GelMA–pectin–polyhedral silsesquioxane nanocomposites for 3D bioprinting of osteogenesis-stimulating scaffolds loaded with BMP-2
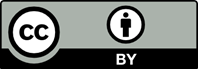
Harnessing the advantage of both naturally derived polymers and nanostructured materials, the current study presents a novel multicomponent hydrogel system double-reinforced with two complementary nanofillers, specifically designed for bioprinting-based tissue engineering applications. In a bioinspired approach, cellulose nanofibrils (CNFs) and polyhedral silsesquioxanes (PSS) nanoparticles were embedded in a proteinaceous–polysaccharidic matrix. To synthesize a robust platform for 3D bioprinting, methacrylate-modified biopolymers were ultraviolet-crosslinked, ensuring optimal conditions for cell encapsulation. The nanocomposite bioinks were supplemented with bone morphogenetic protein 2 (BMP-2), a potent osteogenic factor, to enhance the osteogenic differentiation of preosteoblasts. The 3D scaffold morphology was investigated, with a focus on PSS dispersion, porosity, and geometrical properties of the constructs. Swelling studies confirmed that all hydrogel samples retained their hydrophilic nature, though a slight reduction in swelling capacity was observed upon PSS incorporation. In vitro cytocompatibility tests demonstrated the beneficial effects of CNFs and PSS on cell growth. In vivo studies further revealed that hydrogels supplemented with nanostructured fillers and BMP-2 significantly enhanced osteogenesis, both in osteogenic and non-osteogenic regions. These findings prove that growth factor-reinforced scaffolds hold great potential in addressing the challenges of biomaterial research and represent a promising strategy for hard tissue regeneration.

- Lanza R, Langer R, Vacanti JP. Principles of Tissue Engineering. 5th ed. Academic Press; 2020.
- Tan B, Gan S, Wang X, Liu W, Li X. Applications of 3D bioprinting in tissue engineering: advantages, deficiencies, improvements, and future perspectives. J Mater Chem B. 2021;9(27):5385-5413. doi: 10.1039/D1TB00172H
- Fatimi A, Okoro OV, Podstawczyk D, Siminska-Stannym J, Shavandi A. Natural hydrogel-based bio-inks for 3D bioprinting in tissue engineering: a review. Gels. 2022;8(3):179. doi: 10.3390/gels8030179
- Swarnima A, Shreya S, Krishna BV, et al. Current developments in 3D bioprinting for tissue and organ regeneration–a review. Front Mech Eng. 2020;6: 2297-3079. doi: 10.3389/fmech.2020.589171
- Jang J, Park HJ, Kim SW, et al. 3D printed complex tissue construct using stem cell-laden decellularized extracellular matrix bioinks for cardiac repair. Biomater. 2017;112:264-274. doi: 10.1016/j.biomaterials.2016.10.026
- Keriquel V, Oliveira H, Rémy M, et al. In situ printing of mesenchymal stromal cells, by laser-assisted bioprinting, for in vivo bone regeneration applications. Sci Rep. 2017;7:1778. doi: 10.1038/s41598-017-01914-x
- Peloquin J, Han Y, Gall K. Printability and mechanical behavior as a function of base material, structure, and a wide range of porosities for polymer lattice structures fabricated by vat-based 3D printing. Addit Manuf. 2023;78:103892. doi: 10.1016/j.addma.2023.103892
- Kara Y, Kovács NK, Nagy-György P, Boros R, Molnár K. A novel method and printhead for 3D printing combined nano-/microfiber solid structures. Addit Manuf. 2023;61:103315. doi: 10.1016/j.addma.2022.103315
- Bisht B, Hope A, Mukherjee A, Paul MK. Advances in the fabrication of scaffold and 3D printing of biomimetic bone graft. Ann Biomed Eng. 2021;49:1128-1150. doi: 10.1007/s10439-021-02752-9
- Bahraminasab M. Challenges on optimization of 3D-printed bone scaffolds. Biomed Eng Online. 2020;19:69. doi: 10.1186/s12938-020-00810-2
