Bioprinting in tumor model construction for head and neck squamous cell carcinoma: A review
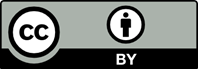
Head and neck squamous cell carcinoma (HNSCC) is a malignancy with increasing incidence worldwide, causing a severe impact on the quality of life and survival rate of affected patients. Traditional tumor models have significant limitations in studying biological properties, tumor microenvironment, and treatment response, and the difficulty in obtaining HNSCC specimens has hampered our understanding and the development of treatment for the disease. Recent rapid development in bioprinting has provided new possibilities for tumor model construction, enabling precise control of cell arrangement and tissue structure to be more realistically simulated tumor biological properties. This review summarizes the latest progress of bioprinting in HNSCC tumor model construction, explores the application of different bioprinting technologies, the properties of constructed tumor models, and the potential applications of these models in drug screening and individualized treatment, aiming to provide reference and inspiration for future research and treatment of HNSCC.

- Lang Y, Dong D. Cetuximab plus chemotherapy versus chemotherapy alone in recurrent or metastatic head and neck squamous cell carcinoma: a cost-effectiveness analysis. Cancer Manag Res. 2020;12:11383-11390. doi: 10.2147/CMAR.S272149
- Liu WH, Lu YN, Sun MT, Nie DH, Han FJ. Trends of ten leading causes of death in head and neck squamous cell carcinoma. Curr Med Sci. 2022;42(1):118-128. doi: 10.1007/s11596-021-2427-x
- Stribbling SM, Ryan AJ. The cell-line-derived subcutaneous tumor model in preclinical cancer research. Nat Protoc. 2022;17(9):2108-2128. doi: 10.1038/s41596-022-00709-3
- Buque A, Galluzzi L. Modeling tumor immunology and immunotherapy in mice. Trends Cancer. 2018;4(9): 599-601. doi: 10.1016/j.trecan.2018.07.003
- Shapiro DD, Virumbrales-Munoz M, Beebe DJ, Abel EJ. Models of renal cell carcinoma used to investigate molecular mechanisms and develop new therapeutics. Front Oncol. 2022;12:871252. doi: 10.3389/fonc.2022.871252
- Tentler JJ, Tan AC, Weekes CD, et al. Patient-derived tumour xenografts as models for oncology drug development. Nat Rev Clin Oncol. 2012;9(6):338-350. doi: 10.1038/nrclinonc.2012.61
- Lambert PF. Transgenic mouse models of tumor virus action. Annu Rev Virol. 2016;3(1):473-489. doi: 10.1146/annurev-virology-100114-054908
- Zitvogel L, Pitt JM, Daillere R, Smyth MJ, Kroemer G. Mouse models in oncoimmunology. Nat Rev Cancer. 2016;16(12):759-773. doi: 10.1038/nrc.2016.91
- Olson B, Li Y, Lin Y, Liu ET, Patnaik A. Mouse models for cancer immunotherapy research. Cancer Discov. 2018;8(11):1358-1365. doi: 10.1158/2159-8290.CD-18-0044
- Nitschinsk K, Idris A, McMillan N. Patient derived xenografts as models for head and neck cancer. Cancer Lett. 2018;434:114-119. doi: 10.1016/j.canlet.2018.07.023
- Karamboulas C, Bruce JP, Hope AJ, et al. Patient-derived xenografts for prognostication and personalized treatment for head and neck squamous cell carcinoma. Cell Rep. 2018;25(5):1318-1331 e4. doi: 10.1016/j.celrep.2018.10.004
- Cox MC, Reese LM, Bickford LR, Verbridge SS. Toward the broad adoption of 3D tumor models in the cancer drug pipeline. ACS Biomater Sci Eng. 2015;1(10): 877-894. doi: 10.1021/acsbiomaterials.5b00172
- Jung AR, Jung CH, Noh JK, Lee YC, Eun YG. Epithelial-mesenchymal transition gene signature is associated with prognosis and tumor microenvironment in head and neck squamous cell carcinoma. Sci Rep. 2020;10(1):3652. doi: 10.1038/s41598-020-60707-x
- He Y, Deng P, Yan Y, et al. Matrisome provides a supportive microenvironment for oral squamous cell carcinoma progression. J Proteomics. 2022;253:104454. doi: 10.1016/j.jprot.2021.104454
- Kimlin LC, Casagrande G, Virador VM. In vitro three-dimensional (3D) models in cancer research: an update. Mol Carcinog. 2013;52(3):167-182. doi: 10.1002/mc.21844
- Fonseca AC, Melchels FPW, Ferreira MJS, et al. Emulating human tissues and organs: a bioprinting perspective toward personalized medicine. Chem Rev. 2020;120(19): 11128-11174. doi: 10.1021/acs.chemrev.0c00342
- Datta P, Barui A, Wu Y, Ozbolat V, Moncal KK, Ozbolat IT. Essential steps in bioprinting: from pre- to post-bioprinting. Biotechnol Adv. 2018;36(5):1481-1504. doi: 10.1016/j.biotechadv.2018.06.003
- Bone JM, Childs CM, Menon A, et al. Hierarchical machine learning for high-fidelity 3D printed biopolymers. ACS Biomater Sci Eng. 2020;6(12):7021-7031. doi: 10.1021/acsbiomaterials.0c00755
