Sericin improves alginate-gelatin hydrogels’ mechanical properties, porosity, durability, and viability of fibroblasts in cardiac spheroids
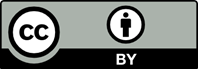
Biofabrication of cardiac patches is a challenging strategy proposed as an alternative to transplantation for end-stage heart failure patients. The optimization of the bioink used for this strategy can be limited by costs, properties, and biocompatibility of its building blocks. Lately, sericin has emerged within a wide range of natural proteins, thanks to its bioadhesive and biocompatibility potential. In this study, we assessed for the first time the effects of adding silk sericin on alginate-gelatin hydrogels, proposed for cardiac applications. To this aim, we first biofabricated sericin-containing hydrogels with increasing protein concentrations. Thus, we characterized hydrogels’ mechanical behavior, porosity and structure through rheology, Brillouin microspectroscopy, and scanning electron microscopy. Then, we bioprinted the formulated hydrogels and evaluated their effects on human cardiac spheroids (CSs) in vitro. Our mechanical characterization demonstrated that adding sericin significantly enhanced the elasticity and the viscosity of alginate-gelatin hydrogels. Sericin also modified hydrogels’ swelling behavior and their pore size, increasing by 20%, 62%, and 92% in Ser1%, Ser2%, and Ser3%, respectively. Although Ser1% did not exhibit significant effects on CSs, Ser2% and Ser3% enhanced cardiac cell viability for up to 14 days compared to the sericin-free hydrogel by acting on the fibroblast population. Sericin-based bioinks showed better printability and durability with +33% and +28% intact patches after 28 days of culture at 37°C compared to alginate-gelatin. Taken together, our results validated the use of sericin as a promising component for the optimization of bioink intended for cardiac applications.

- Tsao CW, Aday AW, Almarzooq ZI, et al. Heart disease and stroke statistics—2023 update: a report from the American Heart Association. Circulation. 2023;147(8):e93-e621. doi: 10.1161/CIR.0000000000001123
- Cooke JP. Is cardiovascular regeneration possible? Methodist Debakey Cardiovasc J. 2023;19(5):1-4. doi: 10.14797/mdcvj.1307
- Frantz S, Hundertmark MJ, Schulz-Menger J, Bengel FM, Bauersachs J. Left ventricular remodelling post-myocardial infarction: pathophysiology, imaging, and novel therapies. Eur Heart J. 2022;43(27):2549-2561. doi: 10.1093/eurheartj/ehac223
- Schwinger RHG. Pathophysiology of heart failure. Cardiovasc Diagn Ther. 2021;11(1):263-276. doi: 10.21037/cdt-20-302
- Awad MA, Shah A, Griffith BP. Current status and outcomes in heart transplantation: a narrative review. Rev Cardiovasc Med. 2022;23(1):11. doi: 10.31083/j.rcm2301011
- Mantha A, Lee RO, Jr., Wolfson AM. Patient selection for heart transplant: balancing risk. Curr Opin Organ Transplant. 2022;27(1):36-44. doi: 10.1097/mot.0000000000000943
- Benck L, Kransdorf EP, Emerson DA, et al. Recipient and surgical factors trigger severe primary graft dysfunction after heart transplant. J Heart Lung Transplant. 2021;40(9):970-980. doi: 10.1016/j.healun.2021.06.002
