3D bioprinting for tendon–bone interface regeneration
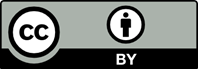
With the rapid advancement of three-dimensional (3D) bioprinting technology, its applications in tissue engineering and regenerative medicine have garnered increasing attention. Tendon–bone healing is a complex biological process, making injuries to the tendon–bone interface challenging to repair. Fortunately, 3D bioprinting provides numerous innovative solutions. Herein, we summarize the current state of 3D bioprinting technology in tendon–bone healing, exploring the latest developments in biomaterials, printing technologies, cell carriers, and preclinical research. In conclusion, the article discusses the current challenges faced in this field and outlines future research directions with careful consideration, providing valuable insights for ongoing investigations.

- Xiaorui L, Fuyin Z, Xudong W, et al. Biomaterial inks for extrusion-based 3D bioprinting: property, classification, modification, and selection. Int J Bioprint. 2023; 9(2):649. doi: 10.18063/ijb.v9i2.649
- Yu J, Park SA, Kim WD, et al. Current advances in 3D bioprinting technology and its applications for tissue engineering. Polymers. 2020;12(12):2958. doi: 10.3390/polym12122958
- Fang Y, Guo Y, Liu T, et al. Advances in 3D bioprinting. Chin J Mechl Eng Addit Manuf Front. 2022;1(1):100011. doi: 10.1016/j.cjmeam.2022.100011
- Timofticiuc IA, Dragosloveanu S, Caruntu A, et al. 3D bioprinting in limb salvage surgery. J Funct Biomater. 2024;15(12):383. doi: 10.3390/jfb15120383
- Marques CF, Diogo GS, Pina S, Oliveira JM, Silva TH, Reis RL. Collagen-based bioinks for hard tissue engineering applications: a comprehensive review. J Mater Sci Mater Med. 2019;30(3):32. doi: 10.1007/s10856-019-6234-x
- Zhang L, Zhang Q, Cui L, Wu L, Gao S. Kartogenin combined platelet-rich plasma (PRP) promoted tendon-bone healing for anterior cruciate ligament (ACL) reconstruction by suppressing inflammatory response via targeting AKT/PI3K/ NF-κB. Appl Biochem Biotechnol. 2023;195(2):1284-1296. doi: 10.1007/s12010-022-04178-y
- Chen Z, Jin M, He H, et al. Mesenchymal stem cells and macrophages and their interactions in tendon-bone healing. J Orthop Transl. 2023;39:63-73. doi: 10.1016/j.jot.2022.12.005
- Yang C, Teng Y, Geng B, et al. Strategies for promoting tendon-bone healing: current status and prospects. Front Bioeng Biotechnol. 2023;11:1118468. doi: 10.3389/fbioe.2023.1118468
- Chen X, Xue C, Li K, et al. Finite element analysis of anterior cruciate ligament reconstruction techniques: a comparison of the mechanical properties of all-inside fixation and traditional fixation. Front Bioeng Biotechnol. 2024;12:1438839. doi: 10.3389/fbioe.2024.1438839
- Chalmers PN, Mall NA, Moric M, et al. Does ACL reconstruction alter natural history? A systematic literature review of long-term outcomes. J Bone Joint Surg Am. 2014;96(4):292-300. doi: 10.2106/jbjs.L.01713
- Galatz LM, Ball CM, Teefey SA, Middleton WD, Yamaguchi K. The outcome and repair integrity of completely arthroscopically repaired large and massive rotator cuff tears. J Bone Joint Surg Am. 2004;86(2):219-224. doi: 10.2106/00004623-200402000-00002