- Xie M, Su J, Zhou S, Li J, Zhang K. Application of hydrogels as three-dimensional bioprinting ink for tissue engineering. Gels. 2023;9(2):88. doi: 10.3390/gels9020088
- Kravchenko SV, Sakhnov SN, Myasnikova VV, Trofimenko AI, Buzko VY. Bioprinting technologies in ophthalmology. Vestn Oftalmol. 2023;139(5):105-112. doi: 10.17116/oftalma2023139051105
- Ravanbakhsh H, Karamzadeh V, Bao G, Mongeau L, Juncker D, Zhang YS. Emerging technologies in multi-material bioprinting. Adv Mater. 2021;33(49):e2104730. doi: 10.1002/adma.202104730
- Meng F, Meyer CM, Joung D, Vallera DA, McAlpine MC, Panoskaltsis-Mortari A. 3D bioprinted in vitro metastatic models via reconstruction of tumor microenvironments. Adv Mater. 2019;31(10):e1806899. doi: 10.1002/adma.201806899
- Li J, Parra-Cantu C, Wang Z, Zhang YS. Improving bioprinted volumetric tumor microenvironments in vitro. Trends Cancer. 2020;6(9):745-756. doi: 10.1016/j.trecan.2020.06.002
- Shukla P, Yeleswarapu S, Heinrich MA, Prakash J, Pati F. Mimicking tumor microenvironment by 3D bioprinting: 3D cancer modeling. Biofabrication. 2022;14(3):032002. doi: 10.1088/1758-5090/ac6d11
- Hynes WF, Pepona M, Robertson C, et al. Examining metastatic behavior within 3D bioprinted vasculature for the validation of a 3D computational flow model. Sci Adv. 2020;6(35):eabb3308. doi: 10.1126/sciadv.abb3308
- Ma X, Liu J, Zhu W, et al. 3D bioprinting of functional tissue models for personalized drug screening and in vitro disease modeling. Adv Drug Deliv Rev. 2018;132:235-251. doi: 10.1016/j.addr.2018.06.011
- Shukla P, Bera AK, Ghosh A, Kiranmai G, Pati F. Assessment and process optimization of high throughput biofabrication of immunocompetent breast cancer model for drug screening applications. Biofabrication. 2024;16(3):035030. doi: 10.1088/1758-5090/ad586b
- Jung M, Ghamrawi S, Du EY, Gooding JJ, Kavallaris M. Advances in 3D bioprinting for cancer biology and precision medicine: from matrix design to application. Adv Healthc Mater. 2022;11(24):e2200690. doi: 10.1002/adhm.202200690
- Mandrycky C, Wang Z, Kim K, Kim DH. 3D bioprinting for engineering complex tissues. Biotechnol Adv. 2016;34(4): 422-434. doi: 10.1016/j.biotechadv.2015.12.011
- Murphy SV, Atala A. 3D bioprinting of tissues and organs. Nat Biotechnol. 2014;32(8):773-785. doi: 10.1038/nbt.2958
- Daly AC, Prendergast ME, Hughes AJ, Burdick JA. Bioprinting for the Biologist. Cell. 2021;184(1):18-32. doi: 10.1016/j.cell.2020.12.002
- Ozbolat IT, Hospodiuk M. Current advances and future perspectives in extrusion-based bioprinting. Biomaterials. 2016;76:321-343. doi: 10.1016/j.biomaterials.2015.10.076
- Ji S, Guvendiren M. Complex 3D bioprinting methods. APL Bioeng. 2021;5(1):011508. doi: 10.1063/5.0034901
- Cui X, Li J, Hartanto Y, et al. Advances in extrusion 3D bioprinting: a focus on multicomponent hydrogel-based bioinks. Adv Healthc Mater. 2020;9(15):e1901648. doi: 10.1002/adhm.201901648
- Fu Z, Naghieh S, Xu C, Wang C, Sun W, Chen X. Printability in extrusion bioprinting. Biofabrication. 2021;13(3):033001. doi: 10.1088/1758-5090/abe7ab
- Kacarevic ZP, Rider PM, Alkildani S, et al. An introduction to 3D bioprinting: possibilities, challenges and future aspects. Materials (Basel). 2018;11(11):2199. doi: 10.3390/ma11112199
- Salih T, Caputo M, Ghorbel MT. Recent advances in hydrogel-based 3D bioprinting and its potential application in the treatment of congenital heart disease. Biomolecules. 2024;14(7):861. doi: 10.3390/biom14070861
- Castilho M, de Ruijter M, Beirne S, et al. Multitechnology biofabrication: a new approach for the manufacturing of functional tissue structures? Trends Biotechnol. 2020;38(12):1316-1328. doi: 10.1016/j.tibtech.2020.04.014
- De Santis MM, Alsafadi HN, Tas S, et al. Extracellular-matrix-reinforced bioinks for 3D bioprinting human tissue. Adv Mater. 2021;33(3):e2005476. doi: 10.1002/adma.202005476
- Faramarzi N, Yazdi IK, Nabavinia M, et al. Patient-specific bioinks for 3D bioprinting of tissue engineering scaffolds. Adv Healthc Mater. 2018;7(11):e1701347. doi: 10.1002/adhm.201701347
- Wendt D, Riboldi SA, Cioffi M, Martin I. Potential and bottlenecks of bioreactors in 3D cell culture and tissue manufacturing. Adv Mater. 2009;21(32–33):3352-3367. doi: 10.1002/adma.200802748
- Jiang B, Elkashif A, Coulter JA, Dunne NJ, McCarthy HO. Immunotherapy for HPV negative head and neck squamous cell carcinoma. Biochim Biophys Acta Rev Cancer. 2024;1879(5):189138. doi: 10.1016/j.bbcan.2024.189138
