3D-printed ellipsoid bionic porous titanium alloy scaffold for promotion of osseointegration
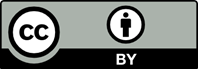
3D-printed porous bionic scaffolds are used to imitate irregular bone shapes and provide a suitable growth environment for integrated bone tissue. Although many bionic structures have emerged, it remains to be determined which structure has the best osseointegration ability. In this study, we found that the trabecular bone structure is ellipsoid-like by scanning human bone specimens. It is speculated that the bionic scaffold produced using the ellipsoid intersection model has better osseointegration properties. Subsequently, computer-aided design was used to contrive porous scaffolds with pores of three different geometric shapes, including tetrahedron (TBC), Schwarz-P (P), and ellipsoid bionic (EB) structures. The scaffolds were prepared by selective laser melting with similar porosities (65%) and pore sizes (480 μm). Mechanical tests have proven the scaffolds to have high accuracy and good mechanical properties. Subsequently, three scaffolds were implanted into the lateral condyle femur of rabbits. Micro-computed tomography quantitative analysis and hard tissue section staining were performed on samples harvested 6 and 12 weeks after surgery. The results demonstrated the advantage of EB structure in bone ingrowth, consistent with the results of the in vitro cell experiments. According to computer fluid dynamics simulations, the EB structure has the most appropriate internal streamline structure and the best wall shear stress distribution for cell proliferation, demonstrating its benefits in osseointegration. The pore geometry has a significant effect on the osseointegration of the scaffold. The EB structure, proposed for the first time in this study, demonstrates significantly superior osseointegration compared to the regular TBC structure and P-curved surface structure, thereby providing a reference for future research on optimal 3D-printed bionic porous scaffolds.

- Schmitz JP, Hollinger JO. The critical size defect as an experimental model for craniomandibulofacial nonunions. Clin Orthop Relat Res. 1986;205:299-308.
- Maietta S, Gloria A, Improta G, Richetta M, De Santis R, Martorelli M. A further analysis on Ti6Al4V lattice structures manufactured by selective laser melting. J Healthc Eng. 2019;2019:3212594. doi: 10.1155/2019/3212594
- Chen Z, Yan X, Yin S, et al. Influence of the pore size and porosity of selective laser melted Ti6Al4V ELI porous scaffold on cell proliferation, osteogenesis and bone ingrowth. Mater Sci Eng C Mater Biol Appl. 2020;106:110289. doi: 10.1016/j.msec.2019.110289
- Pei B, Hu M, Wu X, et al. Investigations into the effects of scaffold microstructure on slow-release system with bioactive factors for bone repair. Front Bioeng Biotechnol. 2023;11:1230682. doi: 10.3389/fbioe.2023.1230682
- Hou C, Liu Y, Xu W, et al. Additive manufacturing of functionally graded porous titanium scaffolds for dental applications. Biomater Adv. 2022;139:213018. doi: 10.1016/j.bioadv.2022.213018
- Limongi T, Susa F, Allione M, di Fabrizio E. Drug delivery applications of three-dimensional printed (3DP) mesoporous scaffolds. Pharmaceutics. 2020;12(9):851. doi: 10.3390/pharmaceutics12090851
- George SM, Nayak C, Singh I, Balani K. Multifunctional hydroxyapatite composites for orthopedic applications: a review. ACS Biomater Sci Eng. 2022;8(8):3162-3186. doi: 10.1021/acsbiomaterials.2c00140
- Bidan CM, Kommareddy KP, Rumpler M, Kollmannsberger P, Fratzl P, Dunlop JW. Geometry as a factor for tissue growth: towards shape optimization of tissue engineering scaffolds. Adv Healthc Mater. 2013;21:186-194. doi: 10.1002/adhm.201200159