- Alexa RL, Cucuruz A, Ghițulică CD, et al. 3D printable composite biomaterials based on GelMA and hydroxyapatite powders doped with cerium ions for bone tissue regeneration. Int J Mol Sci. 2022;23(3):1841. doi: 10.3390/ijms23031841
- Li Z, Wang Q, Liu G. A review of 3D printed bone implants. Micromachines. 2022;13(4):528. doi: 10.3390/mi13040528
- Alexa RL, Cucuruz A, Ghițulică CD, et al. 3D printed composite scaffolds of GelMA and hydroxyapatite nanopowders doped with Mg/Zn ions to evaluate the expression of genes and proteins of osteogenic markers. Nanomater. 2022;12(19):3420. doi: 10.3390/nano12193420
- Lee JM, Sing SL, Zhou M, Yeong WY. 3D bioprinting processes: a perspective on classification and terminology. Int J Bioprint. 2018;4(2):151. doi: 10.18063/IJB.v4i2.151
- Zhang YS, Haghiashtiani G, Hübscher T, et al. 3D extrusion bioprinting. Nat Rev Methods Primers. 2021;1:75. doi: 10.1038/s43586-021-00073-8
- Karvinen J, Kellomäki M. Design aspects and characterization of hydrogel-based bioinks for extrusion-based bioprinting. Bioprinting. 2023;32:e00274. doi: 10.1016/j.bprint.2023.e00274
- Ng WL, Shkolnikov V. Jetting-based bioprinting: process, dispense physics, and applications. Bio-des. Manuf. 2024;7:771-799. doi: 10.1007/s42242-024-00285-3
- Li Y, Zhang X, Zhang X, Zhang Y, Hou D. Recent progress of the vat photopolymerization technique in tissue engineering: a brief review of mechanisms, methods, materials, and applications. Polym. 2023;15(9):3940. doi: 10.3390/polym15193940
- Jooken S, Deschaume O, Bartic C. Nanocomposite hydrogels as functional extracellular matrices. Gels. 2023;9(2):153. doi: 10.3390/gels9020153
- Zhang XN, Zheng Q, Wu ZL. Recent advances in 3D printing of tough hydrogels: a review. Composites B: Eng. 2022;238:109895. doi: 10.1016/j.compositesb.2022.109895
- Chimene D, Kaunas R, Gaharwar AK. Hydrogel bioink reinforcement for additive manufacturing: a focused review of emerging strategies. Adv Mater. 2020;32(1):1902026. doi: 10.1002/adma.201902026
- Curti F, Serafim A, Olaret E, et al. Development of biocomposite alginate-cuttlebone-gelatin 3D printing inks designed for scaffolds with bone regeneration potential. Mar Drugs. 2022;20(11):670. doi: 10.3390/md20110670
- Curti F, Stancu IC, Voicu G, et al. Development of 3D bioactive scaffolds through 3D printing using Wollastonite– Gelatin inks. Polym. 2020;12(10):2420. doi: 10.3390/polym12102420
- Bhattacharyya A, Janarthanan G, Tran HN, et al. Bioink homogeneity control during 3D bioprinting of multicomponent micro/nanocomposite hydrogel for even tissue regeneration using novel twin screw extrusion system. Chem Eng J. 2021;415:128971. doi: 10.1016/j.cej.2021.128971
- Prince E, Kumacheva E. Design and applications of man-made biomimetic fibrillar hydrogels. Nat Rev Mater. 2019;4:99-115. doi: 10.1038/s41578-018-0077-9
- Cernencu AI, Lungu A, Stancu IC, et al. Bioinspired 3D printable pectin-nanocellulose ink formulations. Carbohydr Polym. 2019;220:12-21. doi: 10.1016/j.carbpol.2019.05.026
- Ojansivu M, Rashad A, Ahlinder A, et al. Wood-based nanocellulose and bioactive glass modified gelatin-alginate bioinks for 3D bioprinting of bone cells. Biofabr. 2019;11(3):035010. doi: 10.1088/1758-5090/ab0692
- Nosrati H, Mamoory RS, Le DQS, Bünger CE. Fabrication of gelatin/hydroxyapatite/3D-graphene scaffolds by a hydrogel 3D-printing method. Mater Chem Phys. 2020; 239:122305. doi: 10.1016/j.matchemphys.2019.122305
- Kaya H, Arici Ş, Bulut O, Bilgili F, Ege D. CNT incorporation improves the resolution and stability of porous 3D printed PLGA/HA/CNT scaffolds for bone regeneration. Mater. 2023;18:055028. doi: 10.1088/1748-605X/acf25d
- Cernencu AI, Dinu AI, Dinescu S, et al. Inorganic/ biopolymers hybrid hydrogels dual crosslinked for bone tissue regeneration. Gels. 2022;8(12):762. doi: 10.3390/gels8120762
