3D bioprinting of biomimetic trachea with interspersed cartilage and vascularized connective tissue for prompt segmental tracheal reconstruction
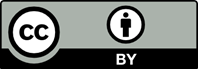
Segmental tracheal reconstruction remains a significant clinical challenge due to the lack of ideal tracheal substitutes. Three-dimensional (3D) bioprinting technique offers a promising avenue for the development of native-like tracheal substitutes. However, several challenges remain to be addressed, including deformation of 3D-printed C-shaped cell-laden hydrogels, fusion of regenerated C-shaped cartilage, and difficulty in regenerating vascularized connective tissue. Herein, we utilized 3D printing techniques to fabricate C-shaped chondrocyte-laden GelMA/ PCL (CcGP) and S-shaped PCL chains (SPC). These were assembled into the SPC-CcGP construct, followed by mold casting (VEGF@GelMA) to establish tissue-engineered tracheal construct (TETC). In vitro experiments confirmed the stable chondrogenic potential of CcGP and the angiogenic capability of VEGF@GelMA. Subcutaneous implantation in nude mice and orthotopic transplantation in rabbit model further demonstrated that the TETC could achieve stable regeneration of cartilage and vascularized connective tissue. The engineered trachea could maintain luminal patency following prompt repair of rabbit tracheal defects. This study offers a promising approach for developing engineered tracheas with regenerated cartilage and vascularized connective tissue for prompt and effective segmental tracheal defect repair.

- Wei S, Zhang Y, Luo F, et al. Tissue-engineered tracheal implants: advancements, challenges, and clinical considerations. Bioeng Transl Med. 2024;9(4):e10671. doi: 10.1002/btm2.10671
- Furlow PW, Mathisen DJ. Surgical anatomy of the trachea. Ann Cardiothorac Surg. 2018;7(2): 255-260. doi: 10.21037/acs.2018.03.01
- Matai I, Kaur G, Seyedsalehi A, McClinton A, Laurencin CT. Progress in 3D bioprinting technology for tissue/organ regenerative engineering. Biomaterials. 2020;226:119536. doi: 10.1016/j.biomaterials.2019.119536
- Wang P, Wang T, Xu Y, Song N, Zhang X. 3D-bioprinted cell-laden hydrogel with anti-inflammatory and anti-bacterial activities for tracheal cartilage regeneration and restoration. IJB. 2023;10(1):0146. doi: 10.36922/ijb.0146
- Sun Y, Huo Y, Ran X, et al. Instant trachea reconstruction using 3D-bioprinted C-shape biomimetic trachea based on tissue-specific matrix hydrogels. Bioact Mater. 2024;32:52-65. doi: 10.1016/j.bioactmat.2023.09.011
- Song X, Zhang P, Luo B, et al. Multi-tissue integrated tissue-engineered trachea regeneration based on 3D printed bioelastomer scaffolds. Adv Sci. 2024;11(39):e2405420. doi: 10.1002/advs.202405420
- Hsieh C.-T., Liao C.-Y., Dai N.-T., et al. 3D printing of tubular scaffolds with elasticity and complex structure from multiple waterborne polyurethanes for tracheal tissue engineering. Appl Mater Today. 2018;12:330-341. doi: 10.1016/j.apmt.2018.06.004
- Park JH, Ahn M, Park SH, et al. 3D bioprinting of a trachea-mimetic cellular construct of a clinically relevant size. Biomaterials. 2021;279:121246. doi: 10.1016/j.biomaterials.2021.121246
- Park JH, Park JY, Nam I-C, et al. A rational tissue engineering strategy based on three-dimensional (3D) printing for extensive circumferential tracheal reconstruction. Biomaterials. 2018;185: 276-283. doi: 10.1016/j.biomaterials.2018.09.031
- Xu Y, Guo Y, Li Y, et al. Biomimetic trachea regeneration using a modular ring strategy based on poly(sebacoyl diglyceride)/polycaprolactone for segmental trachea defect repair. Adv Funct Mater. 2020;30(42):2004276. doi: 10.1002/adfm.202004276
- Xu Y, Dai J, Zhu X, et al. Biomimetic trachea engineering via a modular ring strategy based on bone-marrow stem cells and atelocollagen for use in extensive tracheal reconstruction. Adv Mater. 2022;34(6):2106755. doi: 10.1002/adma.202106755
- Park JH, Hong JM, Ju YM, et al. A novel tissue-engineered trachea with a mechanical behavior similar to native trachea. Biomaterials. 2015;62:106-115. doi: 10.1016/j.biomaterials.2015.05.008.