- Maffulli N, Wong J, Almekinders LC. Types and epidemiology of tendinopathy. Clin Sports Med. 2003;22(4):675-692. doi: 10.1016/s0278-5919(03)00004-8
- Yang J, Kang Y, Zhao W, et al. Evaluation of patches for rotator cuff repair: a systematic review and meta-analysis based on animal studies. Bioact Mater. 2022;10:474-491. doi: 10.1016/j.bioactmat.2021.08.016
- Shen S, Lin Y, Sun J, Liu Y, Chen Y, Lu J. A new tissue engineering strategy to promote tendon-bone healing: regulation of osteogenic and chondrogenic differentiation of tendon-derived stem cells. Orthop Surg. 2024;16(10):2311-2325. doi: 10.1111/os.14152
- Moses B, Orchard J, Orchard J. Systematic review: annual incidence of ACL injury and surgery in various populations. Res Sports Med. 2012;20(3-4):157-179. doi: 10.1080/15438627.2012.680633
- von Porat A, Roos EM, Roos H. High prevalence of osteoarthritis 14 years after an anterior cruciate ligament tear in male soccer players: a study of radiographic and patient relevant outcomes. Ann Rheum Dis. 2004;63(3):269-273. doi: 10.1136/ard.2003.008136
- Albishi W, Baltow B, Albusayes N, Sayed AA, Alrabai HM. Hamstring autograft utilization in reconstructing anterior cruciate ligament: review of harvesting techniques, graft preparation, and different fixation methods. World J Orthop. 2022;13(10):876-890. doi: 10.5312/wjo.v13.i10.876
- Mironov V, Trusk T, Kasyanov V, Little S, Swaja R, Markwald R. Biofabrication: a 21st century manufacturing paradigm. Biofabrication. 2009;1(2):022001. doi: 10.1088/1758-5082/1/2/022001
- Meng M, Wang J, Huang H, Liu X, Zhang J, Li Z. 3D printing metal implants in orthopedic surgery: methods, applications and future prospects. J Orthop Translat. 2023;42:94-112. doi: 10.1016/j.jot.2023.08.004
- Joshua RJN, Raj SA, Hameed Sultan MT, et al. Powder bed fusion 3D printing in precision manufacturing for biomedical applications: a comprehensive review. Materials (Basel). 2024;17(3):769. doi: 10.3390/ma17030769
- Wang X, Xu S, Zhou S, et al. Topological design and additive manufacturing of porous metals for bone scaffolds and orthopaedic implants: a review. Biomaterials. 2016;83:127-141. doi: 10.1016/j.biomaterials.2016.01.012
- Tappa K, Jammalamadaka U, Weisman JA, et al. 3D printing custom bioactive and absorbable surgical screws, pins, and bone plates for localized drug delivery. J Funct Biomater. 2019;10(2):17. doi: 10.3390/jfb10020017
- Li X, Xie J, Lipner J, Yuan X, Thomopoulos S, Xia Y. Nanofiber scaffolds with gradations in mineral content for mimicking the tendon-to-bone insertion site. Nano Lett. 2009;9(7):2763-2768. doi: 10.1021/nl901582f
- Raja N, Park H, Gal CW, Sung A, Choi YJ, Yun HS. Support-less ceramic 3D printing of bioceramic structures using a hydrogel bath. Biofabrication. 2023;15(3). doi: 10.1088/1758-5090/acc903
- Park S, Kim JE, Han J, et al. 3D-printed poly(ε-caprolactone)/ hydroxyapatite scaffolds modified with alkaline hydrolysis enhance osteogenesis in vitro. Polymers. 2021;13(2):257. doi: 10.3390/polym13020257
- Zhao C, Wang X, Gao L, Jing L, Zhou Q, Chang J. The role of the micro-pattern and nano-topography of hydroxyapatite bioceramics on stimulating osteogenic differentiation of mesenchymal stem cells. Acta Biomater. 2018;73:509-521. doi: 10.1016/j.actbio.2018.04.030