- Marin MM, Gifu IC, Pircalabioru GG, et al. Microbial polysaccharide-based formulation with silica nanoparticles; a new hydrogel nanocomposite for 3D printing. Gels. 2023;9(5):425. doi: 10.3390/gels9050425
- Cernencu AI, Vlasceanu GM, Serafim A, Pircalabioru G, Ionita M. 3D double-reinforced graphene oxide - nanocellulose biomaterial inks for tissue engineered constructs. RSC Adv. 2023;10(34):24053-24063. doi: 10.1039/d3ra02786d
- Martins dos Santos D, Moon JI, Kim DS, et al. Hierarchical chitin nanocrystal-based 3D printed dual-layer membranes hydrogels: a dual drug delivery nano-platform for periodontal tissue regeneration. ACS Nano. 2024;18(35):24182-24203. doi: 10.1021/acsnano.4c05558
- Kim N, Lee H, Han G, et al. 3D-printed functional hydrogel by DNA-induced biomineralization for accelerated diabetic wound healing. Adv Sci. 2023;10:2300816. doi: 10.1002/advs.202300816
- Cassimjee H, Kumar P, Choonara YE, Pillay V. Proteosaccharide combinations for tissue engineering applications. Carbohydr Polym. 2020;235:115932. doi: 10.1016/j.carbpol.2020.115932
- Aldana AA, Valente F, Dilley R, Doyle B. Development of 3D bioprinted GelMA-alginate hydrogels with tunable mechanical properties. Bioprint. 2021;21:e00105. doi: 10.1016/j.bprint.2020.e00105
- Allen NB, Abar B, Johnson L, et al. 3D-bioprinted GelMA-gelatin-hydroxyapatite osteoblast-laden composite hydrogels for bone tissue engineering. Bioprint. 2022;26:e00196. doi: 10.1016/j.bprint.2022.e00196
- Cernencu AI, Lungu A, Dragusin DM, et al. 3D bioprinting of biosynthetic nanocellulose-filled GelMA inks highly reliable for soft tissue-oriented constructs. Mater. 2021; 14(17):4891. doi: 10.3390/ma14174891
- Li Z, Li S, Yang J, et al. 3D bioprinted gelatin/gellan gum-based scaffold with double-crosslinking network for vascularized bone regeneration. Carbohydr Polym. 2022;290:119469. doi: 10.1016/j.carbpol.2022.119469
- Wu Z, Xie S, Kang Y, et al. Biocompatibility evaluation of a 3D-bioprinted alginate-GelMA-bacteria nanocellulose (BNC) scaffold laden with oriented-growth RSC96 cells. Mater Sci Eng C. 2021;129:112393. doi: 10.1016/j.msec.2021.112393
- Xu W, Molino BZ, Cheng F, et al. On low-concentration inks formulated by nanocellulose assisted with gelatin methacrylate (GelMA) for 3D printing toward wound healing application. ACS Appl Mater Interf. 2019;11(9):8838-8848. doi: 10.1021/acsami.8b21268
- Zhang M, Yang F, Han D, et al. 3D bioprinting of corneal decellularized extracellular matrix: GelMA composite hydrogel for corneal stroma engineering. Int J Bioprinting. 2023;9(5):774. doi: 10.18063/ijb.774
- Lou J, Mooney DJ. Chemical strategies to engineer hydrogels for cell culture. Nat Rev Chem. 2022;6:726-744. doi: 10.1038/s41570-022-00420-7
- Wang W, Guo Y, Otaigbe JU. The synthesis, characterization and biocompatibility of poly(ester urethane)/polyhedral oligomeric silesquioxane nanocomposites. Polym. 2009;50(24): 5749-5757. doi: 10.1016/j.polymer.2009.05.037
- Liu C, Chiang B, Lewin Mejia D, et al. Mammary fibroblasts remodel fibrillar collagen microstructure in a biomimetic nanocomposite hydrogel. Acta Biomater. 2018;83:221-232. doi: 10.1016/j.actbio.2018.11.010
- Liu C, Lucker K, Lucker G. POSS Nanocomposite Hydrogel for 3D Bioprinting; 2019. US20190328930A1
- Dudman J, Ferreira AM, Gentile P, Wang X, Dalgarno K. Microvalve bioprinting of MSC-chondrocyte co-cultures. Cells. 2021;10(12):3329. doi: 10.3390/cells10123329
- Li X, Liu B, Pei B, et al. Inkjet bioprinting of biomaterials. Chem Rev. 2020;120(19):10793-10833. doi: 10.1021/acs.chemrev.0c00008