- Huang K, Ozpinar EW, Su T, et al. An off-the-shelf artificial cardiac patch improves cardiac repair after myocardial infarction in rats and pigs. Sci Transl Med. 2020;12(538):eaat9683. doi: 10.1126/scitranslmed.aat9683
- Wu CA, Zhu Y, Woo YJ. Advances in 3D bioprinting: techniques, applications, and future directions for cardiac tissue engineering. Bioengineering (Basel). 2023; 10(7):842. doi: 10.3390/bioengineering10070842
- Das S, Nam H, Jang J. 3D bioprinting of stem cell-laden cardiac patch: a promising alternative for myocardial repair. APL Bioeng. 2021;5(3):031508. doi: 10.1063/5.0030353
- Budharaju H, Sundaramurthi D, Sethuraman S. Efficient dual crosslinking of protein-in-polysaccharide bioink for biofabrication of cardiac tissue constructs. Biomater Adv. 2023;152:213486. doi: 10.1016/j.bioadv.2023.213486
- Zheng Z, Tang W, Li Y, et al. Advancing cardiac regeneration through 3D bioprinting: methods, applications, and future directions. Heart Fail Rev. 2024;29(3):599-613. doi: 10.1007/s10741-023-10367-6
- Yao Y, Li A, Wang S, et al. Multifunctional elastomer cardiac patches for preventing left ventricle remodeling after myocardial infarction in vivo. Biomaterials. 2022;282: 121382. doi: 10.1016/j.biomaterials.2022.121382
- Garbern JC, Lee RT. Heart regeneration: 20 years of progress and renewed optimism. Dev Cell. 2022;57(4):424-439. doi: 10.1016/j.devcel.2022.01.012
- Liu Chung Ming C, Ben-Sefer E, Gentile C. Stem cell-based 3D bioprinting for cardiovascular tissue regeneration. In: Zhang J, Serpooshan V, eds. Advanced Technologies in Cardiovascular Bioengineering. Springer International Publishing; 2022:281-312. doi: 10.1007/978-3-030-86140-7_13
- Brazhkina O, Park JH, Park HJ, et al. Designing a 3D printing based auxetic cardiac patch with hiPSC-CMs for heart repair. J Cardiovasc Dev Dis. 2021;8(12):172. doi: 10.3390/jcdd8120172
- Matthews N, Pandolfo B, Moses D, Gentile C. Taking it personally: 3D bioprinting a patient-specific cardiac patch for the treatment of heart failure. Bioengineering. 2022;9(3):93. doi: 10.3390/bioengineering9030093
- Budharaju H, Subramanian A, Sethuraman S. Recent advancements in cardiovascular bioprinting and bioprinted cardiac constructs. Biomater Sci. 2021;9(6):1974-1994. doi: 10.1039/D0BM01428A
- Lu TY, Xiang Y, Tang M, Chen S. 3D printing approaches to engineer cardiac tissue. Curr Cardiol Rep. 2023;25(6):505-514. doi: 10.1007/s11886-023-01881-y
- Baheiraei N, Razavi M, Ghahremanzadeh R. Reduced graphene oxide coated alginate scaffolds: potential for cardiac patch application. Biomater Res. 2023; 27(1):109. doi: 10.1186/s40824-023-00449-9
- Fischer B, Gwinner F, Gepp MM, et al. A highly versatile biopolymer-based platform for the maturation of human pluripotent stem cell-derived cardiomyocytes enables functional analysis in vitro and 3D printing of heart patches. J Biomed Mater Res A. 2023;111(10):1600-1615. doi: 10.1002/jbm.a.37558
- Loureiro J, Miguel SP, Galván-Chacón V, et al. Three-dimensionally printed hydrogel cardiac patch for infarct regeneration based on natural polysaccharides. Polymers. 2023;15(13):2824. doi: 10.3390/polym15132824
- Kazemi Asl S, Rahimzadegan M, Ostadrahimi R. The recent advancement in the chitosan hybrid-based scaffolds for cardiac regeneration after myocardial infarction. Carbohydr Polym. 2023;300:120266. doi: 10.1016/j.carbpol.2022.120266
