Ink comparison for extrusion-based bioprinting in the context of breast cancer and melanoma models
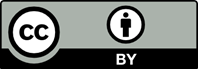
Biofabrication has proven to be a versatile and valuable tool for tissue engineering and cancer research, enabling the mimicry of various tumor microenvironments. In the present study, four different cell lines, including two melanoma cell lines, Mel Im and Mel Wei, and two breast cancer cell lines, MDA-MB-231 and MCF-7, were tested in combination with four distinct hydrogels. The hydrogels used were a composite ink of alginate, hyaluronic acid, and gelatin (Alg/HA/Gel), pre-crosslinked oxidized alginate with gelatin (ADA-GEL), alginate with methylcellulose (Meth-Alg), and acrylated hyaluronic acid (HAA). Rheological analysis, shear stress calculations, printability assays, and dynamic mechanical analysis were performed on all hydrogels. The cell lines were then mixed into the hydrogels, printed, and examined over 14 days, with a focus on cell survival, metabolic activity, and cell cycle analysis using fluorescent ubiquitination-based cell cycle indicator reporters. The results showed that all hydrogels were printable, with Meth-Alg being the softest gel. The cell lines survived the printing process in all bioinks, but there were significant differences in the metabolic activity and the predominant cell cycle stage. In Alg/HA/Gel, the cells grew in spheroid colonies. ADA-GEL proved to be a good bioink, supporting proliferation, migration, and high metabolic activity across all cell lines, while Meth- Alg offered pore structures conducive to cell spreading and proliferation. However, HAA resulted in the lowest cell number and metabolic activity across all cell lines, likely due to its high polymer content, which induced senescence. Our data demonstrated that each of these bioinks presents unique advantages for breast cancer and melanoma research models.

- Bray F, Laversanne M, Sung H, et al. Global cancer statistics 2022: GLOBOCAN estimates of incidence and mortality worldwide for 36 cancers in 185 countries. CA Cancer J Clin. 2024;74(3):229-263. doi: 10.3322/caac.21834
- Schwartz SM. Epidemiology of cancer. Clin Chem. 2024;70(1):140-149. doi: 10.1093/clinchem/hvad202
- Arnold M, Singh D, Laversanne M, et al. Global burden of cutaneous melanoma in 2020 and projections to 2040. JAMA Dermatol. 2022;158(5):495-503. doi: 10.1001/jamadermatol.2022.0160
- Wilkinson L, Gathani T. Understanding breast cancer as a global health concern. Br J Radiol. 2022;95(1130): 20211033. doi: 10.1259/bjr.20211033
- Gerstberger S, Jiang Q, Ganesh K. Metastasis. Cell. 2023;186(8):1564-1579. doi: 10.1016/j.cell.2023.03.003
- Groll J, Burdick JA, Cho DW, et al. A definition of bioinks and their distinction from biomaterial inks. Biofabrication. 2019;11(1):013001. doi: 10.1088/1758-5090/aaec52
- Safari Z, Soudi S, Jafarzadeh N, Hosseini AZ, Vojoudi E, Sadeghizadeh M. Promotion of angiogenesis by M13 phage and RGD peptide in vitro and in vivo. Sci Rep. 2019;9(1):11182. doi: 10.1038/s41598-019-47413-z
- Grigore A, Sarker B, Fabry B, Boccaccini AR, Detsch R. Behavior of encapsulated MG-63 cells in RGD and gelatine-modified alginate hydrogels. Tissue Eng Part A. 2014;20(15-16):2140-2150. doi: 10.1089/ten.tea.2013.0416