- Cho YS, Gwak SJ, Cho YS. Fabrication of polycaprolactone/ nano hydroxyapatite (PCL/nHA) 3D scaffold with enhanced in vitro cell response via design for additive manufacturing (DfAM). Polymers (Basel). 2021;13(9):1394.doi: 10.3390/polym13091394
- Kou XY, Tan ST. A simple and effective geometric representation for irregular porous structure modeling. Computer-Aided Design. 2010;42(10):930-941. doi: 10.1016/j.cad.2010.06.006
- Yoo DJ. Heterogeneous porous scaffold design for tissue engineering using triply periodic minimal surfaces. Int J Precis Eng Manuf. 2012;13(4):527-537. doi: 10.1007/s12541-012-0068-5
- Yang N, Quan Z, Zhang DW. Multi-morphology transition hybridization CAD design of minimal surface porous structures for use in tissue engineering. Computer-Aided Design. 2014;56:11-21. doi: 10.1016/j.cad.2014.06.006
- Li T, Ma Z, Zhang Y, et al. Regeneration of humeral head using a 3D bioprinted anisotropic scaffold with dual modulation of endochondral ossification. Adv Sci (Weinh). 2023;1012:e2205059. doi: 10.1002/advs.202205059
- Kong DY, Wang Q, Huang JG. A biomimetic structural material with adjustable mechanical property for bone tissue engineering. Adv Funct Mater. 2023;33(50):2305412. doi: 10.1002/adfm.202305412
- Hosokawa A. Effect of porosity distribution in the propagation direction on ultrasound waves through cancellous bone. IEEE Trans Ultrason Ferroelectr Freq Control. 2010;57(6):1320-1328. doi: 10.1109/TUFFC.2010.1552
- Chen YT, Chuang YH, Chen CM, Wang JY, Wang J. Development of hybrid scaffolds with biodegradable polymer composites and bioactive hydrogels for bone tissue engineering. Biomater Adv. 2023;153:213562. doi: 10.1016/j.bioadv.2023.213562
- Li J, Cui X, Hooper GJ, Lim KS, Woodfield TBF. Rational design, bio-functionalization and biological performance of hybrid additive manufactured titanium implants for orthopaedic applications: a review. J Mech Behav Biomed Mater. 2020;105:103671. doi: 10.1016/j.jmbbm.2020.103671
- Mohammadi H, Sepantafar M, Muhamad N. How does scaffold porosity conduct bone tissue regeneration? Adv Eng Mater. 2021;23(10):2100463. doi: 10.1002/adem.202100463
- Entezari A, Roohani I, Li G, et al. Architectural design of 3D printed scaffolds controls the volume and functionality of newly formed bone. Adv Healthc Mater. 2019;81:e1801353. doi: 10.1002/adhm.201801353
- Roohaniesfahani I, Wang J, No YJ, et al. Modulatory effect of simultaneously released magnesium, strontium, and silicon ions on injectable silk hydrogels for bone regeneration. Mater Sci Eng C Mater Biol Appl. 2019;94:976-987. doi: 10.1016/j.msec.2018.10.053
- Gentleman E, Fredholm YC, Jell G, et al. The effects of strontium-substituted bioactive glasses on osteoblasts and osteoclasts in vitro. Biomaterials. 2010;3114:3949-3956. doi: 10.1016/j.biomaterials.2010.01.121
- Azarkina K, Gromova E, Malashicheva A. “A Friend Among Strangers” or the ambiguous roles of Runx2. Biomolecules. 2024;14(11):1392. doi: 10.3390/biom14111392
- Karsenty G. Osteocalcin: a multifaceted bone-derived hormone. Annu Rev Nutr. 2023;43:55-71. doi: 10.1146/annurev-nutr-061121-091348
- Zhao Y, Huang Z, Gao L, Ma H, Chang R. Osteopontin/ SPP1: a potential mediator between immune cells and vascular calcification. Front Immunol. 2024;15:1395596. doi: 10.3389/fimmu.2024.1395596
- Gomez S, Vlad MD, Lopez J, Fernandez E. Design and properties of 3D scaffolds for bone tissue engineering. Acta Biomater. 2016;42:341-350. doi: 10.1016/j.actbio.2016.06.032
- Vossenberg P, Higuera GA, van Straten G, van Blitterswijk CA, van Boxtel AJ. Darcian permeability constant as indicator for shear stresses in regular scaffold systems for tissue engineering. Biomech Model Mechanobiol. 2009;86:499-507. doi: 10.1007/s10237-009-0153-6
- Lewis G. Properties of open-cell porous metals and alloys for orthopaedic applications. J Mater Sci Mater Med. 2013;24(10):2293-2325. doi: 10.1007/s10856-013-4998-y
