Computational investigation of a 3D-printed osteochondral interface scaffold with comprehensive interfacial mechanical properties
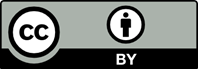
Osteoarthritis is a highly prevalent rheumatic musculoskeletal disorder that often results in both physiological dysfunction and psychological distress. Although many tissue engineering approaches have been proposed for tissue regeneration, the mechanical properties of many scaffolds remain insufficient to fully meet the mechanical demands within the joint. This paper presents a computational investigation and structural design for creating an osteochondral interface scaffold using extrusion-based 3D printing. A finite element method-based mechanics simulation model was employed to evaluate the effects of load-bearing forces on various scaffold designs, enabling the analysis of stress distribution within the biomimetic osteochondral interface. Several structures of osteochondral interface scaffolds were fabricated, demonstrating controllable mechanical properties. The proposed biomimetic osteochondral interface manifested comprehensive mechanical performance, with bonding strength, compressive strength, and shear strength reaching 128, 277, and 276 kPa, respectively. Our research can benefit the future development in tissue engineering and regenerative medicine.

- Nukavarapu SP, Dorcemus DL. Osteochondral tissue engineering: current strategies and challenges. Biotechnol Adv. 2013;31(5):706-721. doi: 10.1016/j.biotechadv.2012.11.004
- Lawrence RC, Felson DT, Helmick CG, et al. Estimates of the prevalence of arthritis and other rheumatic conditions in the United States: part II. Arthritis Rheum. 2008;58(1):26-35. doi: 10.1002/art.23176
- James SL, Abate D, Abate KH, et al. Global, regional, and national incidence, prevalence, and years lived with disability for 354 diseases and injuries for 195 countries and territories, 1990–2017: a systematic analysis for the Global Burden of Disease Study 2017. Lancet. 2018;392(10159):1789-1858. doi: 10.1016/S0140-6736(18)32279-7
- Zhang Y, Jordan JM. Epidemiology of osteoarthritis. Clin Geriatr Med. 2010;26(3):355-369. doi: 10.1016/j.cger.2010.03.001
- Safiri S, Kolahi A, Smith E, et al. Global, regional and national burden of osteoarthritis 1990-2017: a systematic analysis of the Global Burden of Disease Study 2017. Ann Rheum Dis. 2020;79(6):819-828. doi: 10.1136/annrheumdis-2019-216515
- Hasegawa Y, Iwata H, Mizuno M, Genda E, Sato S, Miura T. The natural course of osteoarthritis of the hip due to subluxation or acetabular dysplasia. Arch Orthop Traum Surg. 1992;111(4):187-191. doi: 10.1007/BF00571474
- Jeon OH, David N, Campisi J, Elisseeff JH. Senescent cells and osteoarthritis: a painful connection. J Clin Invest. 2018;128(4):1229-1237. doi: 10.1172/JCI95147
- Wang Z, Le H, Wang Y, et al. Instructive cartilage regeneration modalities with advanced therapeutic implantations under abnormal conditions. Bioact Mater. 2022;11:317-338. doi: 10.1016/j.bioactmat.2021.10.002
- Nguyen D, Hägg DA, Forsman A, et al. Cartilage tissue engineering by the 3D bioprinting of iPS cells in a nanocellulose/alginate bioink. Sci Rep. 2017;7(1):658. doi: 10.1038/s41598-017-00690-y
- Walter H, Kawashima A, Nebelung W, Neumann W, Roessner A. Immunohistochemical analysis of several proteolytic enzymes as parameters of cartilage degradation. Pathol Res Pract. 1998;194(2):73-81. doi: 10.1016/S0344-0338(98)80073-3
- Hu Y, Chen X, Wang S, Jing Y, Su J. Subchondral bone microenvironment in osteoarthritis and pain. Bone Res. 2021;9(1):20. doi: 10.1038/s41413-021-00147-z