- Liu L, Xiang Z, Li Y, et al. The immune checkpoint inhibitors treatment of head and neck squamous cell carcinoma: an expert consensus. Hua Xi Kou Qiang Yi Xue Za Zhi. 2022;40(6):619-628. doi: 10.7518/hxkq.2022.06.001
- Barlak N, Kusdemir G, Gumus R, et al. Overexpression of POFUT1 promotes malignant phenotype and mediates perineural invasion in head and neck squamous cell carcinoma. Cell Biol Int. 2023;47(12):1950-1963. doi: 10.1002/cbin.12085
- Santi M, Mapanao AK, Cappello V, Voliani V. Production of 3D tumor models of head and neck squamous cell carcinomas for nanotheranostics assessment. ACS Biomater Sci Eng. 2020;6(9):4862-4869. doi: 10.1021/acsbiomaterials.0c00617
- Xu JH, Guan YJ, Qiu ZD, et al. System analysis of ROS-related genes in the prognosis, immune infiltration, and drug sensitivity in hepatocellular carcinoma. Oxid Med Cell Longev. 2021;2021:6485871. doi: 10.1155/2021/6485871
- Nie Q, Cao H, Yang J, Liu T, Wang B. Integration RNA bulk and single cell RNA sequencing to explore the change of glycolysis-related immune microenvironment and construct prognostic signature in head and neck squamous cell carcinoma. Transl Oncol. 2024;46:102021. doi: 10.1016/j.tranon.2024.102021
- Bancu A, Cowan R, Chaturvedi A. PD-L1 testing and immunotherapy selection - early laboratory experience and its potential role in head and neck cancer management. Arch Clin Cases. 2021;8(1):14-18. doi: 10.22551/2021.30.0801.10179
- Businello G, Fassan M, Degasperi S, et al. Esophageal squamous cell carcinoma metachronous to head and neck cancers. Pathol Res Pract. 2021;219:153346. doi: 10.1016/j.prp.2021.153346
- Noro J, Vilaca-Faria H, Reis RL, Pirraco RP. Extracellular matrix-derived materials for tissue engineering and regenerative medicine: a journey from isolation to characterization and application. Bioact Mater. 2024;34: 494-519. doi: 10.1016/j.bioactmat.2024.01.004
- Kort-Mascort J, Bao G, Elkashty O, et al. Decellularized extracellular matrix composite hydrogel bioinks for the development of 3D bioprinted head and neck in vitro tumor models. ACS Biomater Sci Eng. 2021;7(11): 5288-5300. doi: 10.1021/acsbiomaterials.1c00812
- Kort-Mascort J, Shen ML, Martin E, et al. Bioprinted cancer-stromalin-vitromodels in a decellularized ECM-based bioink exhibit progressive remodeling and maturation. Biomed Mater. 2023;18(4):045022. doi: 10.1088/1748-605X/acd830
- Mapanao AK, Santi M, Voliani V. Combined chemo-photothermal treatment of three-dimensional head and neck squamous cell carcinomas by gold nano-architectures. J Colloid Interface Sci. 2021;582(Pt B):1003-1011. doi: 10.1016/j.jcis.2020.08.059
- McGarry K, Sefat E, Suh TC, Ali KM, Gluck JM. Comparison of NIH 3T3 cellular adhesion on fibrous scaffolds constructed from natural and synthetic polymers. Biomimetics (Basel). 2023;8(1):99. doi: 10.3390/biomimetics8010099
- Zhou R, Wu Y, Chen K, et al. A polymeric strategy empowering vascular cell selectivity and potential application superior to extracellular matrix peptides. Adv Mater. 2022;34(42):e2200464. doi: 10.1002/adma.202200464
- Lauren I, Farzan A, Teotia A, Lindfors NC, Seppala J. Direct ink writing of biocompatible chitosan/non-isocyanate polyurethane/cellulose nanofiber hydrogels for wound-healing applications. Int J Biol Macromol. 2024; 259(Pt 2):129321. doi: 10.1016/j.ijbiomac.2024.129321
- Kim SB, Kim CH, Lee SY, Park SJ. Carbon materials and their metal composites for biomedical applications: a short review. Nanoscale. 2024;16(35):16313-16328. doi: 10.1039/d4nr02059f
- Kost B, Brzezinski M, Socka M, Basko M, Biela T. Biocompatible polymers combined with cyclodextrins: fascinating materials for drug delivery applications. Molecules. 2020;25(15):3404. doi: 10.3390/molecules25153404
- Rivero RE, Capella V, Cecilia Liaudat A, et al. Mechanical and physicochemical behavior of a 3D hydrogel scaffold during cell growth and proliferation. RSC Adv. 2020;10(10): 5827-5837. doi: 10.1039/c9ra08162c
- Xu Y, Bei Z, Li M, et al. Biomedical application of materials for external auditory canal: history, challenges, and clinical prospects. Bioact Mater. 2024;39:317-335. doi: 10.1016/j.bioactmat.2024.05.035
- Battigelli A, Almeida B, Shukla A. Recent advances in bioorthogonal click chemistry for biomedical applications. Bioconjug Chem. 2022;33(2):263-271. doi: 10.1021/acs.bioconjchem.1c00564
- Di Martino M, Sessa L, Diana R, Piotto S, Concilio S. Recent progress in photoresponsive biomaterials. Molecules. 2023;28(9):3712. doi: 10.3390/molecules28093712