- Ng WL, Huang X, Shkolnikov V, Suntornnond R, Yeong WY. Polyvinylpyrrolidone-based bioink: influence of bioink properties on printing performance and cell proliferation during inkjet-based bioprinting. Bio-des Manuf. 2023;6:676-690. doi: 10.1007/s42242-023-00245-3
- Pereira FR, Sousa A, Barrias CC, Bártolo PJ, Granja PL. A single-component hydrogel bioink for bioprinting of bioengineered 3D constructs for dermal tissue engineering. Mater Horiz. 2018;5(6):1100-1111. doi: 10.1039/C8MH00525G
- Lungu A, Cernencu AI, Dinescu S, et al. Nanocellulose-enriched hydrocolloid-based hydrogels designed using a Ca2+ free strategy based on citric acid. Mater Des. 2021;197:109200. doi: 10.1016/j.matdes.2020.109200
- Cernencu A, Lungu A, Stancu IC, Vasile E, Iovu H. Polysaccharide-based 3d printing inks supplemented with additives. UPB Sci Bull Ser B Chem Mater Sci. 2019;81(4):175-186. https://www.scientificbulletin.upb.ro/SeriaB_-_Chimie_si_ Stiinta_Materialelor.php?page=revistaonline&a=2&arh_ an=2019&arh_ser=B&arh_nr=4
- Heggebö J, Haasters F, Polzer, H., et al. Aged human mesenchymal stem cells: the duration of bone morphogenetic protein-2 stimulation determines induction or inhibition of osteogenic differentiation. Orthopedic Rev. 2014;6(2):5242. doi: 10.4081/or.2014.5242
- Park JH, Koh EB, Seo YJ, Oh HS, Byun JH. BMP-9 improves the osteogenic differentiation ability over BMP-2 through p53 signaling in vitro in human periosteum-derived cells. Int J Mol Sci. 2023;24(20):15252. doi: 10.3390/ijms242015252
- Wu Y, Wenger A, Golzar H, Thang X. 3D bioprinting of bicellular liver lobule-mimetic structures via microextrusion of cellulose nanocrystal-incorporated shear-thinning bioink. Sci Rep. 2020;10:20648. doi: 10.1038/s41598-020-77146-3
- Olăreț E, Dinescu S, Dobranici AE, et al. Osteoblast responsive biosilica-enriched gelatin microfibrillar microenvironments. Biomater Adv. 2024;161:213894. doi: 10.1016/j.bioadv.2024.213894
- Șelaru A, Herman H, Vlăsceanu GM, et al. Graphene–oxide porous biopolymer hybrids enhance in vitro osteogenic differentiation and promote ectopic osteogenesis in vivo. Int J Mol Sci. 2022;23(1):491. doi: 10.3390/ijms23010491
- Hermenean A, Codreanu A, Herman H, et al. Chitosan-graphene oxide 3D scaffolds as promising tools for bone regeneration in critical-size mouse calvarial defects. Sci Rep. 2017;7:16641. doi: 10.1038/s41598-017-16599-5
- Patntirapong S, Chanruangvanit C, Lavanrattanakul K, Satravaha Y. Assessment of bisphosphonate treated-osteoblast behaviors by conventional assays and a simple digital image analysis. Acta Histochem. 2021;123(1):151659. doi: 10.1016/j.acthis.2020.151659
- Huang D, Li R, Ren J, et al. Temporal induction of Lhx8 by optogenetic control system for efficient bone regeneration. Stem Cell Res Ther. 2021;12:339. doi: 10.1186/s13287-021-02412-8
- Korkeamäki JT, Rashad A, Berstad K, et al. Biomimetic highly porous nanocellulose–nanohydroxyapatite scaffolds for bone tissue engineering. Cellulose. 2024;31:1-19. doi: 10.1007/s10570-024-05732-z
- Șelaru A, Mocanu-Dobranici AE, Olăreț E, et al. Gelatin meshes enriched with graphene oxide and magnetic nanoparticles support and enhance the proliferation and neuronal differentiation of human adipose-derived stem cells. Int J Mol Sci. 2023;24(1):555. doi: 10.3390/ijms24010555
- Olăreț E, Drăgușin DM, Serafim A, et al. Electrospinning fabrication and cytocompatibility investigation of nanodiamond particles-gelatin fibrous tubular scaffolds for nerve regeneration. Polym. 2021;13(3):407. doi: 10.3390/polym13030407
- Shi H, Yang J, You M, Li Z, He C. Polyhedral oligomeric silsesquioxanes (POSS)-based hybrid soft gels: molecular design, material advantages, and emerging applications. ACS Mater Lett. 2020;2(4):296-316. doi: 10.1021/acsmaterialslett.9b00491
