Composite bacterial cellulose–psoralen– polycaprolactone scaffold for enhanced bone regeneration and infection prevention in open bone defects
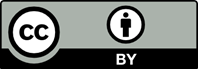
This study aimed to improve the treatment of open bone defects by developing a hybrid scaffold that enhances bone regeneration and prevents infections. Polycaprolactone (PCL) scaffolds were incorporated for mechanical support, while bacterial cellulose (BC) membranes facilitated psoralen (Pso) delivery. PCL scaffolds were fabricated via three-dimensional (3D) printing, and BC was extracted from fungi to prepare three types of scaffolds as follows: PCL, BC–PCL, and BC–Pso–PCL. The scaffolds were characterized through scanning electron microscopy, contact angle measurement, and infrared spectroscopy. Biocompatibility was assessed by cytotoxicity (CCK-8) assay, cell migration assay, live/dead cell staining, and cell proliferation experiments. Antibacterial effects were tested under a simulated bacterial environment. Osteogenic performance was evaluated by alkaline phosphatase (ALP) activity, Alizarin red staining (ARS), and immunofluorescence after osteogenic induction. Quantitative reverse transcriptase PCR (qRT-PCR) and Western blotting were performed to analyze bone regeneration and angiogenesis markers. Bone regeneration efficacy was assessed in vivo using a 5 mm critical-sized cranial bone defect model in rats. Biocompatibility studies demonstrated that all three scaffolds showed good biocompatibility. BC–Pso–PCL scaffold effectively inhibited Staphylococcus aureus growth. The ALP staining and ARS following osteogenic induction indicated that the BC–Pso–PCL group exhibited superior ALP activity and mineralized nodule formation compared to other groups. Subsequent immunofluorescence, qRT-PCR, and Western blot further confirmed the superior osteogenic performance of the BC–Pso–PCL scaffold. In vivo experiments demonstrated that the BC–Pso–PCL scaffold achieved the highest level of new bone formation in rat cranial defects. In conclusion, the BC–Pso–PCL scaffold demonstrated superior biocompatibility in cytotoxicity, cell migration, live/dead staining, and proliferation assays, while also promoting bone regeneration and angiogenesis. The in vivo study further confirmed its superior potential in bone formation. These findings highlight the BC–Pso– PCL hybrid scaffold as a promising implantable scaffold for treating open bone defects, with potential for future clinical applications.

- Wang J, Zhang Y, Tang Q, Zhang Y, Yin Y, Chen L. Application of antioxidant compounds in bone defect repair. Antioxidants (Basel). 2024;13(7):789. doi: 10.3390/antiox13070789
- Masters EA, Ricciardi BF, Bentley KLM, Moriarty TF, Schwarz EM, Muthukrishnan G. Skeletal infections: microbial pathogenesis, immunity and clinical management. Nat Rev Microbiol. 2022;20(7):385-400. doi: 10.1038/s41579-022-00686-0
- Lázár V, Snitser O, Barkan D, Kishony R. Antibiotic combinations reduce Staphylococcus aureus clearance. Nature. 2022;610(7932):540-546. doi: 10.1038/s41586-022-05260-5
- He Y, Liu X, Lei J, et al. Bioactive VS4-based sonosensitizer for robust chemodynamic, sonodynamic and osteogenic therapy of infected bone defects. J Nanobiotechnology. 2024;22(1):31. doi: 10.1186/s12951-023-02283-6
- Ma L, Cheng Y, Feng X, et al. A Janus-ROS healing system promoting infectious bone regeneration via sono-epigenetic modulation. Adv Mater. 2024;36(2): e2307846. doi: 10.1002/adma.202307846
- Yuan X, Zhu W, Yang Z, et al. Recent advances in 3D printing of smart scaffolds for bone tissue engineering and regeneration. Adv Mater. 2024;36(34):e2403641. doi: 10.1002/adma.202403641
- Wang J, Wu Y, Li G, et al. Engineering large-scale self-mineralizing bone organoids with bone matrix-inspired hydroxyapatite hybrid bioinks. Adv Mater. 2024;36(30):e2309875. doi: 10.1002/adma.202309875