- Yoon S, Fuwad A, Jeong S, Cho H, Jeon TJ, Kim SM. Surface deformation of biocompatible materials: recent advances in biological applications. Biomimetics (Basel). 2024; 9(7):395. doi: 10.3390/biomimetics9070395
- Li H, Wang P, Wen C. Recent progress on nanocrystalline metallic materials for biomedical applications. Nanomaterials (Basel). 2022;12(12):2111. doi: 10.3390/nano12122111
- Jiang Q, Zhang M, Mujumdar AS. Novel evaluation technology for the demand characteristics of 3D food printing materials: a review. Crit Rev Food Sci Nutr. 2022;62(17):4669-4683. doi: 10.1080/10408398.2021.1878099
- Patel AS, Yanai I. A developmental constraint model of cancer cell states and tumor heterogeneity. Cell. 2024;187(12): 2907-2918. doi: 10.1016/j.cell.2024.04.032
- Puram SV, Tirosh I, Parikh AS, et al. Single-cell transcriptomic analysis of primary and metastatic tumor ecosystems in head and neck cancer. Cell. 2017;171(7): 1611-1624 e24. doi: 10.1016/j.cell.2017.10.044
- Xue Y, Friedl V, Ding H, Wong CK, Stuart JM. Single-cell signatures identify microenvironment factors in tumors associated with patient outcomes. Cell Rep Methods. 2024;4(6):100799. doi: 10.1016/j.crmeth.2024.100799
- Zhang Y, Chen P, Zhou Q, et al. A novel immune-related prognostic signature in head and neck squamous cell carcinoma. Front Genet. 2021;12:570336. doi: 10.3389/fgene.2021.570336
- Lynch AW, Brown M, Meyer CA. Multi-batch single-cell comparative atlas construction by deep learning disentanglement. Nat Commun. 2023;14(1):4126. doi: 10.1038/s41467-023-39494-2
- Sun H, Sun L, Ke X, et al. Prediction of clinical precision chemotherapy by patient-derived 3D bioprinting models of colorectal cancer and its liver metastases. Adv Sci (Weinh). 2024;11(2):e2304460. doi: 10.1002/advs.202304460
- Lee TW, Lai A, Harms JK, et al. Patient-derived xenograft and organoid models for precision medicine targeting of the tumour microenvironment in head and neck cancer. Cancers (Basel). 2020;12(12):3743. doi: 10.3390/cancers12123743
- Dai Y, Wang Z, Xia Y, et al. Integrative single-cell and bulk transcriptomes analyses identify intrinsic HNSCC subtypes with distinct prognoses and therapeutic vulnerabilities. Clin Cancer Res. 2023;29(15):2845-2858. doi: 10.1158/1078-0432.CCR-22-3563
- Gilbert DF, Friedrich O, Wiest J. Assaying proliferation characteristics of cells cultured under static versus periodic conditions. Methods Mol Biol. 2023;2644:35-45. doi: 10.1007/978-1-0716-3052-5_3
- Ciccarese D, Micali G, Borer B, Ruan C, Or D, Johnson DR. Rare and localized events stabilize microbial community composition and patterns of spatial self-organization in a fluctuating environment. ISME J. 2022;16(5):1453-1463. doi: 10.1038/s41396-022-01189-9
- Vasiljevs S, Gupta A, Baines D. Effect of glucose on growth and co-culture of Staphylococcus aureus and Pseudomonas aeruginosa in artificial sputum medium. Heliyon. 2023;9(11):e21469. doi: 10.1016/j.heliyon.2023.e21469
- Immohr MB, Dos Santos Adrego F, Teichert HL, et al. 3D-bioprinting of aortic valve interstitial cells: impact of hydrogel and printing parameters on cell viability. Biomed Mater. 2022;18(1):015004. doi: 10.1088/1748-605X/ac9f91
- Trucco D, Sharma A, Manferdini C, et al. Modeling and fabrication of silk fibroin-gelatin-based constructs using extrusion-based three-dimensional bioprinting. ACS Biomater Sci Eng. 2021;7(7):3306-3320. doi: 10.1021/acsbiomaterials.1c00410
- Pieri K, Felix BM, Zhang T, Soman P, Henderson JH. Printing parameters of fused filament fabrication affect key properties of four-dimensional printed shape-memory polymers. 3D Print Addit Manuf. 2023;10(2):279-288. doi: 10.1089/3dp.2021.0072
- Kim MH, Lee YW, Jung WK, Oh J, Nam SY. Enhanced rheological behaviors of alginate hydrogels with carrageenan for extrusion-based bioprinting. J Mech Behav Biomed Mater. 2019;98:187-194. doi: 10.1016/j.jmbbm.2019.06.014
- Li X, Li X, Yang J, et al. Living and injectable porous hydrogel microsphere with paracrine activity for cartilage regeneration. Small. 2023;19(17):e2207211. doi: 10.1002/smll.202207211
- Asghar W, El Assal R, Shafiee H, Pitteri S, Paulmurugan R, Demirci U. Engineering cancer microenvironments for in vitro 3-D tumor models. Mater Today (Kidlington). 2015;18(10):539-553. doi: 10.1016/j.mattod.2015.05.002
- Dey M, Kim MH, Dogan M, et al. Chemotherapeutics and CAR-T cell-based immunotherapeutics screening on a 3D bioprinted vascularized breast tumor model. Adv Funct Mater. 2022;32(52):3966. doi: 10.1002/adfm.202203966
- Langer EM, Allen-Petersen BL, King SM, et al. Modeling tumor phenotypes in vitro with three-dimensional bioprinting. Cell Rep. 2019;26(3):608-623 e6. doi: 10.1016/j.celrep.2018.12.090