- Li L, Shi J, Zhang K, et al. Early osteointegration evaluation of porous Ti6Al4V scaffolds designed based on triply periodic minimal surface models. J Orthop Translat. 2019; 19:94-105. doi: 10.1016/j.jot.2019.03.003
- Beaudoin AJ, Mihalko WM, Krause WR. Finite element modelling of polymethylmethacrylate flow through cancellous bone. J Biomech. 1991;24(2):127-136. doi: 10.1016/0021-9290(91)90357-s
- Nauth A, Lee M, Gardner MJ, et al. Principles of nonunion management: state of the art. J Orthop Trauma. 2018;32(Suppl 1):S52-S57. doi: 10.1097/BOT.0000000000001122
- Huang EE, Zhang N, Shen H, et al. Novel techniques and future perspective for investigating critical-size bone defects. Bioengineering (Basel). 2022;9(4):171. doi: 10.3390/bioengineering9040171
- Papakostidis C, Bhandari M, Giannoudis PV. Distraction osteogenesis in the treatment of long bone defects of the lower limbs: effectiveness, complications and clinical results; a systematic review and meta-analysis. Bone Joint J. 2013;95(B12):1673-1680. doi: 10.1302/0301-620X.95B12.32385
- Fung B, Hoit G, Schemitsch E, Godbout C, Nauth A. The induced membrane technique for the management of long bone defects. Bone Joint J. 2020;102(B12):1723-1734. doi: 10.1302/0301-620X.102B12.BJJ-2020-1125.R1
- Niinomi M, Nakai M. Titanium-based biomaterials for preventing stress shielding between implant devices and bone. Int J Biomater. 2011;2011:836587. doi: 10.1155/2011/836587
- Song K, Wang Z, Lan J, Ma S. Porous structure design and mechanical behavior analysis based on TPMS for customized root analogue implant. J Mech Behav Biomed Mater. 2021;115:104222. doi: 10.1016/j.jmbbm.2020.104222
- Li X, Xiong ZY, Zhang H. Development of functionally graded porous titanium/silk fibroin composite scaffold for bone repair. Mater Lett. 2021;282:128670. doi: 10.1016/j.matlet.2020.128670
- Morais AR, Alencar Edo N, Xavier Junior FH, et al. Freeze-drying of emulsified systems: a review. Int J Pharm. 2016;503(1-2):102-114. doi: 10.1016/j.ijpharm.2016.02.047
- Tang MS, Abdul Kadir AZ, Ngadiman NH. Simulation analysis of different bone scaffold porous structures for fused deposition modelling fabrication process. IOP Conf Ser: Mater Sci Eng. 2020;788(1):012023. doi: 10.1088/1757-899X/788/1/012023
- Zadpoor AA. Bone tissue regeneration: the role of scaffold geometry. Biomater Sci. 2015;32:231-245. doi: 10.1039/C4BM00291A
- Limmahakhun S, Oloyede A, Sitthiseripratip K. Stiffness and strength tailoring of cobalt chromium graded cellular structures for stress-shielding reduction. Mater Design. 2017;114:633-641. doi: 10.1016/j.matdes.2016.11.090
- Di Luca A, Longoni A, Criscenti G. Toward mimicking the bone structure: design of novel hierarchical scaffolds with a tailored radial porosity gradient. Biofabrication. 2016;8(4):045007. doi: 10.1088/1758-5090/8/4/045007
- Shi Y, Shi J, Sun Y. A hierarchical 3D graft printed with nanoink for functional craniofacial bone restoration. Adv Funct Mater. 2023;33(40):2301099. doi: 10.1002/adfm.202301099
- Zhang M, Lin R, Wang X, et al. 3D printing of Haversian bone-mimicking scaffolds for multicellular delivery in bone regeneration. Sci Adv. 2020;612:eaaz6725. doi: 10.1126/sciadv.aaz6725
- Pobloth AM, Checa S, Razi H, et al. Mechanobiologically optimized 3D titanium-mesh scaffolds enhance bone regeneration in critical segmental defects in sheep. Sci Transl Med. 2018;10(423):eaam8828. doi: 10.1126/scitranslmed.aam8828
- Li JJ, Dunstan CR, Entezari A, et al. A novel bone substitute with high bioactivity, strength, and porosity for repairing large and load-bearing bone defects. Adv Healthc Mater. 2019;8(8):e1801298. doi: 10.1002/adhm.201801298
- Yang W, Han Q, Chen H, et al. Additive manufactured trabecular-like Ti-6Al-4V scaffolds for promoting bone regeneration. J Mater Sci Technol. 2024; 188:116-130. doi: 10.1016/j.jmst.2023.10.061