- Lee H, Han G, Na Y, et al. 3D-printed tissue-specific nanospike-based adhesive materials for time-regulated synergistic tumor therapy and tissue regeneration in vivo. Adv Funct Mater. 2024;34(48):2406237. doi: 10.1002/adfm.202406237
- Xue Y, Niu W, Wang M, Chen M, Guo Y, Lei B. Engineering a biodegradable multifunctional antibacterial bioactive nanosystem for enhancing tumor photothermo-chemotherapy and bone regeneration. ACS Nano. 2020;14(1):442-453. doi: 10.1021/acsnano.9b06145
- Fang L, Liu Z, Wang C, et al. Vascular restoration through local delivery of angiogenic factors stimulates bone regeneration in critical size defects. Bioact Mater. 2024;36:580-594. doi: 10.1016/j.bioactmat.2024.07.003
- Shim JH, Yoon MC, Jeong CM, et al. Efficacy of rhBMP-2 loaded PCL/PLGA/β-TCP guided bone regeneration membrane fabricated by 3D printing technology for reconstruction of calvaria defects in rabbit. Biomed Mater. 2014;9(6):065006. doi: 10.1088/1748-6041/9/6/065006
- Wang J, Tavakoli J, Tang Y. Bacterial cellulose production, properties and applications with different culture methods - a review. Carbohydr Polym. 2019;219:63-76. doi: 10.1016/j.carbpol.2019.05.008
- Ren Y, Song X, Tan L, et al. A review of the pharmacological properties of psoralen. Front Pharmacol. 2020;11: 571535. doi: 10.3389/fphar.2020.571535
- An J, Yang H, Zhang Q, et al. Natural products for treatment of osteoporosis: the effects and mechanisms on promoting osteoblast-mediated bone formation. Life Sci. 2016;147:46-58. doi: 10.1016/j.lfs.2016.01.024
- Zhang T, Han W, Zhao K, et al. Psoralen accelerates bone fracture healing by activating both osteoclasts and osteoblasts. FASEB J. 2019;33(4):5399-5410. doi: 10.1096/fj.201801797R
- Liu L, Zhang L, Cui ZX, Liu XY, Xu W, Yang XW. Transformation of psoralen and isopsoralen by human intestinal microbial in vitro, and the biological activities of its metabolites. Molecules. 2019;24(22):4080. doi: 10.3390/molecules24224080
- Thakur A, Sharma R, Jaswal VS, Nepovimova E, Chaudhary A, Kuca K. Psoralen: a biologically important coumarin with emerging applications. Mini Rev Med Chem. 2020;20(18):1838-1845. doi: 10.2174/1389557520666200429101053
- Galiatsatos P, Maydan DD, Macalpine E, et al. Psoralen: a narrative review of current and future therapeutic uses. J Cancer Res Clin Oncol. 2024;150(3):130. doi: 10.1007/s00432-024-05648-y
- Huang K, Wu B, Hou Z, et al. Psoralen downregulates osteoarthritis chondrocyte inflammation via an estrogen-like effect and attenuates osteoarthritis. Aging (Albany NY). 2022;14(16):6716-6726. doi: 10.18632/aging.204245
- Yin BF, Li ZL, Yan ZQ, et al. Psoralen alleviates radiation-induced bone injury by rescuing skeletal stem cell stemness through AKT-mediated upregulation of GSK-3β and NRF2. Stem Cell Res Ther. 2022;13(1):241. doi: 10.1186/s13287-022-02911-2
- Huang Y, Liao L, Su H, et al. Psoralen accelerates osteogenic differentiation of human bone marrow mesenchymal stem cells by activating the TGF-β/Smad3 pathway. Exp Ther Med. 2021;22(3):940. doi: 10.3892/etm.2021.10372
- Huang K, Sun YQ, Chen XF, et al. Psoralen, a natural phytoestrogen, improves diaphyseal fracture healing in ovariectomized mice: a preliminary study. Exp Ther Med. 2021;21(4):368. doi: 10.3892/etm.2021.9799
- Li JP, Xie BP, Zhang WJ, et al. [Psoralen inhibits RAW264.7 differentiation into osteoclasts and bone resorption by regulating CD4+T cell differentiation]. Zhongguo Zhong Yao Za Zhi. 2018;43(6):1228-1234. doi: 10.19540/j.cnki.cjcmm.20180104.017
- Chai L, Zhou K, Wang S, et al. Psoralen and bakuchiol ameliorate M-CSF plus RANKL-induced osteoclast differentiation and bone resorption via inhibition of AKT and AP-1 pathways in vitro. Cell Physiol Biochem. 2018;48(5):2123-2133. doi: 10.1159/000492554