- Stoth M, Mineif AT, Sauer F, et al. A tissue engineered 3D model of cancer cell invasion for human head and neck squamous-cell carcinoma. Curr Issues Mol Biol. 2024;46(5):4049-4062. doi: 10.3390/cimb46050250
- Atat OE, Farzaneh Z, Pourhamzeh M, et al. 3D modeling in cancer studies. Hum Cell. 2022;35(1):23-36. doi: 10.1007/s13577-021-00642-9
- Hong Q, Ding S, Xing C, Mu Z. Advances in tumor immune microenvironment of head and neck squamous cell carcinoma: a review of literature. Medicine (Baltimore). 2024;103(9):e37387. doi: 10.1097/MD.0000000000037387
- Chen SMY, Krinsky AL, Woolaver RA, Wang X, Chen Z, Wang JH. Tumor immune microenvironment in head and neck cancers. Mol Carcinog. 2020;59(7):766-774. doi: 10.1002/mc.23162
- Ruffin AT, Li H, Vujanovic L, Zandberg DP, Ferris RL, Bruno TC. Improving head and neck cancer therapies by immunomodulation of the tumour microenvironment. Nat Rev Cancer. 2023;23(3):173-188. doi: 10.1038/s41568-022-00531-9
- El Herch I, Tornaas S, Dongre HN, Costea DE. Heterogeneity of cancer-associated fibroblasts and tumor-promoting roles in head and neck squamous cell carcinoma. Front Mol Biosci. 2024;11:1340024. doi: 10.3389/fmolb.2024.1340024
- Wang HC, Chan LP, Cho SF. Targeting the immune microenvironment in the treatment of head and neck squamous cell carcinoma. Front Oncol. 2019;9:1084. doi: 10.3389/fonc.2019.01084
- Du W, Xia X, Hu F, Yu J. Extracellular matrix remodeling in the tumor immunity. Front Immunol. 2023;14:1340634. doi: 10.3389/fimmu.2023.1340634
- Huang J, Zhang L, Wan D, et al. Extracellular matrix and its therapeutic potential for cancer treatment. Signal Transduct Target Ther. 2021;6(1):153. doi: 10.1038/s41392-021-00544-0
- Jang DG, Sim HJ, Song EK, Kwon T, Park TJ. Extracellular matrixes and neuroinflammation. BMB Rep. 2020;53(10):491-499. doi: 10.5483/BMBRep.2020.53.10.156
- Azhakesan A, Kern J, Mishra A, et al. 3D bioprinted head and neck squamous cell carcinoma (HNSCC) model using tunicate derived nanocellulose (NC) bioink. Adv Healthc Mater. 2025:e2403114. doi: 10.1002/adhm.202403114
- Gugulothu SB, Asthana S, Homer-Vanniasinkam S, Chatterjee K. Trends in photopolymerizable bioinks for 3D bioprinting of tumor models. JACS Au. 2023;3(8): 2086-2106. doi: 10.1021/jacsau.3c00281
- Mironi-Harpaz I, Wang DY, Venkatraman S, Seliktar D. Photopolymerization of cell-encapsulating hydrogels: crosslinking efficiency versus cytotoxicity. Acta Biomater. 2012;8(5):1838-1848. doi: 10.1016/j.actbio.2011.12.034
- Petta D, Armiento AR, Grijpma D, Alini M, Eglin D, D‘Este M. 3D bioprinting of a hyaluronan bioink through enzymatic-and visible light-crosslinking. Biofabrication. 2018;10(4):044104. doi: 10.1088/1758-5090/aadf58
- Czekay RP, Cheon DJ, Samarakoon R, Kutz SM, Higgins PJ. Cancer-associated fibroblasts: mechanisms of tumor progression and novel therapeutic targets. Cancers (Basel). 2022;14(5):1231. doi: 10.3390/cancers14051231
- Wang X, Wang X, Li J, et al. PDPN contributes to constructing immunosuppressive microenvironment in IDH wildtype glioma. Cancer Gene Ther. 2023;30(2):345-357. doi: 10.1038/s41417-022-00550-6
- Kumar D, New J, Vishwakarma V, et al. Cancer-associated fibroblasts drive glycolysis in a targetable signaling loop implicated in head and neck squamous cell carcinoma progression. Cancer Res. 2018;78(14):3769-3782. doi: 10.1158/0008-5472.CAN-17-1076
- Dean T, Li NT, Cadavid JL, Ailles L, McGuigan AP. A TRACER culture invasion assay to probe the impact of cancer associated fibroblasts on head and neck squamous cell carcinoma cell invasiveness. Biomater Sci. 2020;8(11): 3078-3094. doi: 10.1039/c9bm02017a
- Armingol E, Officer A, Harismendy O, Lewis NE. Deciphering cell-cell interactions and communication from gene expression. Nat Rev Genet. 2021;22(2):71-88. doi: 10.1038/s41576-020-00292-x
- Lammert A, Affolter A, Gvaramia D, et al. [The tumor stem cell niche of head and neck - point of intersection with therapeutic potential?]. Laryngorhinootologie. 2021;100(1):23-29. Die Tumorstammzellnische im Kopf-Hals-Bereich - Knotenpunkt mit therapeutischem Potenzial? doi: 10.1055/a-1260-3054
- Augustine R, Kalva SN, Ahmad R, et al. 3D bioprinted cancer models: revolutionizing personalized cancer therapy. Transl Oncol. 2021;14(4):101015. doi: 10.1016/j.tranon.2021.101015
- Ganguly D, Chandra R, Karalis J, et al. Cancer-associated fibroblasts: versatile players in the tumor microenvironment. Cancers (Basel). 2020;12(9):2652. doi: 10.3390/cancers12092652