- Sharma P, Gentile C. Cardiac spheroids as in vitro bioengineered heart tissues to study human heart pathophysiology. J Vis Exp. 2021;167(167):33554972. doi: 10.3791/61962
- Polonchuk L, Chabria M, Badi L, et al. Cardiac spheroids as promising in vitro models to study the human heart microenvironment. Sci Rep. 2017;7(1):7005. doi: 10.1038/s41598-017-06385-8
- Sharma P, Liu Chung Ming C, Wang X, et al. Biofabrication of advanced in vitro 3D models to study ischaemic and doxorubicin-induced myocardial damage. Biofabrication. 2022;14(2):109-116. doi: 10.1088/1758-5090/ac47d8
- Sharma P, Wang X, Ming CLC, et al. Considerations for the bioengineering of advanced cardiac in vitro models of myocardial infarction. Small. 2021;17(15):e2003765. doi: 10.1002/smll.202003765
- Roche CD, Lin H, Huang Y, et al. 3D bioprinted alginate-gelatin hydrogel patches containing cardiac spheroids recover heart function in a mouse model of myocardial infarction. Bioprinting. 2023;30:e00263. doi: 10.1016/j.bprint.2023.e00263
- Polonchuk L, Surija L, Lee MH, et al. Towards engineering heart tissues from bioprinted cardiac spheroids. Biofabrication. 2021;13(4):34265755. doi: 10.1088/1758-5090/ac14ca
- Roche CD, Sharma P, Ashton AW, Jackson C, Xue M, Gentile C. Printability, durability, contractility and vascular network formation in 3D bioprinted cardiac endothelial cells using alginate-gelatin hydrogels. Front Bioeng Biotechnol. 2021;9:636257. doi: 10.3389/fbioe.2021.636257
- Saad M, El-Samad LM, Gomaa RA, Augustyniak M, Hassan MA. A comprehensive review of recent advances in silk sericin: extraction approaches, structure, biochemical characterization, and biomedical applications. Int J Biol Macromol 2023;250:126067. doi: 10.1016/j.ijbiomac.2023.126067
- Sahoo JK, Hasturk O, Falcucci T, Kaplan DL. Silk chemistry and biomedical material designs. Nat Rev Chem. 2023;7(5):302-318. doi: 10.1038/s41570-023-00486-x
- Liu J, Shi L, Deng Y, et al. Silk sericin-based materials for biomedical applications. Biomaterials. 2022;287:121638. doi: 10.1016/j.biomaterials.2022.121638
- Capar G, Pilevneli T. Cost-effective process development for sericin recovery from silk degumming wastewater. Sustain Chem Pharm. 2024;37:101405. doi: 10.1016/j.scp.2023.101405
- Silva AS, Costa EC, Reis S, et al. Silk sericin: a promising sustainable biomaterial for biomedical and pharmaceutical applications. Polymers. 2022;14(22):4931. doi: 10.3390/polym14224931
- Song Y, Zhang C, Zhang J, et al. An injectable silk sericin hydrogel promotes cardiac functional recovery after ischemic myocardial infarction. Acta Biomater. 2016;41:210-223. doi: 10.1016/j.actbio.2016.05.039
- Han L, Wang W, Chen Z, et al. Sericin-reinforced dual-crosslinked hydrogel for cartilage defect repair. Colloids Surf B Biointerfaces. 2023;222:113061. doi: 10.1016/j.colsurfb.2022.113061
- Zhang Y, Liu J, Huang L, Wang Z, Wang L. Design and performance of a sericin-alginate interpenetrating network hydrogel for cell and drug delivery. Sci Rep. 2015; 5:12374. doi: 10.1038/srep12374
- Li Y, Wei Y, Zhang G, Zhang Y. Sericin from fibroin-deficient silkworms served as a promising resource for biomedicine. Polymers. 2023;15(13):2941. doi: 10.3390/polym15132941