- Białczyk A, Wełniak A, Kamińska B, Czajkowski R. Oxidative stress and potential antioxidant therapies in vitiligo: a narrative review. Mol Diagn Ther. 2023;27(6):723-739. doi: 10.1007/s40291-023-00672-z
- Ma Q, Bian M, Gong G, et al. Synthesis and evaluation of bakuchiol derivatives as potent anti-inflammatory agents in vitro and in vivo. J Nat Prod. 2022;85(1):15-24. doi: 10.1021/acs.jnatprod.1c00377
- Guo Z, Li P, Wang C, et al. Five constituents contributed to the psoraleae fructus-induced hepatotoxicity via mitochondrial dysfunction and apoptosis. Front Pharmacol. 2021;12:682823. doi: 10.3389/fphar.2021.682823
- Zhang R, Shi W, Li L, Huang X, Xu D, Wu L. Biological activity and health promoting effects of psoralidin. Pharmazie. 2019;74(2):67-72. doi: 10.1691/ph.2019.8619
- Chiou WF, Don MJ, Liao JF, Wei BL. Psoralidin inhibits LPS-induced iNOS expression via repressing Syk-mediated activation of PI3K-IKK-IκB signaling pathways. Eur J Pharmacol. 2011;650(1):102-109. doi: 10.1016/j.ejphar.2010.10.004
- Kong L, Ma R, Yang X, et al. Psoralidin suppresses osteoclastogenesis in BMMs and attenuates LPS-mediated osteolysis by inhibiting inflammatory cytokines. Int Immunopharmacol. 2017;51:31-39. doi: 10.1016/j.intimp.2017.07.003
- Wang X, Yang X, Xiao X, Li X, Chen C, Sun D. Biomimetic design of platelet-rich plasma controlled release bacterial cellulose/hydroxyapatite composite hydrogel for bone tissue engineering. Int J Biol Macromol. 2024;269(Pt 2):132124. doi: 10.1016/j.ijbiomac.2024.132124
- Rivero-Buceta V, Aguilar MR, Hernández-Arriaga AM, et al. Anti-staphylococcal hydrogels based on bacterial cellulose and the antimicrobial biopolyester poly(3- hydroxy-acetylthioalkanoate-co-3-hydroxyalkanoate). Int J Biol Macromol. 2020;162:1869-1879. doi: 10.1016/j.ijbiomac.2020.07.289
- Cao Z, Wang H, Chen J, et al. Silk-based hydrogel incorporated with metal-organic framework nanozymes for enhanced osteochondral regeneration. Bioact Mater. 2022;20:221-242. doi: 10.1016/j.bioactmat.2022.05.025
- Yuan S, Zheng B, Zheng K, et al. Immunoregulation in skull defect repair with a smart hydrogel loaded with mesoporous bioactive glasses. Biomater Res. 2024;28:0074. doi: 10.34133/bmr.0074
- Wasti S, Adhikari S. Use of biomaterials for 3d printing by fused deposition modeling technique: a review. Front Chem. 2020;8:315. doi: 10.3389/fchem.2020.00315
- Xie Y, Zheng Y, Fan J, Wang Y, Yue L, Zhang N. Novel electronic-ionic hybrid conductive composites for multifunctional flexible bioelectrode based on in situ synthesis of poly(dopamine) on bacterial cellulose. ACS Appl Mater Interfaces. 2018;10(26):22692-22702. doi: 10.1021/acsami.8b05345
- Nie R, Zhang QY, Feng ZY, et al. Hydrogel-based immunoregulation of macrophages for tissue repair and regeneration. Int J Biol Macromol. 2024;268(Pt 1):131643. doi: 10.1016/j.ijbiomac.2024.131643
- Duda GN, Geissler S, Checa S, Tsitsilonis S, Petersen A, Schmidt-Bleek K. The decisive early phase of bone regeneration. Nat Rev Rheumatol. 2023;19(2):78-95. doi: 10.1038/s41584-022-00887-0
- Yang N, Liu Y. The role of the immune microenvironment in bone regeneration. Int J Med Sci. 2021;18(16):3697-3707. doi: 10.7150/ijms.61080
- Riegger J, Schoppa A, Ruths L, Haffner-Luntzer M, Ignatius A. Oxidative stress as a key modulator of cell fate decision in osteoarthritis and osteoporosis: a narrative review. Cell Mol Biol Lett. 2023;28(1):76. doi: 10.1186/s11658-023-00489-y
- Iantomasi T, Romagnoli C, Palmini G, et al. Oxidative stress and inflammation in osteoporosis: molecular mechanisms involved and the relationship with microRNAs. Int J Mol Sci. 2023;24(4):3772. doi: 10.3390/ijms24043772
- Zaidi M, Yuen T, Kim SM. Pituitary crosstalk with bone, adipose tissue and brain. Nat Rev Endocrinol. 2023;19(12):708-721. doi: 10.1038/s41574-023-00894-5
- Wang T, Ye J, Zhang Y, et al. Role of oxytocin in bone. Front Endocrinol (Lausanne). 2024;15:1450007.