- Chandler EM, Seo BR, Califano JP, et al. Implanted adipose progenitor cells as physicochemical regulators of breast cancer. Proc Natl Acad Sci U S A. 2012;109(25):9786-9791. doi: 10.1073/pnas.1121160109
- Raz Y, Erez N. An inflammatory vicious cycle: fibroblasts and immune cell recruitment in cancer. Exp Cell Res. 2013;319(11):1596-603. doi: 10.1016/j.yexcr.2013.03.022
- Tanaka M, Siemann DW. Gas6/Axl signaling pathway in the tumor immune microenvironment. Cancers (Basel). 2020;12(7):1850. doi: 10.3390/cancers12071850
- Baker AT, Abuwarwar MH, Poly L, Wilkins S, Fletcher AL. Cancer-associated fibroblasts and T cells: from mechanisms to outcomes. J Immunol. 2021;206(2):310-320. doi: 10.4049/jimmunol.2001203
- Alkema NG, Wisman GB, van der Zee AG, van Vugt MA, de Jong S. Studying platinum sensitivity and resistance in high-grade serous ovarian cancer: different models for different questions. Drug Resist Updat. 2016;24:55-69. doi: 10.1016/j.drup.2015.11.005
- Alemany-Ribes M, Semino CE. Bioengineering 3D environments for cancer models. Adv Drug Deliv Rev. 2014;79–80:40-49. doi: 10.1016/j.addr.2014.06.004
- Carvalho MR, Lima D, Reis RL, Correlo VM, Oliveira JM. Evaluating biomaterial- and microfluidic-based 3D tumor models. Trends Biotechnol. 2015;33(11):667-678. doi: 10.1016/j.tibtech.2015.09.009
- Ostapowicz J, Ostrowska K, Golusinski W, Kulcenty K, Suchorska WM. Improving therapeutic strategies for head and neck cancer: insights from 3D hypoxic cell culture models in treatment response evaluation. Adv Med Sci. 2024;69(2):368-376. doi: 10.1016/j.advms.2024.07.007
- Torre-Castro J, Rios-Vinuela E, Balaguer-Franch I, et al. Perineural infiltration: a comprehensive review of diagnostic, prognostic, and therapeutic implications. Am J Dermatopathol. 2024;46(5):271-286. doi: 10.1097/DAD.0000000000002667
- Jafari Nivlouei S, Soltani M, Carvalho J, Travasso R, Salimpour MR, Shirani E. Multiscale modeling of tumor growth and angiogenesis: evaluation of tumor-targeted therapy. PLoS Comput Biol. 2021;17(6):e1009081. doi: 10.1371/journal.pcbi.1009081
- Nan J, Roychowdhury S, Randles A. Investigating the influence of heterogeneity within cell types on microvessel network transport. Cell Mol Bioeng. 2023;16(5-6):497-507. doi: 10.1007/s12195-023-00790-y
- Cuenca MB, Canedo L, Perez-Castro C, Grecco HE. An integrative and modular framework to recapitulate emergent behavior in cell migration. Front Cell Dev Biol. 2020;8:615759. doi: 10.3389/fcell.2020.615759
- Park JY, Choi JC, Shim JH, et al. A comparative study on collagen type I and hyaluronic acid dependent cell behavior for osteochondral tissue bioprinting. Biofabrication. 2014;6(3):035004. doi: 10.1088/1758-5082/6/3/035004
- Fischetti T, Di Pompo G, Baldini N, Avnet S, Graziani G. 3D printing and bioprinting to model bone cancer: the role of materials and nanoscale cues in directing cell behavior. Cancers (Basel). 2021;13(16):4065. doi: 10.3390/cancers13164065
- Mojena-Medina D, Martinez-Hernandez M, de la Fuente M, et al. Design, implementation, and validation of a piezoelectric device to study the effects of dynamic mechanical stimulation on cell proliferation, migration and morphology. Sensors (Basel). 2020;20(7):2155. doi: 10.3390/s20072155
- Hong S, Song SJ, Lee JY, et al. Cellular behavior in micropatterned hydrogels by bioprinting system depended on the cell types and cellular interaction. J Biosci Bioeng. 2013;116(2):224-230. doi: 10.1016/j.jbiosc.2013.02.011
- Liu N, Liang W, Liu L, et al. Extracellular-controlled breast cancer cell formation and growth using non-UV patterned hydrogels via optically-induced electrokinetics. Lab Chip. 2014;14(7):1367-1376. doi: 10.1039/c3lc51247a
- Petreus T, Cadogan E, Hughes G, et al. Tumour-on-chip microfluidic platform for assessment of drug pharmacokinetics and treatment response. Commun Biol. 2021;4(1):1001. doi: 10.1038/s42003-021-02526-y
- Ruchika, Bhardwaj N, Yadav SK, Saneja A. Recent advances in 3D bioprinting for cancer research: From precision models to personalized therapies. Drug Discov Today. 2024;29(4):103924. doi: 10.1016/j.drudis.2024.103924
- Chen H, Wu Z, Gong Z, et al. Acoustic bioprinting of patient-derived organoids for predicting cancer therapy responses. Adv Healthc Mater. 2022;11(13):e2102784. doi: 10.1002/adhm.202102784
- Park A, Lee Y, Nam S. A performance evaluation of drug response prediction models for individual drugs. Sci Rep. 2023;13(1):11911. doi: 10.1038/s41598-023-39179-2