- Rahimpour S, Jabbari H, Yousofi H, et al. Regulatory effect of sericin protein in inflammatory pathways; a comprehensive review. Pathol Res Pract. 2023;243:154369. doi: 10.1016/j.prp.2023.154369
- Rujimongkon K, Ampawong S, Isarangkul D, Reamtong O, Aramwit P. Sericin-mediated improvement of dysmorphic cardiac mitochondria from hypercholesterolaemia is associated with maintaining mitochondrial dynamics, energy production, and mitochondrial structure. Pharm Biol. 2022;60(1):708-721. doi: 10.1080/13880209.2022.2055088
- Zhu Y, Liu H, Qin S, et al. Antibacterial sericin cryogels promote hemostasis by facilitating the activation of coagulation pathway and platelets. Adv Healthc Mater. 2022;11(11):2102717. doi: 10.1002/adhm.202102717
- Mukherjee S, Krishnan A, Athira RK, Kasoju N, Sah MK. Chapter 13 - silk fibroin and silk sericin in skin tissue engineering and wound healing: retrospect and prospects. In: Sah MK, Kasoju N, Mano JF, eds. Natural Polymers in Wound Healing and Repair. Elsevier; 2022: 301-331. doi: 10.1016/B978-0-323-90514-5.00005-5
- Vettori L, Sharma P, Rnjak-Kovacina J, Gentile C. 3D bioprinting of cardiovascular tissues for in vivo and in vitro applications using hybrid hydrogels containing silk fibroin: state of the art and challenges. Curr Tissue Microenviron Rep. 2020;1(4):261-276. doi: 10.1007/s43152-020-00026-5
- Song Y, Wang H, Yue F, et al. Silk-based biomaterials for cardiac tissue engineering. Adv Healthc Mater. 2020;9(23):e2000735. doi: 10.1002/adhm.202000735
- Tarsitano M, Mancuso A, Cristiano MC, Paolino D, Fresta M. In situ swelling formulation of glycerol-monooleate-derived lyotropic liquid crystals proposed for local vaginal application. Molecules. 2022;27(19):6295. doi: 10.3390/molecules27196295
- Mancuso A, Cianflone E, Cristiano MC, et al. Lyotropic liquid crystals: a biocompatible and safe material for local cardiac application. Pharmaceutics. 2022;14(2):452. doi: 10.3390/pharmaceutics14020452
- Mahmodi H, Piloni A, Utama RH, Kabakova I. Mechanical mapping of bioprinted hydrogel models by brillouin microscopy. Bioprinting. 2021;23:e00151. doi: 10.1016/j.bprint.2021.e00151
- Baptista M, Joukhdar H, Alcala-Orozco CR, et al. Silk fibroin photo-lyogels containing microchannels as a biomaterial platform for in situ tissue engineering. Biomater Sci. 2020;8(24):7093-7105. doi: 10.1039/d0bm01010c
- Schneider CA, Rasband WS, Eliceiri KW. NIH Image to ImageJ: 25 years of image analysis. Nat Methods. 2012;9(7):671-675. doi: 10.1038/nmeth.2089
- Tarsitano M, Liu Chung Ming C, Bennar L, et al. Chlorella-enriched hydrogels protect against myocardial damage and reactive oxygen species production in an in vitro ischemia/ reperfusion model using cardiac spheroids. Biofabrication. 2025;17(1):015006. doi: 10.1088/1758-5090/ad8266
- Zhang Y, Cao X, Zhang J, et al. A novel injectable sericin hydrogel with strong fluorescence for tracing. Int J Biol Macromol. 2024;258:129000. doi: 10.1016/j.ijbiomac.2023.129000
- Li J-X, Zhao S-X, Zhang Y-Q. Silk protein composite bioinks and their 3D scaffolds and in vitro characterization. Int J Mol Sci. 2022;23(2):910. doi: 10.3390/ijms23020910
- Vettori L, Tran HA, Mahmodi H, et al. Silk fibroin increases the elasticity of alginate-gelatin hydrogels and regulates cardiac cell contractile function in cardiac bioinks. Biofabrication. 2024;16(3):035025. doi: 10.1088/1758-5090/ad4f1b
