Enhancing bone ingrowth and mechanical bonding in 3D-printed titanium alloy implants via lattice design and growth factors
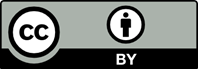
Patient-specific three-dimensional (3D)-printed titanium alloy implants often face challenges such as stress shielding and inadequate osseointegration. This study investigated the effects of lattice designs and growth factor-enriched sticky bone on bone ingrowth and mechanical bond strength at the implant–bone interface of large-scale bone defects at the distal femur condyle in a New Zealand rabbit model. Hollow cylindrical implants (length: 12 mm; diameter: 6 mm [outer], 2 mm [inner]) with diamond (DU) and randomly deformed spherical (YMR) lattices were designed and implanted into rabbit femoral condyles. Platelet-rich fibrin (PRF) was prepared using a novel negative pressure centrifuge and mixed with synthetic bone graft material to form sticky bone, which was used to fill the implant cavities. Micro-computed tomography (CT) imaging assessed bone ingrowth volume across zones, while mechanical testing evaluated shear bond strength. The results demonstrated that growth factors are the primary driver of bone ingrowth and mechanical strength. Bone growth volume increased significantly in zone A (implant cavity), with sticky bone yielding a 6.9-fold increase for DU (6.66 mm³ vs. 45.89 mm³) and a 3.5-fold increase for YMR (14.68 mm³ vs. 51.95 mm³). Across zones B and C (lattice layers), YMR lattices consistently outperformed DU in promoting bone growth and stability. Push-out tests demonstrated shear bond strengths of 2.78 MPa for DU and 2.83 MPa for YMR with growth factors, compared to 1.71 MPa for controls. This study highlights the critical role of growth factors in enhancing bone integration and demonstrates the complementary benefits of optimized lattice designs, particularly YMR, in improving osseointegration and mechanical stability. The findings provide a promising strategy for using 3D-printed titanium alloy implants with sticky bone systems to address large bone defects in clinical applications.

- Aung L, Tin AS, Quah TC, Pho RW. Osteogenic sarcoma in children and young adults. Ann Acad Med Singap. 2014;43(6):305-313.
- Wu PK, Chen CF, Chen CM, et al. Intraoperative extracorporeal irradiation and frozen treatment on tumor-bearing autografts show equivalent outcomes for biologic reconstruction. Clin Orthop Relat Res. 2018;476(4):877-889. doi: 10.1007/s11999.0000000000000022
- Li CH, Wu CH, Lin CL. Design of a patient-specific mandible reconstruction implant with dental prosthesis for metal 3D printing using integrated weighted topology optimization and finite element analysis. J Mech Behav Biomed Mater. 2020;105:103700. doi: 10.1016/j.jmbbm.2020.103700
- Omar O, Engstrand T, Kihlstrom Burenstam Linder L, et al. In situ bone regeneration of large cranial defects using synthetic ceramic implants with a tailored composition and design. Proc Natl Acad Sci U S A. 2020;117(43): 26660-26671. doi: 10.1073/pnas.2007635117
- Wu PK, Lee CW, Sun WH, Lin CL. Biomechanical analysis and design method for patient-specific reconstructive implants for large bone defects of the distal lateral femur. Biosensors (Basel). 2021;12(1):4. doi: 10.3390/bios12010004
- Wong KW, Wu CD, Chien C-S, Lee C-W, Yang T-H, Lin C-L. Patient-specific 3-dimensional printing titanium implant biomechanical evaluation for complex distal femoral open fracture reconstruction with segmental large bone defect: a nonlinear finite element analysis. Appl Sci. 2020; 10(12):4098. doi: 10.3390/app10124098
- Meng M, Wang J, Huang H, Liu X, Zhang J, Li Z. 3D printing metal implants in orthopedic surgery: methods, applications and future prospects. J Orthop Translat. 2023;42:94-112. doi: 10.1016/j.jot.2023.08.004
- Park JW, Seo E, Park H, et al. Hybrid solid mesh structure for electron beam melting customized implant to treat bone cancer. Int J Bioprint. 2023;9(4):716. doi: 10.18063/ijb.716
- Wu Y, Liu J, Kang L, et al. An overview of 3D printed metal implants in orthopedic applications: Present and future perspectives. Heliyon. 2023;9(7):e17718. doi: 10.1016/j.heliyon.2023.e17718
- Marin E, Lanzutti A. Biomedical applications of titanium alloys: a comprehensive review. Materials (Basel). 2023;17(1):114. doi: 10.3390/ma17010114