- Toole BP. Hyaluronan: from extracellular glue to pericellular cue. Nat Rev Cancer. 2004;4(7):528-539. doi: 10.1038/nrc1391
- Viktor Z, Farcet C, Moire C, Brothier F, Pfukwa H, Pasch H. Comprehensive two-dimensional liquid chromatography for the characterization of acrylate-modified hyaluronic acid. Anal Bioanal Chem. 2019;411(15):3321-3330. doi: 10.1007/s00216-019-01799-x
- Horder H, Böhringer D, Endrizzi N, et al. Cancer cell migration depends on adjacent ASC and adipose spheroids in a 3D bioprinted breast cancer model. Biofabrication. 2024;16(3). doi: 10.1088/1758-5090/ad57f7
- Rodríguez-Pérez E, Lloret Compañ A, Monleón Pradas M, Martínez-Ramos C. Scaffolds of hyaluronic acid–poly(ethyl acrylate) interpenetrating networks: characterization and in vitro studies. Macromol Biosci. 2016;16(8):1147-1157. doi: 10.1002/mabi.201600028
- Sarker B, Rompf J, Silva R, et al. Alginate-based hydrogels with improved adhesive properties for cell encapsulation. Int J Biol Macromol. 2015;78:72-78. doi: 10.1016/j.ijbiomac.2015.03.061
- Gunes OC, Ziylan Albayrak A. Antibacterial polypeptide nisin containing cotton modified hydrogel composite wound dressings. Polymer Bull. 2021;78(11):6409-6428. doi: 10.1007/s00289-020-03429-4
- Kim WS, Mooney DJ, Arany PR, Lee K, Huebsch N, Kim J. Adipose tissue engineering using injectable, oxidized alginate hydrogels. Tissue Eng Part A. 2012;18(7-8):737-743. doi: 10.1089/ten.TEA.2011.0250
- Jejurikar A, Seow XT, Lawrie G, Martin D, Jayakrishnan A, Grøndahl L. Degradable alginate hydrogels crosslinked by the macromolecular crosslinker alginate dialdehyde. J Mater Chem. 2012;22(19):9751-9758. doi: 10.1039/C2JM30564J
- Liao H, Zhang H, Chen W. Differential physical, rheological, and biological properties of rapid in situ gelable hydrogels composed of oxidized alginate and gelatin derived from marine or porcine sources. J Mater Sci Mater Med. 2009;20(6):1263-1271. doi: 10.1007/s10856-009-3694-4
- Sarker B, Singh R, Silva R, et al. Evaluation of fibroblasts adhesion and proliferation on alginate-gelatin crosslinked hydrogel. PLoS One. 2014;9(9):e107952. doi: 10.1371/journal.pone.0107952
- Sprenger L, Lu HH, Trippmacher S, et al. Composite alginate dialdehyde-gelatin (ADA-GEL) hydrogel containing short ribbon-shaped fillers for skeletal muscle tissue biofabrication. ACS Appl Mater Interfaces. 2024;16(34):44605-44622. doi: 10.1021/acsami.4c10751
- Ruther F, Distler T, Boccaccini AR, Detsch R. Biofabrication of vessel-like structures with alginate di-aldehyde-gelatin (ADA-GEL) bioink. J Mater Sci Mater Med. 2018; 30(1):8. doi: 10.1007/s10856-018-6205-7
- Steiner D, Lingens L, Fischer L, et al. Encapsulation of mesenchymal stem cells improves vascularization of alginate-based scaffolds. Tissue Eng Part A. 2018;24(17-18):1320-1331. doi: 10.1089/ten.TEA.2017.0496
- Schulik J, Salehi S, Boccaccini AR, et al. Comparison of the behavior of 3D-printed endothelial cells in different bioinks. Bioengineering (Basel). 2023;10(7):751. doi: 10.3390/bioengineering10070751
- Schütz K, Placht A-M, Paul B, Brüggemeier S, Gelinsky M, Lode A. Three-dimensional plotting of a cell-laden alginate/methylcellulose blend: towards biofabrication of tissue engineering constructs with clinically relevant dimensions. J Tissue Eng Regen Med. 2017;11(5):1574-1587. doi: https://doi.org/10.1002/term.2058