- López-Valverde N, Flores-Fraile J, Ramírez JM, Sousa BM, Herrero-Hernández S, López-Valverde A. Bioactive surfaces vs. conventional surfaces in titanium dental implants: a comparative systematic review. J Clin Med. 2020;9(7):2047. doi: 10.3390/jcm9072047
- Shayeb MA, Elfadil S, Abutayyem H, et al. Bioactive surface modifications on dental implants: a systematic review and meta-analysis of osseointegration and longevity. Clin Oral Investig. 2024;28(11):592. doi: 10.1007/s00784-024-05958-y
- Acar K, Ersöz H. Comparison of three different surgical techniques in patients undergoing VATS and open thoracotomy. J Perianesth Nurs. 2022;37(4):479-484. doi: 10.1016/j.jopan.2021.10.007
- Liu W, Lipner J, Xie J, Manning CN, Thomopoulos S, Xia Y. Nanofiber scaffolds with gradients in mineral content for spatial control of osteogenesis. ACS Appl Mater Interfaces. 2014;6(4):2842-2849. doi: 10.1021/am405418g
- Zhou K, Yu P, Shi X, et al. Hierarchically porous hydroxyapatite hybrid scaffold incorporated with reduced graphene oxide for rapid bone ingrowth and repair. ACS Nano. 2019;13(8):9595-9606. doi: 10.1021/acsnano.9b04723
- Zhang Y, Yu T, Peng L, Sun Q, Wei Y, Han B. Advancements in hydrogel-based drug sustained release systems for bone tissue engineering. Front Pharmacol. 2020;11:622. doi: 10.3389/fphar.2020.00622
- Zhang CY, Fu CP, Li XY, et al. Three-dimensional bioprinting of decellularized extracellular matrix-based bioinks for tissue engineering. Molecules. 2022;27(11):3442. doi: 10.3390/molecules27113442
- Panda S, Hajra S, Mistewicz K, et al. A focused review on three-dimensional bioprinting technology for artificial organ fabrication. Biomater Sci. 2022;10(18):5054-5080. doi: 10.1039/d2bm00797e
- Zhao T, Liu Y, Wu Y, Zhao M, Zhao Y. Controllable and biocompatible 3D bioprinting technology for microorganisms: fundamental, environmental applications and challenges. Biotechnol Adv. 2023;69:108243. doi: 10.1016/j.biotechadv.2023.108243
- Ng WL, Shkolnikov V. Jetting-based bioprinting: process, dispense physics, and applications. Bio-Des Manuf. 2024;7(5):771-799. doi: 10.1007/s42242-024-00285-3
- Wang Y, Chen J, Yin Z, Li Y. A high-adaptability nozzle-array printing system based on a set covering printing planning model for printed display manufacturing. Sci Rep. 2023;13(1):156. doi: 10.1038/s41598-022-24135-3
- Chae S, Cho DW. Biomaterial-based 3D bioprinting strategy for orthopedic tissue engineering. Acta Biomater. 2023;156:4-20. doi: 10.1016/j.actbio.2022.08.004
- Sun LMP, To AC. Inexpensive DIY bioprinting in a secondary school setting. J Microbiol Biol Educ. 2023;24(2): e00124-22. doi: 10.1128/jmbe.00124-22
- Zhang YS, Haghiashtiani G, Hübscher T, et al. 3D extrusion bioprinting. Nat Rev Methods Primers. 2021;1(1):75. doi: 10.1038/s43586-021-00073-8
- Levato R, Dudaryeva O, Garciamendez-Mijares CE, et al. Light-based vat-polymerization bioprinting. Nat Rev Methods Primers. 2023;3(1):47. doi: 10.1038/s43586-023-00231-0
- Li Y, Zhang X, Zhang X, Zhang Y, Hou D. Recent progress of the vat photopolymerization technique in tissue engineering: a brief review of mechanisms, methods, materials, and applications. Polymers (Basel). 2023;15(19):3940. doi: 10.3390/polym15193940