- Li G, Yin J, Gao J, et al. Subchondral bone in osteoarthritis: insight into risk factors and microstructural changes. Arthritis Res Ther. 2013;15(6):223. doi: 10.1186/ar4405
- Mandl LA. Osteoarthritis year in review 2018: clinical. Osteoarthr Cartilage. 2019;27(3):359-364. doi: 10.1016/j.joca.2018.11.001
- Steadman JR, Rodkey WG, Rodrigo JJ. Microfracture: surgical technique and rehabilitation to treat chondral defects. Clin Orthop Relat Res. 2001;391(391 Suppl):S362-S369. doi: 10.1097/00003086-200110001-00033
- Chimutengwende-Gordon M, Donaldson J, Bentley G. Current solutions for the treatment of chronic articular cartilage defects in the knee. EFORT Open Rev. 2020;5(3):156-163. doi: 10.1302/2058-5241.5.190031
- Bhosale AM, Richardson JB. Articular cartilage: structure, injuries and review of management. Brit Med Bull. 2008;87(1):77-95. doi: 10.1093/bmb/ldn025
- Di Luca A, Lorenzo Moldero I, Mota C, et al. Tuning cell differentiation into a 3D scaffold presenting a pore shape gradient for osteochondral regeneration. Adv Healthc Mater. 2016;5(14):1753-1763. doi: 10.1002/adhm.201600083
- Di Luca A, Longoni A, Criscenti G, et al. Surface energy and stiffness discrete gradients in additive manufactured scaffolds for osteochondral regeneration. Biofabrication. 2016;8(1):015014. doi: 10.1088/1758-5090/8/1/015014
- Martin I, Miot S, Barbero A, Jakob M, Wendt D. Osteochondral tissue engineering. J Biomech. 2007;40(4): 750-765. doi: 10.1016/j.jbiomech.2006.03.008
- Zhang X, Li Z, Yang P, et al. Polyphenol scaffolds in tissue engineering. Mater Horiz. 2021;8(1):145-167. doi: 10.1039/D0MH01317J
- Gao Q, Yu K, Chen F, Lu L, Zhang P. Investigation on the temperature distribution uniformity of an extrusion-based 3D print head and its temperature control strategy. Pharmaceutics. 2022;14(10):2108. doi: 10.3390/pharmaceutics14102108
- Yu K, Gao Q, Lu L, Zhang P. A process parameter design method for improving the filament diameter accuracy of extrusion 3D printing. Materials. 2022;15(7):2454. doi: 10.3390/ma15072454
- Galarraga JH, Kwon MY, Burdick JA. 3D bioprinting via an in situ crosslinking technique towards engineering cartilage tissue. Sci Rep. 2019;9(1):19987. doi: 10.1038/s41598-019-56117-3
- Choe R, Devoy E, Jabari E, Packer JD, Fisher JP. Biomechanical aspects of osteochondral regeneration: implications and strategies for three-dimensional bioprinting. Tissue Eng Part B Rev. 2022;28(4):766-788. doi: 10.1089/ten.teb.2021.0101
- Chen L, Deng C, Li J, et al. 3D printing of a lithium-calcium-silicate crystal bioscaffold with dual bioactivities for osteochondral interface reconstruction. Biomaterials. 2019;196:138-150. doi: 10.1016/j.biomaterials.2018.04.005
- Akizuki S, Mow VC, Muller F, Pita JC, Howell DS, Manicourt DH. Tensile properties of human knee joint cartilage: I. Influence of ionic conditions, weight bearing, and fibrillation on the tensile modulus. J Orthop Res. 1986; 4(4):379-392. doi: 10.1002/jor.1100040401
- Poole A, Kojima T, Yasuda T, Mwale F, Kobayashi M, Laverty S. Composition and structure of articular cartilage - a template for tissue repair. Clin Orthop Relat Res. 2001;(391 Suppl):S26-S33 doi: 10.1097/00003086-200110001-00004
- Sophia Fox AJ, Bedi A, Rodeo SA. The basic science of articular cartilage: structure, composition, and function. Sports Health. 2009;1(6):461-468. doi: 10.1177/1941738109350438
- Smith MR, Kawcak CE, McIlwraith CW. Science in brief: report on the Havemeyer Foundation workshop on subchondral bone problems in the equine athlete. Equine Vet J. 2016;48(1):6-8. doi: 10.1111/evj.12518