- Wu N, Li S, Zhang B, et al. The advances of topology optimization techniques in orthopedic implants: a review. Med Biol Eng Comput. 2021;59(9):1673-1689. doi: 10.1007/s11517-021-02361-7
- Al-Barqawi MO, Church B, Thevamaran M, Thoma DJ, Rahman A. Design and validation of additively manufactured metallic cellular scaffold structures for bone tissue engineering. Materials (Basel). 2022;15(9):3310. doi: 10.3390/ma15093310
- Al-Barqawi MO, Church B, Thevamaran M, Thoma DJ, Rahman A. Experimental validation and evaluation of the bending properties of additively manufactured metallic cellular scaffold structures for bone tissue engineering. Materials (Basel). 2022;15(10):3447. doi: 10.3390/ma15103447
- Smit T, Koppen S, Ferguson SJ, Helgason B. Conceptual design of compliant bone scaffolds by full-scale topology optimization. J Mech Behav Biomed Mater. 2023; 143:105886. doi: 10.1016/j.jmbbm.2023.105886
- Luo W, Wang Y, Wang Z, et al. Advanced topology of triply periodic minimal surface structure for osteogenic improvement within orthopedic metallic screw. Mater Today Bio. 2024;27:101118. doi: 10.1016/j.mtbio.2024.101118
- Van Bael S, Chai YC, Truscello S, et al. The effect of pore geometry on the in vitro biological behavior of human periosteum-derived cells seeded on selective laser-melted Ti6Al4V bone scaffolds. Acta Biomater. 2012; 8(7):2824-2834. doi: 10.1016/j.actbio.2012.04.001
- Wang H, Su K, Su L, Liang P, Ji P, Wang C. The effect of 3D-printed Ti(6)Al(4)V scaffolds with various macropore structures on osteointegration and osteogenesis: a biomechanical evaluation. J Mech Behav Biomed Mater. 2018;88:488-496. doi: 10.1016/j.jmbbm.2018.08.049
- Deering J, Grandfield K. Current interpretations on the in vivo response of bone to additively manufactured metallic porous scaffolds: a review. Biomater Biosyst. 2021; 2:100013. doi: 10.1016/j.bbiosy.2021.100013
- Kiselevskiy MV, Anisimova NY, Kapustin AV, et al. Development of bioactive scaffolds for orthopedic applications by designing additively manufactured titanium porous structures: a critical review. Biomimetics (Basel). 2023;8(7):546. doi: 10.3390/biomimetics8070546
- Bolukbasi N, Yeniyol S, Tekkesin MS, Altunatmaz K. The use of platelet-rich fibrin in combination with biphasic calcium phosphate in the treatment of bone defects: a histologic and histomorphometric study. Curr Ther Res Clin Exp. 2013;75:15-21. doi: 10.1016/j.curtheres.2013.05.002
- Masuki H, Okudera T, Watanebe T, et al. Growth factor and pro-inflammatory cytokine contents in platelet-rich plasma (PRP), plasma rich in growth factors (PRGF), advanced platelet-rich fibrin (A-PRF), and concentrated growth factors (CGF). Int J Implant Dent. 2016;2(1):19. doi: 10.1186/s40729-016-0052-4
- Agrawal AA. Evolution, current status and advances in application of platelet concentrate in periodontics and implantology. World J Clin Cases. 2017;5(5):159-171. doi: 10.12998/wjcc.v5.i5.159
- Miron RJ, Zucchelli G, Pikos MA, et al. Use of platelet-rich fibrin in regenerative dentistry: a systematic review. Clin Oral Investig. 2017;21(6):1913-1927. doi: 10.1007/s00784-017-2133-z
- Lee YK, Wadhwa P, Cai H, et al. Micro-CT and histomorphometric study of bone regeneration effect with autogenous tooth biomaterial enriched with platelet-rich fibrin in an animal model. Scanning. 2021; 2021:6656791. doi: 10.1155/2021/6656791
- Wong KW, Chen YS, Lin CL. Evaluation optimum ratio of synthetic bone graft material and platelet rich fibrin mixture in a metal 3D printed implant to enhance bone regeneration. J Orthop Surg Res. 2024;19(1):299. doi: 10.1186/s13018-024-04784-y
- Wang YT, Chang CM, Liu PS, Lin CL. Feasibility evaluation of a new lattice for porous surface design in additive manufacturing medical implants under interfacial tensile bonded testing. Additive Manuf. 2023;66:103455. doi: 10.1016/j.addma.2023.103455
- Geng H, Todd NM, Devlin-Mullin A, et al. A correlative imaging based methodology for accurate quantitative assessment of bone formation in additive manufactured implants. J Mater Sci Mater Med. 2016;27(6):112. doi: 10.1007/s10856-016-5721-6
- Palmquist A, Shah FA, Emanuelsson L, Omar O, Suska F. A technique for evaluating bone ingrowth into 3D printed, porous Ti6Al4V implants accurately using X-ray micro-computed tomography and histomorphometry. Micron. 2017;94:1-8. doi: 10.1016/j.micron.2016.11.009
