/s41556-023-01332-4