- Kazemi M, Williams JL. Properties of cartilage–subchondral bone junctions: a narrative review with specific focus on the growth plate. Cartilage. 2021;13(2_suppl): 16S-33S. doi: 10.1177/1947603520924776
- Chen Y, Hu Y, Yu YE, et al. Subchondral trabecular rod loss and plate thickening in the development of osteoarthritis. J Bone Miner Res. 2018;33(2):316-327. doi: 10.1002/jbmr.3313
- Yang PJ, Temenoff JS. Engineering orthopedic tissue interfaces. Tissue Eng Part B Rev. 2009; 15(2):127-141. doi: 10.1089/ten.teb.2008.0371
- Oegema T, Carpenter R, Hofmeister F, Thompson R. The interaction of the zone of calcified cartilage and subchondral bone in osteoarthritis. Microsc Res Tech. 1997;37(4):324-332. doi: 10.1002/(SICI)1097-0029(19970515)37:4<324::AID-JEMT7>3.0.CO;2-K
- Scotti C, Wirz D, Wolf F, et al. Engineering human cell-based, functionally integrated osteochondral grafts by biological bonding of engineered cartilage tissues to bony scaffolds. Biomaterials. 2010;31(8):2252-2259. doi: 10.1016/j.biomaterials.2009.11.110
- Mekhileri NV, Lim KS, Brown G, et al. Automated 3D bioassembly of micro-tissues for biofabrication of hybrid tissue engineered constructs. Biofabrication. 2018;10(2):024103. doi: 10.1088/1758-5090/aa9ef1
- Bittner SM, Smith BT, Diaz-Gomez L, et al. Fabrication and mechanical characterization of 3D printed vertical uniform and gradient scaffolds for bone and osteochondral tissue engineering. Acta Biomater. 2019;90:37-48. doi: 10.1016/j.actbio.2019.03.041
- Choe R, Devoy E, Kuzemchak B, et al. Computational investigation of interface printing patterns within 3D printed multilayered scaffolds for osteochondral tissue engineering. Biofabrication. 2022;14(2):10.1088/1758-5090/ac5220. doi: 10.1088/1758-5090/ac5220
- Zhang W, Lian Q, Li D, et al. The effect of interface microstructure on interfacial shear strength for osteochondral scaffolds based on biomimetic design and 3D printing. Mater Sci Eng C. 2015;46:10-15. doi: 10.1016/j.msec.2014.09.042
- Schaefer D, Martin I, Jundt G, et al. Tissue‐engineered composites for the repair of large osteochondral defects. Arthritis Rheum. 2002;46(9):2524-2534. doi: 10.1002/art.10493
- Bertassoni LE, Coelho PG. Preface: engineering mineralized and load-bearing tissues: progress and challenges. Adv Exp Med Biol. 2015;881:v-vii. doi: 10.1007/978-3-319-22345-2
- Zhou L, GJVM VO, Malda J, et al. Innovative tissue‐engineered strategies for osteochondral defect repair and regeneration: current progress and challenges. Adv Healthc Mater. 2020;9(23):e2001008. doi: 10.1002/adhm.202001008
- Richbourg NR, Wancura M, Gilchrist AE, et al. Precise control of synthetic hydrogel network structure via linear, independent synthesis-swelling relationships. Sci Adv. 2021;7(7):eabe3245. doi: 10.1126/sciadv.abe3245
- Yang Z, Wu Y, Li C, et al. Improved mesenchymal stem cells attachment and in vitro cartilage tissue formation on chitosan-modified poly(L-lactide-co-epsilon-caprolactone) scaffold. Tissue Eng Part A. 2012;18(3-4):242-251. doi: 10.1089/ten.TEA.2011.0315
- Duan R, Wang Y, Zhang Y, et al. Blending with poly(l-lactic acid) improves the printability of poly(l-lactide-co-caprolactone) and enhances the potential application in cartilage tissue engineering. ACS Omega. 2021;6(28):18300-18313. doi: 10.1021/acsomega.1c02190
- Qiao Z, Zhang W, Jiang H, Li X, An W, Yang H. 3D-printed composite scaffold with anti-infection and osteogenesis potential against infected bone defects. RSC Adv. 2022;12(18):11008-11020. doi: 10.1039/d2ra00214k
- Sta Agueda JRH, Chen Q, Maalihan RD, et al. 3D printing of biomedically relevant polymer materials and biocompatibility. MRS Commun. 2021;11(2):197-212. doi: 10.1557/s43579-021-00038-8