- Shukla P, Bera AK, Yeleswarapu S, Pati F. High throughput bioprinting using decellularized adipose tissue-based hydrogels for 3D breast cancer modeling. Macromol Biosci. 2024;24(8):e2400035. doi: 10.1002/mabi.202400035
- Yi HG, Kim H, Kwon J, Choi YJ, Jang J, Cho DW. Application of 3D bioprinting in the prevention and the therapy for human diseases. Signal Transduct Target Ther. 2021;6(1):177. doi: 10.1038/s41392-021-00566-8
- Driehuis E, Kolders S, Spelier S, et al. Oral mucosal organoids as a potential platform for personalized cancer therapy. Cancer Discov. 2019;9(7):852-871. doi: 10.1158/2159-8290.CD-18-1522
- Yang K, Wang L, Vijayavenkataraman S, Yuan Y, Tan ECK, Kang L. Recent applications of three-dimensional bioprinting in drug discovery and development. Adv Drug Deliv Rev. 2024;214:115456. doi: 10.1016/j.addr.2024.115456
- Smith AD, Roda D, Yap TA. Strategies for modern biomarker and drug development in oncology. J Hematol Oncol. 2014;7:70. doi: 10.1186/s13045-014-0070-8
- Tate KM, Munson JM. Assessing drug response in engineered brain microenvironments. Brain Res Bull. 2019;150:21-34. doi: 10.1016/j.brainresbull.2019.04.027
- Morikawa A, Li J, Ulintz P, et al. Optimizing precision medicine for breast cancer brain metastases with functional drug response assessment. Cancer Res Commun. 2023;3(6):1093-1103. doi: 10.1158/2767-9764.CRC-22-0492
- Norkin M, Ordonez-Moran P, Huelsken J. High-content, targeted RNA-seq screening in organoids for drug discovery in colorectal cancer. Cell Rep. 2021;35(3):109026. doi: 10.1016/j.celrep.2021.109026
- Lim SH, Kathuria H, Tan JJY, Kang L. 3D printed drug delivery and testing systems - a passing fad or the future? Adv Drug Deliv Rev. 2018;132:139-168. doi: 10.1016/j.addr.2018.05.006
- Day D, Siu LL. Approaches to modernize the combination drug development paradigm. Genome Med. 2016; 8(1):115. doi: 10.1186/s13073-016-0369-x
- Karavasili C, Eleftheriadis GK, Gioumouxouzis C, Andriotis EG, Fatouros DG. Mucosal drug delivery and 3D printing technologies: a focus on special patient populations. Adv Drug Deliv Rev. 2021;176:113858. doi: 10.1016/j.addr.2021.113858
- Sandler N, Preis M. Printed drug-delivery systems for improved patient treatment. Trends Pharmacol Sci. 2016;37(12):1070-1080. doi: 10.1016/j.tips.2016.10.002
- Soman SS, Vijayavenkataraman S. Applications of 3D bioprinted-induced pluripotent stem cells in healthcare. Int J Bioprint. 2020;6(4):280. doi: 10.18063/ijb.v6i4.280
- Frohlich H, Bontridder N, Petrovska-Delacreta D, et al. Leveraging the potential of digital technology for better individualized treatment of Parkinson‘s disease. Front Neurol. 2022;13:788427. doi: 10.3389/fneur.2022.788427
- Zhao W, Hu C, Xu T. In vivo bioprinting: broadening the therapeutic horizon for tissue injuries. Bioact Mater. 2023;25:201-222. doi: 10.1016/j.bioactmat.2023.01.018
- Sekar MP, Budharaju H, Zennifer A, et al. Current standards and ethical landscape of engineered tissues-3D bioprinting perspective. J Tissue Eng. 2021;12:20417314211027677. doi: 10.1177/20417314211027677
- Zou W, McAdorey A, Yan H, Chen W. Nanomedicine to overcome antimicrobial resistance: challenges and prospects. Nanomedicine (Lond). 2023;18(5): 471-484. doi: 10.2217/nnm-2023-0022
- Li J, Wang H. Selective organ targeting nanoparticles: from design to clinical translation. Nanoscale Horiz. 2023;8(9):1155-1173. doi: 10.1039/d3nh00145h
- Younis MA, Tawfeek HM, Abdellatif AAH, Abdel-Aleem JA, Harashima H. Clinical translation of nanomedicines: challenges, opportunities, and keys. Adv Drug Deliv Rev. 2022;181:114083. doi: 10.1016/j.addr.2021.114083
- Bettinger CJ, Ecker M, Kozai TDY, Malliaras GG, Meng E, Voit W. Recent advances in neural interfaces-Materials chemistry to clinical translation. MRS Bull. 2020;45(8): 655-668. doi: 10.1557/mrs.2020.195
- Jaskulski S, Nuszbaum C, Michels KB. Components, prospects and challenges of personalized prevention. Front Public Health. 2023;11:1075076. doi: 10.3389/fpubh.2023.1075076
- Assefa D, Melaku T, Alemu S. Commentary: nanoparticle-based chemotherapy delivery and potential health risks: prospects for effective clinical translation. Technol Cancer Res Treat. 2023;22:15330338231220171. doi: 10.1177/15330338231220171
- Chen XB, Fazel Anvari-Yazdi A, Duan X, et al. Biomaterials/ bioinks and extrusion bioprinting. Bioact Mater. 2023;28:511-536. doi: 10.1016/j.bioactmat.2023.06.006
- Kiyotake EA, Douglas AW, Thomas EE, Nimmo SL, Detamore MS. Development and quantitative characterization of the precursor rheology of hyaluronic acid hydrogels for bioprinting. Acta Biomater. 2019;95:176-187. doi: 10.1016/j.actbio.2019.01.041
