Optimizing printability and mechanical properties of poly(3-hydroxybutyrate) (P3HB) biocomposite blends and their biological response to Saos-2 cells
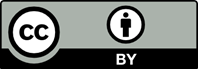
Bone tissue engineering requires scaffolds with three-dimensional structures that facilitate vascularization and new tissue growth. 3D printing, especially through fused deposition modeling (FDM), has emerged as an effective method for creating complex structures with high reproducibility. Early research in this area demonstrated the potential of poly(ε-caprolactone) (PCL) and poly(L-lactide) (PLLA) scaffolds for bone regeneration. Recently, polylactide (PLA) and polyhydroxyalkanoates (PHAs) have garnered attention for their biocompatibility and ability to support cell proliferation. Among PHAs, poly(3-hydroxybutyrate) (P3HB) shows promise due to its intrinsic biocompatibility and resorbability, making it a candidate for FDM-based scaffold fabrication. In the presented study, we aim to develop and optimize a biocompatible P3HB-based composite material for bone tissue engineering, incorporating PLA, hydroxyapatite (HA), and the plasticizer Syncroflex3114 (SN) to enhance mechanical properties and printability. This composite was processed into filaments for 3D printing and characterized through thermal, mechanical, and biological evaluations. Using a design of experiment (DoE) approach, we investigated factors such as temperature performance, warping, degradation, and strength to determine the optimal composition for use in tissue engineering. Four optimal mixture compositions fulfilling the optimization criteria of having the most suitable properties for bone tissue engineering, namely the best printability and maximum mechanical properties, were obtained. The mixtures were optimized specifically for minimum warping coefficient (0.5); maximum flexural strength (66.9 MPa); maximum compression modulus (2.4 GPa); and maximum compression modulus (2.3 GPa) with a warping coefficient of no more than one at the same time. In conclusion, the study shows a new possible way to effectively develop and test 3D printed P3HB-based scaffolds with specifically optimized material properties.