Projection algorithm and its optimizations for computed axial lithography: A review
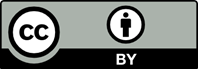
Volumetric additive manufacturing (AM) is a novel AM method that offers advantages such as fast printing speed and isotropic mechanical properties. As an important branch of volumetric AM, computed axial lithography (CAL) based on azimuthal projections and rotational printing has attracted great attention and has been widely used in recent years. Here, we focus on the projection algorithms of CAL, which is critical to the printing quality, including its fidelity and accuracy. Different optimization methods, including iterative optimization of projection space and objective model, optimization for the optical influences of materials, and optimizations with hardware upgrades, are summarized. The features and advantages of different projection optimization algorithms are also analyzed and discussed, which could promote the application and development of volumetric AM.

- Burger J, Huber T, Lloret-Fritschi E, et al. Design and fabrication of optimised ribbed concrete floor slabs using large scale 3D printed formwork. Automat Constr. 2022;114:104599. doi: 10.1016/j.autcon.2022.104599
- de Mattos Nascimento DL, Mury Nepomuceno R, Caiado RGG, et al. A sustainable circular 3D printing model for recycling metal scrap in the automotive industry. J Manuf Technol Manage. 2022;33:876-892. doi: 10.1108/JMTM-10-2021-0391
- Yan Q, Dong H, Song B, et al. A review of 3D printing technology for medical applications. Engineering. 2018;4:729-742. doi: 10.1016/j.eng.2018.07.021
- Atala A. Introduction: 3D printing for biomaterials. Chem Rev. 2020;120:10545-10546. doi: 10.1021/acs.chemrev.0c00139
- Joshi SC, Sheikh AA. 3D printing in aerospace and its long-term sustainability. Virtual Phys Prototyp. 2015;10:175-185. doi: 10.1080/17452759.2015.1111519
- Mishra PK, Jagadesh T. Applications and challenges of 3D printed polymer composites in the emerging domain of automotive and aerospace: a converged review. J Institut Eng (India): Ser C. 2023;104:849-866. doi: 10.1007/s40033-022-00426-x
- Tan K, Chua C, Leong K, et al. Selective laser sintering of biocompatible polymers for applications in tissue engineering. Biomed Mater Eng. 2005;15:113-124.
- Bertrand P, Bayle F, Combe C, et al. Ceramic components manufacturing by selective laser sintering. Appl Surf Sci. 2007;254:989-992. doi: 10.1016/j.apsusc.2007.08.085
- Olakanmi EO, Cochrane RF, Dalgarno KW, et al. A review on selective laser sintering/melting (SLS/SLM) of aluminium alloy powders: processing, microstructure, and properties. Prog Mater Sci. 2015;74:401-477. doi: 10.1016/j.pmatsci.2015.03.002
- Hu Q, Rance G, Trindade G, et al. The influence of printing parameters on multi-material two-photon polymerisation based micro additive manufacturing. Addit Manuf. 2022;51:102575. doi: 10.1016/j.addma.2021.102575
- Serles P, Haché M, Tam J, et al. Mechanically robust pyrolyzed carbon produced by two photon polymerization. Carbon. 2023;201:161-169. doi: 10.1016/j.carbon.2022.09.016
- Colombo F, Taale M, Taheri F, et al. Two-photon laser printing to mechanically stimulate multicellular systems in 3D. Adv Funct Mater. 2024;34:2303601. doi: 10.1002/adfm.202303601
- Zhang Y, Li D, Liu Y. Inkjet printing in liquid environments. Small. 2018;14:1801212. doi: 10.1002/smll.201801212
- Zub K, Hoeppener S, Schubert US. Inkjet printing and 3D printing strategies for biosensing, analytical, and diagnostic applications. Adv Mater. 2022;34:2105015. doi: 10.1002/adma.202105015