- Nagakura R, Yamamoto M, Jeong J, et al. Switching of Sox9 expression during musculoskeletal system development. Sci Rep. 2020;10(1):8425. doi: 10.1038/s41598-020-65339-9
- Yoshimoto Y, Oishi Y. Mechanisms of skeletal muscle-tendon development and regeneration/healing as potential therapeutic targets. Pharmacol Ther. 2023;243:108357. doi: 10.1016/j.pharmthera.2023.108357
- Matson A, Konow N, Miller S, Konow PP, Roberts TJ. Tendon material properties vary and are interdependent among turkey hindlimb muscles. J Exp Biol. 2012; 215(Pt 20):3552-3558. doi: 10.1242/jeb.072728
- Ma H, Yang C, Ma Z, et al. Multiscale hierarchical architecture-based bioactive scaffolds for versatile tissue engineering. Adv Healthc Mater. 2022;11(13): e2102837. doi: 10.1002/adhm.202102837
- Kuznetsov S, Pankow M, Peters K, Huang HS. A structural-based computational model of tendon-bone insertion tissues. Math Biosci. 2020;327:108411. doi: 10.1016/j.mbs.2020.108411
- Pedaprolu K, Szczesny SE. Mouse Achilles tendons exhibit collagen disorganization but minimal collagen denaturation during cyclic loading to failure. J Biomech. 2023;151:111545. doi: 10.1016/j.jbiomech.2023.111545
- Yang S, Shi X, Li X, Wang J, Wang Y, Luo Y. Oriented collagen fiber membranes formed through counter-rotating extrusion and their application in tendon regeneration. Biomaterials. 2019;207:61-75. doi: 10.1016/j.biomaterials.2019.03.041
- Astill BD, Katsma MS, Cauthon DJ, et al. Sex-based difference in Achilles peritendinous levels of matrix metalloproteinases and growth factors after acute resistance exercise. J Appl Physiol (1985). 2017;122(2):361-367. doi: 10.1152/japplphysiol.00878.2016
- Basso O, Amis AA, Race A, Johnson DP. Patellar tendon fiber strains: their differential responses to quadriceps tension. Clin Orthop Relat Res. 2002;(400):246-253. doi: 10.1097/00003086-200207000-00030
- Samiric T, Parkinson J, Ilic MZ, Cook J, Feller JA, Handley CJ. Changes in the composition of the extracellular matrix in patellar tendinopathy. Matrix Biol. 2009; 28(4):230-236. doi: 10.1016/j.matbio.2009.04.001
- Attia M, Scott A, Carpentier G, et al. Greater glycosaminoglycan content in human patellar tendon biopsies is associated with more pain and a lower VISA score. Br J Sports Med. 2014;48(6):469-475. doi: 10.1136/bjsports-2013-092633
- Danielson P, Alfredson H, Forsgren S. Immunohistochemical and histochemical findings favoring the occurrence of autocrine/paracrine as well as nerve-related cholinergic effects in chronic painful patellar tendon tendinosis. Microsc Res Tech. 2006;69(10):808-819. doi: 10.1002/jemt.20351
- Das S, Sulaiman IM, Hussan F, Latiff AA, Suhaimi FH, Othman F. The additional tendons of the extensor digitorum muscle of the hand: an anatomical study with a clinical significance. Bratisl Lek Listy. 2008;109(12):584-586.
- Sbernardori MC, Pirino A, Fabbriciani C, Montella A. Localization of collagen type IV, fibronectin and elastin in the flexor digitorum tendons and in the perichondrium during prenatal development of the human hand. Ital J Anat Embryol. 2001;106(3):205-213.