- Baptista-Silva S, Borges S, Costa-Pinto AR, et al. In situ forming silk sericin-based hydrogel: a novel wound healing biomaterial. ACS Biomater Sci Eng. 2021;7(4):1573-1586. doi: 10.1021/acsbiomaterials.0c01745
- Chlapanidas T, Faragò S, Lucconi G, et al. Sericins exhibit ROS-scavenging, anti-tyrosinase, anti-elastase, and in vitro immunomodulatory activities. Int J Biol Macromol. 2013;58:47-56. doi: 10.1016/j.ijbiomac.2013.03.054
- Rocha LK, Favaro LI, Rios AC, et al. Sericin from Bombyx mori cocoons. Part I: extraction and physicochemical-biological characterization for biopharmaceutical applications. Process Biochem. 2017;61:163-177. doi: 10.1016/j.procbio.2017.06.019
- Sellimi S, Younes I, Ayed HB, et al. Structural, physicochemical and antioxidant properties of sodium alginate isolated from a Tunisian brown seaweed. Int J Biol Macromol. 2015;72:1358-1367. doi: 10.1016/j.ijbiomac.2014.10.016
- Rad MA, Mahmodi H, Filipe EC, Cox TR, Kabakova I, Tipper JL. Micromechanical characterisation of 3D bioprinted neural cell models using Brillouin microspectroscopy. Bioprinting. 2022;25:e00179. doi: 10.1016/j.bprint.2021.e00179
- Avallone PR, Romano M, Sarrica A, Delmonte M, Pasquino R, Grizzuti N. Effect of sugars on gelation kinetics of gelatin gels. Fluids. 2022;7(5):163. doi: 10.3390/fluids7050163
- Ahmad MI, Li Y, Pan J, et al. Collagen and gelatin: structure, properties, and applications in food industry. Int J Biol Macromol. 2024;254:128037. doi: 10.1016/j.ijbiomac.2023.128037
- Poon C, Chou J, Cortie M, Kabakova I. Brillouin imaging for studies of micromechanics in biology and biomedicine: from current state-of-the-art to future clinical translation. J Phys Photonics. 2021;3(1):012002. doi: 10.1088/2515-7647/abbf8c
- Rioboó RJJ, Gontán N, Sanderson D, Desco M, Gómez- Gaviro MV. Brillouin spectroscopy: from biomedical research to new generation pathology diagnosis. Int J Mol Sci. 2021;22(15):8055. doi: 10.3390/ijms22158055
- Kabakova I, Zhang J, Xiang Y, et al. Brillouin microscopy. Nature Revs Methods Primers. 2024;4(1):8. doi: 10.1038/s43586-023-00286-z
- Wu P-J, Kabakova IV, Ruberti JW, et al. Water content, not stiffness, dominates Brillouin spectroscopy measurements in hydrated materials. Nat Methods. 2018;15(8): 561-562. doi: 10.1038/s41592-018-0076-1
- Tao G, Cai R, Wang Y, Zuo H, He H. Fabrication of antibacterial sericin based hydrogel as an injectable and mouldable wound dressing. Mater Sci Eng C. 2021;119:111597. doi: 10.1016/j.msec.2020.111597
- Ekasurya W, Sebastian J, Puspitasari D, Asri PPP, Asri LATW. Synthesis and degradation properties of Sericin/ PVA hydrogels. Gels. 2023;9(2):76. doi: 10.3390/gels9020076
- Zhang Y, Chen H, Li Y, et al. A transparent sericin-polyacrylamide interpenetrating network hydrogel as visualized dressing material. Polymer Test. 2020; 87:106517. doi: 10.1016/j.polymertesting.2020.106517
- Miri AK, Mirzaee I, Hassan S, et al. Effective bioprinting resolution in tissue model fabrication. Lab Chip. 2019;19(11):2019-2037. doi: 10.1039/C8LC01037D
- Schwartz R, Malpica M, Thompson GL, Miri AK. Cell encapsulation in gelatin bioink impairs 3D bioprinting resolution. J Mech Behav Biomed Mater. 2020; 103:103524. doi: 10.1016/j.jmbbm.2019.103524