- Bychkov AL, Podgorbunskikh EM, Ryabchikova EI, Lomovsky OI. The role of mechanical action in the process of the thermomechanical isolation of lignin. Cellulose. 2018;25(1):1-5. doi: 10.1007/s10570-017-1536-y
- Sarma NJ, Takeda A, Yaseen NR. Colony forming cell (CFC) assay for human hematopoietic cells. J Vis Exp. 2010;(46):2195. doi: 10.3791/2195
- Hodder E, Duin S, Kilian D, et al. Investigating the effect of sterilisation methods on the physical properties and cytocompatibility of methyl cellulose used in combination with alginate for 3D-bioplotting of chondrocytes. J Mater Sci Mater Med. 2019;30(1):10. doi: 10.1007/s10856-018-6211-9
- Schmid R, Schmidt SK, Detsch R, et al. A new printable alginate/hyaluronic acid/gelatin hydrogel suitable for biofabrication of in vitro and in vivo metastatic melanoma models. Adv Funct Mater. 2022;32(2):2107993. doi: 10.1002/adfm.202107993
- Johnson JP, Demmer-Dieckmann M, Meo T, Hadam MR, Riethmuller G. Surface antigens of human melanoma cells defined by monoclonal antibodies. I. Biochemical characterization of two antigens found on cell lines and fresh tumors of diverse tissue origin. Eur J Immunol. 1981;11(10):825-831. doi: 10.1002/eji.1830111015
- Sarker B, Papageorgiou DG, Silva R, et al. Fabrication of alginate–gelatin crosslinked hydrogel microcapsules and evaluation of the microstructure and physico-chemical properties. J Mater Chem B. 2014;2(11):1470. doi: 10.1039/c3tb21509a
- Hazur J, Röder J, Czwalinna J, Schubert DW, Boccaccini AR. Pre‐crosslinking with hydrogel microparticles enhances the printability of alginate‐based inks. Macromol Mater Eng. 2023;308(12):202200675 doi: 10.1002/mame.202200675
- Schmid R, Schmidt SK, Schrüfer S, et al. A vascularized in vivo melanoma model suitable for metastasis research of different tumor stages using fundamentally different bioinks. Mater Today Bio. 2024;26:101071. doi: 10.1016/j.mtbio.2024.101071
- Schmid R, Schmidt SK, Hazur J, et al. Comparison of hydrogels for the development of well-defined 3D cancer models of breast cancer and melanoma. Cancers. 2020;12(8):2320. doi: 10.3390/cancers12082320
- Koh SB, Mascalchi P, Rodriguez E, et al. A quantitative FastFUCCI assay defines cell cycle dynamics at a single-cell level. J Cell Sci. 2017;130(2):512-520. doi: 10.1242/jcs.195164
- Hazur J, Detsch R, Karakaya E, et al. Improving alginate printability for biofabrication: establishment of a universal and homogeneous pre-crosslinking technique. Biofabrication. 2020;12(4):045004. doi: 10.1088/1758-5090/ab98e5
- Ahlfeld T, Guduric V, Duin S, et al. Methylcellulose - a versatile printing material that enables biofabrication of tissue equivalents with high shape fidelity. Biomater Sci. 2020;8(8):2102-2110. doi: 10.1039/d0bm00027b
- Schipka R, Heltmann-Meyer S, Schneidereit D, et al. Characterization of two different alginate-based bioinks and the influence of melanoma growth within. Sci Rep. 2024; 14(1):12945. doi: 10.1038/s41598-024-63642-3
- Heid S, Becker K, Byun J, et al. Bioprinting with bioactive alginate dialdehyde-gelatin (ADA-GEL) composite bioinks: time-dependent in-situ crosslinking via addition of calcium-silicate particles tunes in vitro stability of 3D bioprinted constructs. Bioprinting. 2022;26:e00200. doi: 10.1016/j.bprint.2022.e00200