- Choi E, Kim D, Kang D, et al. 3D-printed gelatin methacrylate (GelMA)/silanated silica scaffold assisted by two-stage cooling system for hard tissue regeneration. Regen Biomater. 2021;8(2):rbab001. doi: 10.1093/rb/rbab001
- Zonderland J, Moroni L. Steering cell behavior through mechanobiology in 3D: a regenerative medicine perspective. Biomater. 2021;268:120572. doi: 10.1016/j.biomaterials.2020.120572
- Sahebalzamani M, Ziminska M, McCarthy HO, et al. Advancing bone tissue engineering one layer at a time: a layer-by-layer assembly approach to 3D bone scaffold materials. Biomater Sci. 2022;10(11):2734-2758. doi: 10.1039/d1bm01756j
- Wong SK, Yee MMF, Chin KY, Ima-Nirwana S. A review of the application of natural and synthetic scaffolds in bone regeneration. J Funct Biomater. 2023;14(5):286. doi: 10.3390/jfb14050286
- Soleymani S, Naghib SM. 3D and 4D printing hydroxyapatite-based scaffolds for bone tissue engineering and regeneration. Heliyon. 2023;9(9):e19363. doi: 10.1016/j.heliyon.2023.e19363
- Yu J, Park SA, Kim WD, et al. Current advances in 3D bioprinting technology and its applications for tissue engineering. Polym (Basel). 2020;12(12):2958. doi: 10.3390/polym12122958
- Park JY, Choi YJ, Shim JH, Park JH, Cho DW. Development of a 3D cell printed structure as an alternative to autologs cartilage for auricular reconstruction. J Biomed Mater Res B Appl Biomater. 2017;105(5):1016-1028. doi: 10.1002/jbm.b.33639
- Guillemot F, Mironov V, Nakamura M. Bioprinting is coming of age: report from the international conference on bioprinting and biofabrication in Bordeaux. Biofabrication. 2010;2(1):010201. doi: 10.1088/1758-5082/2/1/010201
- Kim W, Kim G. Collagen/bioceramic-based composite bioink to fabricate a porous 3D hASCs-laden structure for bone tissue regeneration. Biofabrication. 2019;12(1):015007. doi: 10.1088/1758-5090/ab436d
- Das S, Pati F, Choi YJ, et al. Bioprintable, cell-laden silk fibroin-gelatin hydrogel supporting multilineage differentiation of stem cells for fabrication of three-dimensional tissue constructs. Acta Biomater. 2015;11:233-246. doi: 10.1016/j.actbio.2014.09.023
- Im S, Choe G, Seok JM, et al. An osteogenic bioink composed of alginate, cellulose nanofibrils, and polydopamine nanoparticles for 3D bioprinting and bone tissue engineering. Int J Biol Macromol. 2022;205:520-529. doi: 10.1016/j.ijbiomac.2022.02.012
- Janmohammadi M, Nazemi Z, Salehi AOM, et al. Cellulose-based composite scaffolds for bone tissue engineering and localized drug delivery. Bioact Mater. 2023; 20:137-163. doi: 10.1016/j.bioactmat.2022.05.018
- Zhu S, Chen W, Masson A, Li YP. Cell signaling and transcriptional regulation of osteoblast lineage commitment, differentiation, bone formation, and homeostasis. Cell Discov. 2024;10(1):71. doi: 10.1038/s41421-024-00689-6
- Kim YH, Dawson JI, Oreffo ROC, et al. Gelatin methacryloyl hydrogels for musculoskeletal tissue regeneration. Bioeng. 2022;9(7):332. doi: 10.3390/bioengineering9070332
- Jun L, Wenzhao W, Mingxin L, et al. Biomimetic methacrylated gelatin hydrogel loaded with bone marrow mesenchymal stem cells for bone tissue regeneration. Front Bioeng Biotechnol. 2021;9:770049. doi: 10.3389/fbioe.2021.770049
- Yi MH, Lee JE, Kim CB, Lee KW, Lee KH. Locally controlled diffusive release of bone morphogenetic protein-2 using micropatterned gelatin methacrylate hydrogel carriers. BioChip J. 2020;14:405-420. doi: 10.1007/s13206-020-4411-0
- Munarin F, Guerreiro SG, Grellier MA, et al. Pectin-based injectable biomaterials for bone tissue engineering. Biomacromol. 2011;12(3):568-577. doi: 10.1021/bm101110x
- Di Pietro L, Ravizza A, Vozzi G, et al. European regulatory framework for the clinical translation of bioprinted scaffolds and tissues. Biomed Sci Eng. 2020;1(1):108. doi: 10.4081/bse.2019.108