- Ma L, Xu Y, Xu X, Pan Q, Xu Y. Application of biomimetic double-layer biofilm stent in arthroscopic rotator cuff repair: a protocol of randomized controlled trial. Medicine (Baltimore). 2021;100(1):e23960. doi: 10.1097/md.0000000000023960
- Li X, Shen P, Su W, Zhao S, Zhao J. Into-tunnel repair versus onto-surface repair for rotator cuff tears in a rabbit model. Am J Sports Med. 2018;46(7):1711-1719. doi: 10.1177/0363546518764685
- Chang CH, Chen CH, Liu HW, et al. Bioengineered periosteal progenitor cell sheets to enhance tendon-bone healing in a bone tunnel. Biomed J. 2012;35(6):473-480. doi: 10.4103/2319-4170.104412
- Xu Z, Xu W, Zhang T, Luo L. Mechanisms of tendon-bone interface healing: biomechanics, cell mechanics, and tissue engineering approaches. J Orthop Surg Res. 2024;19(1):817. doi: 10.1186/s13018-024-05304-8
- Dong Y, Li J, Jiang Q, et al. Structure, ingredient, and function-based biomimetic scaffolds for accelerated healing of tendon-bone interface. J Orthop Translat. 2024;48:70-88. doi: 10.1016/j.jot.2024.07.007
- Han F, Zhang P, Chen T, Lin C, Wen X, Zhao P. A LbL-assembled bioactive coating modified nanofibrous membrane for rapid tendon-bone healing in ACL reconstruction. Int J Nanomed. 2019;14:9159-9172. doi: 10.2147/ijn.S214359
- Bülow A, Schäfer B, Beier JP. Three-dimensional bioprinting in soft tissue engineering for plastic and reconstructive surgery. Bioengineering (Basel). 2023;10(10):1232. doi: 10.3390/bioengineering10101232
- Huang Y, He B, Wang L, et al. Bone marrow mesenchymal stem cell-derived exosomes promote rotator cuff tendon-bone healing by promoting angiogenesis and regulating M1 macrophages in rats. Stem Cell Res Ther. 2020;11(1):496. doi: 10.1186/s13287-020-02005-x
- Wang L, Guan C, Zhang T, et al. Comparative effect of skeletal stem cells versus bone marrow mesenchymal stem cells on rotator cuff tendon-bone healing. J Orthop Transl. 2024;47:87-96. doi: 10.1016/j.jot.2024.05.005
- Fu S, Lan Y, Wang G, et al. External stimulation: a potential therapeutic strategy for tendon-bone healing. Front Bioeng Biotechnol. 2023;11:1150290. doi: 10.3389/fbioe.2023.1150290
- Wong CC, Yeh YY, Yang TL, Tsuang YH, Chen CH. Augmentation of tendon graft-bone tunnel interface healing by use of bioactive platelet-rich fibrin scaffolds. Am J Sports Med. 2020;48(6):1379-1388. doi: 10.1177/0363546520908849
- Zhao X, Wu G, Zhang J, Yu Z, Wang J. Activation of CGRP receptor-mediated signaling promotes tendon-bone healing. Sci Adv. 2024;10(10):eadg7380. doi: 10.1126/sciadv.adg7380
- Bian X, Liu X, Zhou M, et al. Mechanical stimulation promotes fibrochondrocyte proliferation by activating the TRPV4 signaling pathway during tendon-bone insertion healing: CCN2 plays an important regulatory role. Burns Trauma. 2024;12:tkae028. doi: 10.1093/burnst/tkae028
- Lu H, Chen C, Qu J, et al. Initiation timing of low-intensity pulsed ultrasound stimulation for tendon-bone healing in a rabbit model. Am J Sports Med. 2016;44(10):2706-2715. doi: 10.1177/0363546516651863
- Li S, Xu Z, Wang Z, Xiang J, Zhang T, Lu H. Acceleration of bone-tendon interface healing by low-intensity pulsed ultrasound is mediated by macrophages. Phys Ther. 2021;101(7):pzab055. doi: 10.1093/ptj/pzab055