- Kim YB, Lee H, Kim GH. Strategy to achieve highly porous/biocompatible macroscale cell blocks, using a collagen/genipin-bioink and an optimal 3D printing process. ACS Appl Mater Interfaces. 2016;8(47): 32230-32240. doi: 10.1021/acsami.6b11669
- Jumaniyazova E, Lokhonina A, Dzhalilova D, Kosyreva A, Fatkhudinov T. Role of microenvironmental components in head and neck squamous cell carcinoma. J Pers Med. 2023;13(11):1616. doi: 10.3390/jpm13111616
- Lopez de Andres J, Rodriguez-Santana C, de Lara-Pena L, Jimenez G, Escames G, Marchal JA. A bioengineered tumor matrix-based scaffold for the evaluation of melatonin efficacy on head and neck squamous cancer stem cells. Mater Today Bio. 2024;29:101246. doi: 10.1016/j.mtbio.2024.101246
- Zhou J, Liu C, Amornphimoltham P, et al. Mouse models for head and neck squamous cell carcinoma. J Dent Res. 2024;103(6):585-595. doi: 10.1177/00220345241240997
- Knops AM, South A, Rodeck U, et al. Cancer-associated fibroblast density, prognostic characteristics, and recurrence in head and neck squamous cell carcinoma: a meta-analysis. Front Oncol. 2020;10:565306. doi: 10.3389/fonc.2020.565306
- Piluso S, Skvortsov GA, Altunbek M, et al. 3D bioprinting of molecularly engineered PEG-based hydrogels utilizing gelatin fragments. Biofabrication. 2021;13(4):045008. doi: 10.1088/1758-5090/ac0ff0
- Cai Y, Chang SY, Gan SW, Ma S, Lu WF, Yen CC. Nanocomposite bioinks for 3D bioprinting. Acta Biomater. 2022;151:45-69. doi: 10.1016/j.actbio.2022.08.014
- Najafi M, Mortezaee K, Majidpoor J. Cancer stem cell (CSC) resistance drivers. Life Sci. 2019;234:116781. doi: 10.1016/j.lfs.2019.116781
- Bhat AA, Yousuf P, Wani NA, et al. Tumor microenvironment: an evil nexus promoting aggressive head and neck squamous cell carcinoma and avenue for targeted therapy. Signal Transduct Target Ther. 2021;6(1):12. doi: 10.1038/s41392-020-00419-w
- Dai X, Ma C, Lan Q, Xu T. 3D bioprinted glioma stem cells for brain tumor model and applications of drug susceptibility. Biofabrication. 2016;8(4):045005. doi: 10.1088/1758-5090/8/4/045005
- You S, Xiang Y, Hwang HH, et al. High cell density and high-resolution 3D bioprinting for fabricating vascularized tissues. Sci Adv. 2023;9(8):eade7923. doi: 10.1126/sciadv.ade7923
- Lee S, Sani ES, Spencer AR, Guan Y, Weiss AS, Annabi N. Human-recombinant-elastin-based bioinks for 3d bioprinting of vascularized soft tissues. Adv Mater. 2020;32(45):e2003915. doi: 10.1002/adma.202003915
- Zhang Z, Wu C, Dai C, et al. A multi-axis robot-based bioprinting system supporting natural cell function preservation and cardiac tissue fabrication. Bioact Mater. 2022;18:138-150. doi: 10.1016/j.bioactmat.2022.02.009
- Ali ASM, Wu D, Bannach-Brown A, et al. 3D bioprinting of liver models: a systematic scoping review of methods, bioinks, and reporting quality. Mater Today Bio. 2024;26:100991. doi: 10.1016/j.mtbio.2024.100991
- Chimene D, Miller L, Cross LM, Jaiswal MK, Singh I, Gaharwar AK. Nanoengineered osteoinductive bioink for 3D bioprinting bone tissue. ACS Appl Mater Interfaces. 2020;12(14):15976-15988. doi: 10.1021/acsami.9b19037
- Jiu J, Liu H, Li D, et al. 3D bioprinting approaches for spinal cord injury repair. Biofabrication. 2024;16(3):032003. doi: 10.1088/1758-5090/ad3a13
- Sheth RA, Wehrenberg-Klee E, Patel SP, Brock KK, Fotiadis N, de Baere T. Intratumoral injection of immunotherapeutics: state of the art and future directions. Radiology. 2024;312(1):e232654. doi: 10.1148/radiol.232654
- Mazzaglia C, Sheng Y, Rodrigues LN, Lei IM, Shields JD, Huang YYS. Deployable extrusion bioprinting of compartmental tumoroids with cancer associated fibroblasts for immune cell interactions. Biofabrication. 2023;15(2):025005. doi: 10.1088/1758-5090/acb1db
- Zhang H, Dong S, Li Z, et al. Biointerface engineering nanoplatforms for cancer-targeted drug delivery. Asian J Pharm Sci. 2020;15(4):397-415. doi: 10.1016/j.ajps.2019.11.004
- Yang Y, Qiao X, Huang R, et al. E-jet 3D printed drug delivery implants to inhibit growth and metastasis of orthotopic breast cancer. Biomaterials. 2020;230:119618. doi: 10.1016/j.biomaterials.2019.119618
- Beg S, Almalki WH, Malik A, et al. 3D printing for drug delivery and biomedical applications. Drug Discov Today. 2020;25(9):1668-1681. doi: 10.1016/j.drudis.2020.07.007
- Ravnic DJ, Leberfinger AN, Koduru SV, et al. Transplantation of bioprinted tissues and organs: technical and clinical challenges and future perspectives. Ann Surg. 2017;266(1):48-58. doi: 10.1097/SLA.0000000000002141