- Carou-Senra P, Rodriguez-Pombo L, Awad A, et al. Inkjet printing of pharmaceuticals. Adv Mater. 2024;36:2309164. doi: 10.1002/adma.202309164
- Yan Y, Xiong Z, Hu Y, et al. Layered manufacturing of tissue engineering scaffolds via multinozzle deposition. Mater Lett. 2003;57:2623-2628. doi: 10.1016/S0167-577X(02)01339-3
- Woodfield TB, Malda J, De Wijn J, et al. Design of porous scaffolds for cartilage tissue engineering using a three-dimensional fiber-deposition. Biomaterials. 2004;25:4149-4161. doi: 10.1016/j.biomaterials.2003.10.056
- Rajan K, Samykano M, Kadirgama K, et al. Fused deposition modeling: process, materials, parameters, properties, and applications. Int J Adv Manuf Technol. 2022;120:1531-1570. doi: 10.1007/s00170-022- 08860-7
- Mu Q, Wang L, Dun C, et al. Digital light processing 3D printing of conductive complex structures. Addit Manuf. 2017;18:74-83. doi: 10.1016/j.addma.2017.08.011
- Li W, Wang M, Mille LS, et al. A smartphone-enabled portable digital light processing 3D printer. Adv Mater. 2021;33:2102153. doi: 10.1002/adma.202102153
- He CF, Sun Y, He Y, et al. High-resolution projection-based 3D bioprinting. Nat Rev Bioeng. 2024:1-16. doi: 10.1038/s44222-024-00218-w
- Tumbleston JR, Shirvanyants D, Ermoshkin N, et al. Continuous liquid interface production of 3D objects. Science. 2015;347:1349-1352. doi: 10.1126/science.aaa2397
- Janusziewicz R, Tumbleston JR, Quintanilla AL, et al. Layerless fabrication with continuous liquid interface production. Proc Natl Acad Sci USA. 2016;113:11703-11708. doi: 10.1073/pnas.1605271113
- Hsiao K, Lee BJ, Samuelsen T, et al. Single-digit-micrometer-resolution continuous liquid interface production. Sci Adv. 2022;8:eabq2846. doi: 10.1126/sciadv.abq2846
- Jiménez M, Romero L, Dominguez IA, et al. Additive manufacturing technologies: an overview about 3D printing methods and future prospects. Complexity. 2019;2019:9656938. doi: 10.1155/2019/965693
- Quan H, Zhang T, Xu H, et al. Photocuring 3D printing technique and its challenges. Bioact Mater. 2020;5:110-115. doi: 10.1016/j.bioactmat.2019.12.003
- Oropallo W, Piegl LA. Ten challenges in 3D printing. Eng Comput. 2016;32:135-148. doi: 10.1007/s00366-015-0407-0
- Jambhulkar S, Ravichandran D, Sundaravadivelan B, Song K. Hybrid 3D printing for highly efficient nanoparticle micropatterning. J Mater Chem C. 2023;11:4333-4341. doi: 10.1039/D3TC00168G
- Dai C, Wang CC, Wu C, et al. Support-free volume printing by multi-axis motion. ACM Trans Graph. 2018;37:1-14. doi: 10.1145/3197517.3201342
- Bhattacharya I, Toombs J, Taylor H, et al. High fidelity volumetric additive manufacturing. Addit Manuf. 2021;47:102299. doi: 10.1016/j.addma.2021.102299
- Yao Y, Cheng L, Li Z. A comparative review of multiaxis 3D printing. J Manuf Process. 2024;120:1002-1022. doi: 10.1016/j.jmapro.2024.04.084
- Shusteff M, Browar AEM, Kelly BE, et al. One-step volumetric additive manufacturing of complex polymer structures. Sci Adv. 2017;3:eaao5496. doi: 10.1126/sciadv.aao5496
- Kelly BE, Bhattacharya I, Taylor HK, et al. Volumetric additive manufacturing via tomographic reconstruction. Science. 2019;363:1075-1079. doi: 10.1126/science.aau7114
- Van Der Laan HL, Burns MA, Scott TF. Volumetric photopolymerization confinement through dual-wavelength photoinitiation and photoinhibition. ACS Macro Lett. 2019;8: 899-904. doi: 10.1021/acsmacrolett.9b00412
- Regehly M, Garmshausen Y, Reuter M, et al. Xolography for linear volumetric 3D printing. Nature. 2020;588:620-624. doi: 10.1038/s41586-020-3029-7