- Huegel J, Chan PYW, Weiss SN, et al. Pulsed electromagnetic field therapy alters early healing in a rat model of rotator cuff injury and repair: Potential mechanisms. J Orthop Res. 2022;40(7):1593-1603. doi: 10.1002/jor.25185
- Nakazawa K, Toyoda H, Manaka T, et al. Non-thermal atmospheric pressure gas discharge plasma enhances tendon-to-bone junction repair in a rabbit model. J Shoulder Elbow Surg. 2024: S1058-2746(24)00640-2. doi: 10.1016/j.jse.2024.07.039
- Longo UG, Risi Ambrogioni L, Berton A, et al. Physical therapy and precision rehabilitation in shoulder rotator cuff disease. Int Orthop. 2020;44(5):893-903. doi: 10.1007/s00264-020-04511-2
- Schindler OS. Surgery for anterior cruciate ligament deficiency: a historical perspective. Knee Surg Sports Traumatol Arthrosc. 2012;20(1):5-47. doi: 10.1007/s00167-011-1756-x
- Suchenski M, McCarthy MB, Chowaniec D, et al. Material properties and composition of soft-tissue fixation. Arthroscopy. 2010;26(6):821-831. doi: 10.1016/j.arthro.2009.12.026
- Gonzalez-Lomas G, Cassilly RT, Remotti F, Levine WN. Is the etiology of pretibial cyst formation after absorbable interference screw use related to a foreign body reaction? Clin Orthop Relat Res. 2011;469(4):1082-1088. doi: 10.1007/s11999-010-1580-5
- Warden WH, Chooljian D, Jackson DW. Ten-year magnetic resonance imaging follow-up of bioabsorbable poly-L-lactic acid interference screws after anterior cruciate ligament reconstruction. Arthroscopy. 2008;24(3):370.e1-370.e3. doi: 10.1016/j.arthro.2006.12.032
- Joshi YV, Bhaskar D, Phaltankar PM, Charalambous CP. Tibial tunnel cyst formation after anterior cruciate ligament reconstruction using a non-bioabsorbable interference screw. Knee Surg Relat Res. 2015;27(4):269-273. doi: 10.5792/ksrr.2015.27.4.269
- Chen CH, Chang WJ, Chen YS, et al. Development of a novel hybrid suture anchor for osteoporosis by integrating titanium 3D printing and traditional machining. Int J Bioprint. 2022;8(4):608.doi: 10.18063/ijb.v8i4.608
- Feng W, Jin Q, Ming-Yu Y, et al. MiR-6924-5p-rich exosomes derived from genetically modified Scleraxis-overexpressing PDGFRα(+) BMMSCs as novel nanotherapeutics for treating osteolysis during tendon-bone healing and improving healing strength. Biomaterials. 2021;279:121242. doi: 10.1016/j.biomaterials.2021.121242
- Guo D, Yang J, Liu D, Zhang P, Sun H, Wang J. Human umbilical cord mesenchymal stem cells overexpressing RUNX1 promote tendon-bone healing by inhibiting osteolysis, enhancing osteogenesis and promoting angiogenesis. Genes Genomics. 2024;46(4):461-473. doi: 10.1007/s13258-023-01478-3
- Liu Q, Yu Y, Reisdorf RL, et al. Engineered tendon-fibrocartilage-bone composite and bone marrow-derived mesenchymal stem cell sheet augmentation promotes rotator cuff healing in a non-weight-bearing canine model. Biomaterials. 2019;192:189-198. doi: 10.1016/j.biomaterials.2018.10.037
- Kobayashi Y, Kida Y, Kabuto Y, et al. Healing effect of subcutaneous administration of granulocyte colony-stimulating factor on acute rotator cuff injury in a rat model. Tissue Eng Part A. 2021;27(17-18):1205-1212. doi: 10.1089/ten.tea.2020.0239.A