- Salamanca E, Hsu CC, Huang HM, et al. Bone regeneration using a porcine bone substitute collagen composite in vitro and in vivo. Sci Rep. 2018;8(1):984. doi: 10.1038/s41598-018-19629-y
- Tsujino T, Takahashi A, Yamaguchi S, et al. Evidence for contamination of silica microparticles in advanced platelet-rich fibrin matrices prepared using silica-coated plastic tubes. Biomedicines. 2019;7(2):45. doi: 10.3390/biomedicines7020045
- Kusaka T, Nakayama M, Nakamura K, Ishimiya M, Furusawa E, Ogasawara K. Effect of silica particle size on macrophage inflammatory responses. PLoS One. 2014;9(3):e92634. doi: 10.1371/journal.pone.0092634
- Miron RJ, Chai J, Fujioka-Kobayashi M, Sculean A, Zhang Y. Evaluation of 24 protocols for the production of platelet-rich fibrin. BMC Oral Health. 2020;20(1):310. doi: 10.1186/s12903-020-01299-w
- Frosch S, Nusse V, Frosch KH, Lehmann W, Buchhorn G. Osseointegration of 3D porous and solid Ti-6Al-4V implants - narrow gap push-out testing and experimental setup considerations. J Mech Behav Biomed Mater. 2021;115:104282. doi: 10.1016/j.jmbbm.2020.104282
- Sun C, Dong E, Chen J, et al. The promotion of mechanical properties by bone ingrowth in additive-manufactured titanium scaffolds. J Funct Biomater. 2022;13(3):127. doi: 10.3390/jfb13030127
- Egle K, Salma I, Dubnika A. From blood to regenerative tissue: how autologous platelet-rich fibrin can be combined with other materials to ensure controlled drug and growth factor release. Int J Mol Sci. 2021;22(21):11553. doi: 10.3390/ijms222111553
- Son HJ, Lee MN, Kim Y, et al. Bone generation following repeated administration of recombinant bone morphogenetic protein 2. Tissue Eng Regen Med. 2021;18(1):155-164. doi: 10.1007/s13770-020-00290-4
- Hwang JW, Han YH. Novel bone morphogenetic protein (BMP)-2/4 consensus peptide (BCP) for the osteogenic differentiation of c2C12 cells. Curr Protein Pept Sci. 2023;24(7):610-619. doi: 10.2174/1389203724666230614112027
- Wang M, Fan J, Wang A, et al. Effect of local application of bone morphogenetic protein -2 on experimental tooth movement and biological remodeling in rats. Front Physiol. 2023;14:1111857. doi: 10.3389/fphys.2023.1111857
- Clark DP, Badea CT. Micro-CT of rodents: state-of-the-art and future perspectives. Phys Med. 2014;30(6):619-634. doi: 10.1016/j.ejmp.2014.05.011
- Buie HR, Bosma NA, Downey CM, Jirik FR, Boyd SK. Micro-CT evaluation of bone defects: applications to osteolytic bone metastases, bone cysts, and fracture. Med Eng Phys. 2013;35(11):1645-1650. doi: 10.1016/j.medengphy.2013.05.016
- Hutton BF, Thomas BA, Erlandsson K, et al. What approach to brain partial volume correction is best for PET/MRI? Nucl Instrum Methods Phys Res A: Accelerat Spectrom Detect Assoc Equip. 2013;702:29-33. doi: 10.1016/j.nima.2012.07.059
- Vandeweghe S, Coelho PG, Vanhove C, Wennerberg A, Jimbo R. Utilizing micro-computed tomography to evaluate bone structure surrounding dental implants: a comparison with histomorphometry. J Biomed Mater Res B Appl Biomater. 2013;101(7):1259-1266. doi: 10.1002/jbm.b.32938
- Gabler C, Zietz C, Bieck R, et al. Quantification of osseointegration of plasma-polymer coated titanium alloyed implants by means of microcomputed tomography versus histomorphometry. Biomed Res Int. 2015; 2015:103137. doi: 10.1155/2015/103137
- Li F, Li J, Xu G, Liu G, Kou H, Zhou L. Fabrication, pore structure and compressive behavior of anisotropic porous titanium for human trabecular bone implant applications. J Mech Behav Biomed Mater. 2015;46:104-114. doi: 10.1016/j.jmbbm.2015.02.023
- Chang B, Song W, Han T, et al. Influence of pore size of porous titanium fabricated by vacuum diffusion bonding of titanium meshes on cell penetration and bone ingrowth. Acta Biomater. 2016;33:311-321. doi: 10.1016/j.actbio.2016.01.022
- Taniguchi N, Fujibayashi S, Takemoto M, et al. Effect of pore size on bone ingrowth into porous titanium implants fabricated by additive manufacturing: an in vivo experiment. Mater Sci Eng C Mater Biol Appl. 2016;59:690-701. doi: 10.1016/j.msec.2015.10.069
- Alaña M, Cutolo A, Probst G, Ruiz de Galarreta S, Van Hooreweder B. Understanding elastic anisotropy in diamond-based lattice structures produced by laser powder bed fusion: Effect of manufacturing deviations. Mater Design. 2020;195:108971. doi: 10.1016/j.matdes.2020.108971