doi: 10.3389/fendo.2024.1450007
- Liu M, Banerjee R, Rossa C Jr, D’Silva NJ. RAP1-RAC1 signaling has an important role in adhesion and migration in HNSCC. J Dent Res. 2020;99(8):959-968. doi: 10.1177/0022034520917058
- Peña OA, Martin P. Cellular and molecular mechanisms of skin wound healing. Nat Rev Mol Cell Biol. 2024;25(8):599-616. doi: 10.1038/s41580-024-00715-1
- Wang T, Ouyang H, Luo Y, et al. Rehabilitation exercise-driven symbiotic electrical stimulation system accelerating bone regeneration. Sci Adv. 2024;10(1):eadi6799. doi: 10.1126/sciadv.adi6799
- Sun H, Xu J, Wang Y, et al. Bone microenvironment regulative hydrogels with ROS scavenging and prolonged oxygen-generating for enhancing bone repair. Bioact Mater. 2023;24:477-496. doi: 10.1016/j.bioactmat.2022.12.021
- Gao Z, Song Z, Guo R, et al. Mn single-atom nanozyme functionalized 3D-printed bioceramic scaffolds for enhanced antibacterial activity and bone regeneration. Adv Healthc Mater. 2024;13(13):e2303182. doi: 10.1002/adhm.202303182
- Zhang Q, Jing Y, Gong Q, et al. Endorepellin downregulation promotes angiogenesis after experimental traumatic brain injury. Neural Regen Res. 2024;19(5):1092-1097. doi: 10.4103/1673-5374.382861
- Lin J, Shi Y, Men Y, Wang X, Ye J, Zhang C. Mechanical roles in formation of oriented collagen fibers. Tissue Eng Part B Rev. 2020;26(2):116-128. doi: 10.1089/ten.TEB.2019.0243
- Wang H, Hsu YC, Wang C, et al. Conductive and enhanced mechanical strength of Mo2Ti2C3 MXene-based hydrogel promotes neurogenesis and bone regeneration in bone defect repair. ACS Appl Mater Interfaces. 2024;16(14):17208-17218. doi: 10.1021/acsami.3c19410
- Zhao Z, Xia X, Liu J, et al. Cartilage-inspired self-assembly glycopeptide hydrogels for cartilage regeneration via ROS scavenging. Bioact Mater. 2023;32:319-332. doi: 10.1016/j.bioactmat.2023.10.013
- Shaw P, Dwivedi SKD, Bhattacharya R, Mukherjee P, Rao G. VEGF signaling: role in angiogenesis and beyond. Biochim Biophys Acta Rev Cancer. 2024;1879(2):189079. doi: 10.1016/j.bbcan.2024.189079
- Portais A, Gallouche M, Pavese P, et al. Staphylococcus aureus screening and preoperative decolonisation with Mupirocin and Chlorhexidine to reduce the risk of surgical site infections in orthopaedic surgery: a pre-post study. Antimicrob Resist Infect Control. 2024;13(1):75. doi: 10.1186/s13756-024-01432-2
- Cheung GYC, Bae JS, Otto M. Pathogenicity and virulence of Staphylococcus aureus. Virulence. 2021;12(1):547-569. doi: 10.1080/21505594.2021.1878688