- Hu Y, Ran J, Zheng Z, et al. Exogenous stromal derived factor-1 releasing silk scaffold combined with intra-articular injection of progenitor cells promotes bone-ligament-bone regeneration. Acta Biomater. 2018;71:168-183. doi: 10.1016/j.actbio.2018.02.019
- Guan G, Qizhuang L, Liu S, Jiang Z, Zhou C, Liao W. 3D-bioprinted peptide coupling patches for wound healing. Mater Today Bio. 2022;13:100188. doi: 10.1016/j.mtbio.2021.100188
- Chen P, Cui L, Chen G, et al. The application of BMP-12- overexpressing mesenchymal stem cells loaded 3D-printed PLGA scaffolds in rabbit rotator cuff repair. Int J Biol Macromol. 2019;138:79-88. doi: 10.1016/j.ijbiomac.2019.07.041
- Wang Z, Liang X, Wang G, Wang X, Chen Y. Emerging bioprinting for wound healing. Adv Mater. 2023:e2304738. doi: 10.1002/adma.202304738
- Zhong S, Lan Y, Liu J, et al. Advances focusing on the application of decellularization methods in tendon-bone healing. J Adv Res. 2025;67:361-372. doi: 10.1016/j.jare.2024.01.020
- Revathi S, Amanullah M, Al-Samghan AS, et al. Sustainable heavy metal (Cr(VI) ion) remediation: ternary blend approach with chitosan, carboxymethyl cellulose, and bioactive glass. Int J Biol Macromol. 2024;278(Pt 3):134769. doi: 10.1016/j.ijbiomac.2024.134769
- Liao H, Yu HP, Song W, et al. Amorphous calcium phosphate nanoparticles using adenosine triphosphate as an organic phosphorus source for promoting tendon-bone healing. J Nanobiotechnol. 2021;19(1):270. doi: 10.1186/s12951-021-01007-y
- Fang H, Xu J, Ma H, et al. Functional materials of 3D bioprinting for wound dressings and skin tissue engineering applications: a review. Int J Bioprint. 2023;9(5):757.
- Luo Y, Xu X, Ye Z, et al. 3D bioprinted mesenchymal stromal cells in skin wound repair. Front Surg. 2022;9:988843. doi: 10.3389/fsurg.2022.988843
- Mörö A, Samanta S, Honkamäki L, et al. Hyaluronic acid based next generation bioink for 3D bioprinting of human stem cell derived corneal stromal model with innervation. Biofabrication. 2022;15(1). doi: 10.1088/1758-5090/acab34
- Chen Y, Li Y, Zhu W, Liu Q. Biomimetic gradient scaffolds for the tissue engineering and regeneration of rotator cuff enthesis. Biofabrication. 2024;16(3). doi: 10.1088/1758-5090/ad467d
- Jeon O, Lee YB, Lee SJ, Guliyeva N, Lee J, Alsberg E. Stem cell-laden hydrogel bioink for generation of high resolution and fidelity engineered tissues with complex geometries. Bioact Mater. 2022;15:185-193. doi: 10.1016/j.bioactmat.2021.11.025
- Roshangar L, Rad JS, Kheirjou R, Khosroshahi AF. Using 3D-bioprinting scaffold loaded with adipose-derived stem cells to burns wound healing. J Tissue Eng Regen Med. 2021;15(6):546-555. doi: 10.1002/term.3194
- Jiang X, Wu S, Kuss M, et al. 3D printing of multilayered scaffolds for rotator cuff tendon regeneration. Bioact Mater. 2020;5(3):636-643. doi: 10.1016/j.bioactmat.2020.04.017
- Cao Y, Yang S, Zhao D, et al. Three-dimensional printed multiphasic scaffolds with stratified cell-laden gelatin methacrylate hydrogels for biomimetic tendon-to-bone interface engineering. J Orthop Transl. 2020;23: 89-100. doi: 10.1016/j.jot.2020.01.004
- Chae S, Sun Y, Choi YJ, Ha DH, Jeon I, Cho DW. 3D cell-printing of tendon-bone interface using tissue-derived extracellular matrix bioinks for chronic rotator cuff repair. Biofabrication. 2021;13(3). doi: 10.1088/1758-5090/abd159