- Lei IM, Zhang D, Gu W, Liu J, Zi Y, Huang YYS. Soft hydrogel shapeability via supportive bath matching in embedded 3D printing. Adv Mater Technol. 2023;8(15):2300001. doi: 10.1002/admt.202300001
- Chand R, Muhire BS, Vijayavenkataraman S. Computational fluid dynamics assessment of the effect of bioprinting parameters in extrusion bioprinting. Int J Bioprint. 2022;8(2):545. doi: 10.18063/ijb.v8i2.545
- Müller SJ, Fabry B, Gekle S. Predicting cell stress and strain during extrusion bioprinting. Phys Rev Appl. 2023;19(6):064061. doi: 10.1103/PhysRevApplied.19.064061
- Brunel LG, Christakopoulos F, Kilian D, et al. Embedded 3D bioprinting of collagen inks into microgel baths to control hydrogel microstructure and cell spreading. Adv Healthc Mater. 2024;13(25):e2303325. doi: 10.1002/adhm.202303325
- Gonzalez-Fernandez T, Rathan S, Hobbs C, et al. Pore-forming bioinks to enable spatio-temporally defined gene delivery in bioprinted tissues. J Control Release. 2019;301:13-27. doi: 10.1016/j.jconrel.2019.03.006
- Truskowski K, Amend SR, Pienta KJ. Dormant cancer cells: programmed quiescence, senescence, or both? Cancer Metastasis Rev. 2023;42(1):37-47. doi: 10.1007/s10555-022-10073-z
- Basu S, Dong Y, Kumar R, Jeter C, Tang DG. Slow-cycling (dormant) cancer cells in therapy resistance, cancer relapse and metastasis. Semin Cancer Biol. 2022;78:90-103. doi: 10.1016/j.semcancer.2021.04.021
- Chen X, Du Y, Liu Y, et al. Hyaluronan arrests human breast cancer cell growth by prolonging the G0/G1 phase of the cell cycle. Acta Biochim Biophys Sin (Shanghai). 2018;50(12):1181-1189. doi: 10.1093/abbs/gmy126
- Hazur J, Endrizzi N, Schubert DW, Boccaccini AR, Fabry B. Stress relaxation amplitude of hydrogels determines migration, proliferation, and morphology of cells in 3-D culture. Biomater Sci. 2021;10(1):270-280. doi: 10.1039/d1bm01089a
- Souza AG, Silva IBB, Campos-Fernandez E, et al. Comparative assay of 2D and 3D cell culture models: proliferation, gene expression and anticancer drug response. Curr Pharm Des. 2018;24(15):1689-1694. doi: 10.2174/1381612824666180404152304
- Menon N, Dang HX, Datla US, et al. Heparin-based hydrogel scaffolding alters the transcriptomic profile and increases the chemoresistance of MDA-MB-231 triple-negative breast cancer cells. Biomater Sci. 2020;8(10):2786-2796. doi: 10.1039/c9bm01481k
- Kim BJ, Zhao S, Bunaciu RP, Yen A, Wu M. A 3D in situ cell counter reveals that breast tumor cell (MDA-MB-231) proliferation rate is reduced by the collagen matrix density. Biotechnol Prog. 2015;31(4):990-996. doi: 10.1002/btpr.2062
- Azimi T, Loizidou M, Dwek MV. Cancer cells grown in 3D under fluid flow exhibit an aggressive phenotype and reduced responsiveness to the anti-cancer treatment doxorubicin. Sci Rep. 2020;10(1):12020. doi: 10.1038/s41598-020-68999-9
- Elia I, Haigis MC. Metabolites and the tumour microenvironment: from cellular mechanisms to systemic metabolism. Nat Metab. 2021;3(1):21-32. doi: 10.1038/s42255-020-00317-z
- Schaefer N, Andrade Mier MS, Sonnleitner D, et al. Rheological and biological impact of printable PCL-fibers as reinforcing fillers in cell-laden spider-silk bio-inks. Small Methods. 2023;7(10):e2201717. doi: 10.1002/smtd.202201717