- Tang H, Sun W, Liu X, et al. A bioengineered trachea-like structure improves survival in a rabbit tracheal defect model. Sci Transl Med. 2023;15(714):eabo4272. doi: 10.1126/scitranslmed.abo4272
- Gao E, Wang Y, Wang P, et al. C-shaped cartilage development using Wharton’s Jelly-derived hydrogels to assemble a highly biomimetic neotrachea for use in circumferential tracheal reconstruction. Adv Funct Mater. 2023;33(14):2212830. doi: 10.1002/adfm.202212830
- Luo X, Liu Y, Zhang Z, et al. Long-term functional reconstruction of segmental tracheal defect by pedicled tissue-engineered trachea in rabbits. Biomaterials. 2013; 34(13): 3336-3344. doi: 10.1016/j.biomaterials.2013.01.060
- Li D, Yin Z, Liu Y, et al. Regeneration of trachea graft with cartilage support, vascularization, and epithelization. Acta Biomater. 2019;89: 206-216. doi: 10.1016/j.actbio.2019.03.003
- Xu Y, Duan L, Li Y, et al. Nanofibrillar decellularized Wharton’s Jelly matrix for segmental tracheal repair. Adv Funct Mater. 2020;30(14):1910067. doi: 10.1002/adfm.201910067
- Gao E, Li G, Cao R, et al. Bionic tracheal tissue regeneration using a ring-shaped scaffold comprised of decellularized cartilaginous matrix and silk fibroin. Compos B: Eng. 2022;229:109470. doi: 10.1016/j.compositesb.2021.109470
- Xu W, Wu Y, Lu H, et al. Sustained delivery of vascular endothelial growth factor mediated by bioactive methacrylic anhydride hydrogel accelerates peripheral nerve regeneration after crush injury. Neural Regen Res. 2022;17(9):2064-2071. doi: 10.4103/1673-5374.335166
- Dikina AD, Alt DS, Herberg S, et al. A modular strategy to engineer complex tissues and organs. Adv Sci (Weinh). 2018;5(5):1700402. doi: 10.1002/advs.201700402
- Damiano G, Palumbo VD, Fazzotta S, et al. Current strategies for tracheal replacement: a review. Life (Basel). 2021;11(7):618. doi: 10.3390/life11070618
- Zhang YS, Haghiashtiani G, Hübscher T, et al. 3D extrusion bioprinting. Nat Rev Methods Primers. 2021;1(1):75. doi: 10.1038/s43586-021-00073-8
- Xu Y, Li D, Yin Z, et al. Tissue-engineered trachea regeneration using decellularized trachea matrix treated with laser micropore technique. Acta Biomater. 2017;58:113-121. doi: 10.1016/j.actbio.2017.05.010
- Crowley C, Klanrit P, Butler CR, et al. Surface modification of a POSS-nanocomposite material to enhance cellular integration of a synthetic bioscaffold. Biomaterials. 2016; 83:283-293. doi: 10.1016/j.biomaterials.2016.01.005
- Eming SA, Martin P, Tomic-Canic M. Wound repair and regeneration: mechanisms, signaling, and translation. Sci Transl Med. 2014;6(265):265sr6. doi: 10.1126/scitranslmed.3009337
- Hu K, Olsen BR. Vascular endothelial growth factor control mechanisms in skeletal growth and repair. Dev Dyn. 2017;246(4):227-234. doi: 10.1002/dvdy.24463
- Li M, Yin H, Yan Z, et al. The immune microenvironment in cartilage injury and repair. Acta Biomater. 2022;140:23-42. doi: 10.1016/j.actbio.2021.12.006
- Li X, Cho B, Martin R, et al. Nanofiber-hydrogel composite– mediated angiogenesis for soft tissue reconstruction. Sci Transl Med. 2019;11(490):eaau6210. doi: 10.1126/scitranslmed.aau6210
- Liu Y, Zheng K, Meng Z, et al. A cell-free tissue-engineered tracheal substitute with sequential cytokine release maintained airway opening in a rabbit tracheal full circumferential defect model. Biomaterials. 2023;300:122208. doi: 10.1016/j.biomaterials.2023.122208