- Chae S, Yong U, Park W, et al. 3D cell-printing of gradient multi-tissue interfaces for rotator cuff regeneration. Bioact Mater. 2023;19:611-625. doi: 10.1016/j.bioactmat.2022.05.004
- Zhang X, Li K, Wang C, et al. Facile and rapid fabrication of a novel 3D-printable, visible light-crosslinkable and bioactive polythiourethane for large-to-massive rotator cuff tendon repair. Bioact Mater. 2024;37:439-458. doi: 10.1016/j.bioactmat.2024.03.036
- Alkaissy R, Richard M, Morris H, et al. Manufacture of soft-hard implants from electrospun filaments embedded in 3D printed structures. Macromol Biosci. 2022; 22(12):e2200156. doi: 10.1002/mabi.202200156
- Han J, Han SC, Kim YK, et al. Bioactive scaffold with spatially embedded growth factors promotes bone-to-tendon interface healing of chronic rotator cuff tear in rabbit model. Am J Sports Med. 2023;51(9):2431-2442. doi: 10.1177/03635465231180289
- Han J, Han SC, Jeong HJ, et al. Recombinant human parathyroid hormone biocomposite promotes bone-to-tendon interface healing by enhancing tenogenesis, chondrogenesis, and osteogenesis in a rabbit model of chronic rotator cuff tears. Arthroscopy. 2024;40(4): 1093-1104.e2. doi: 10.1016/j.arthro.2023.09.034
- Wang T, Yu Z, Lin S, et al. 3D-printed Mg-incorporated PCL-based scaffolds improves rotator cuff tendon-bone healing through regulating macrophage polarization. Front Bioeng Biotechnol. 2024;12:1407512. doi: 10.3389/fbioe.2024.1407512
- Wu Z, Yang J, Chong H, et al. 3D-printed biomimetic scaffolds loaded with ADSCs and BMP-2 for enhanced rotator cuff repair. J Mater Chem B. 2024;12: 12365-12377. doi: 10.1039/d4tb01073f
- Chou YC, Yeh WL, Chao CL, et al. Enhancement of tendon-bone healing via the combination of biodegradable collagen-loaded nanofibrous membranes and a three-dimensional printed bone-anchoring bolt. Int J Nanomed. 2016;11:4173-4186. doi: 10.2147/ijn.S108939
- Parry JA, Olthof MG, Shogren KL, et al. Three-dimension-printed porous poly(propylene fumarate) scaffolds with delayed rhBMP-2 release for anterior cruciate ligament graft fixation. Tissue Eng Part A. 2017;23(7-8):359-365. doi: 10.1089/ten.TEA.2016.0343
- Wang Y, Ren C, Bi F, Li P, Tian K. The hydroxyapatite modified 3D printed poly L-lactic acid porous screw in reconstruction of anterior cruciate ligament of rabbit knee joint: a histological and biomechanical study. BMC Musculoskeletal Disord. 2023;24(1):151. doi: 10.1186/s12891-023-06245-9
- Antoniac I, Popescu D, Zapciu A, Antoniac A, Miculescu F, Moldovan H. Magnesium filled polylactic acid (PLA) material for filament based 3D printing. Materials (Basel). 2019;12(5):719. doi: 10.3390/ma12050719
- Huang YM, Huang CC, Tsai PI, et al. Three-dimensional printed porous titanium screw with bioactive surface modification for bone-tendon healing: a rabbit animal model. Int J Mol Sci. 2020;21(10):3628. doi: 10.3390/ijms21103628
- Park SH, Choi YJ, Moon SW, et al. Three-dimensional bio-printed scaffold sleeves with mesenchymal stem cells for enhancement of tendon-to-bone healing in anterior cruciate ligament reconstruction using soft-tissue tendon graft. Arthroscopy. 2018;34(1):166-179. doi: 10.1016/j.arthro.2017.04.016