- Cook CC, Fong EJ, Schwartz M, et al. Highly tunable thiol-ene photoresins for volumetric additive manufacturing. Adv Mater. 2020;32:2003376. doi: 10.1002/adma.202003376
- Li CC, Toombs J, Taylor H, et al. Tomographic color Schlieren refractive index mapping for computed axial lithography. Proc 5th Annu ACM Symp Comput Fabr. 2020;12:1–7. doi: 10.1145/3424630.3425421
- Orth A, Sampson KL, Zhang Y, et al. On-the-fly 3D metrology of volumetric additive manufacturing. Addit Manuf. 2022;56:102869. doi: 10.1016/j.addma.2022.102869
- Rackson CM, Toombs JT, De Beer MP, et al. Latent image volumetric additive manufacturing. Opt Lett. 2022;47:1279-1282. doi: 10.1364/OL.449220
- Salajeghe R, Meile DH, Kruse CS, et al. Numerical modeling of part sedimentation during volumetric additive manufacturing. Addit Manuf. 2023;66:103459. doi: 10.1016/j.addma.2023.103459
- Darkes-Burkey C, Shepherd RF. Volumetric 3D printing of endoskeletal soft robots. Adv Mater. 2024;36:2402217. doi: 10.1002/adma.202402217
- Bernal PN, Delrot P, Loterie D, et al. Volumetric bioprinting of complex living-tissue constructs within seconds. Adv Mater. 2019;31:1904209. doi: 10.1002/adma.201904209
- Rizzo R, Ruetsche D, Liu H, et al. Optimized photoclick (bio) resins for fast volumetric bioprinting. Adv Mater. 2021;33:2102900. doi: 10.1002/adma.202102900
- Rodríguez-Pombo L, Martínez-Castro L, Xu X, et al. Simultaneous fabrication of multiple tablets within seconds using tomographic volumetric 3D printing. Int J Pharm. 2023;5:100166. doi: 10.1016/j.ijpx.2023.100166
- Helgason S. The Radon Transform. Boston: Birkhäuser; 2019. doi: 10.1007/978-1-4757-1463-0
- Li CC, Toombs J, Taylor HK, et al. Tomographic projection optimization for volumetric additive manufacturing with general band constraint Lp-norm minimization. Addit Manuf. 2024;94:104447. doi: 10.1016/j.addma.2024.104447
- Rackson CM, Champley KM, Toombs JT, et al. Object-space optimization of tomographic reconstructions for additive manufacturing. Addit Manuf. 2021;48:102367. doi: 10.1016/j.addma.2021.102367
- Zhang Y, Liu M, Gao C, et al. Edge-enhanced object-space model optimization of tomographic reconstructions for additive manufacturing. Micromachines. 2023;14:1362. doi: 10.3390/mi1407136
- Chen T, Li HF, Liu X. Statistical iterative pattern generation in volumetric additive manufacturing based on ML-EM. Opt Commun. 2023;537:129448. doi: 10.1016/j.optcom.2023.129448
- Chen T, You S, Li HF, et al. High-fidelity tomographic additive manufacturing for large-volume and high-attenuation situations using expectation maximization algorithm. Addit Manuf. 2024;80:103968. doi: 10.1016/j.addma.2024.103968
- Madrid-Wolff J, Boniface A, Loterie D, et al. Controlling light in scattering materials for volumetric additive manufacturing. Adv Sci. 2022;9:2105144. doi: 10.1002/advs.202105144
- Loterie D, Delrot P, Moser C. High-resolution tomographic volumetric additive manufacturing. Nat Commun. 2020;11:852. doi: 10.1038/s41467-020-14630-4
- Webber D, Zhang Y, Picard M, et al. Versatile volumetric additive manufacturing with 3D ray tracing. Opt Express. 2023;31:5531-5546. doi: 10.1364/OE.481318
- Willemink MJ, Noël PB. The evolution of image reconstruction for CT—from filtered back projection to artificial intelligence. Eur Radiol. 2019;29:2185-2195. doi: 10.1007/s00330-018-5810-7
- Thijssen Q, Toombs J, Li CC, et al. From pixels to voxels: a mechanistic perspective on volumetric 3D-printing. Prog Polym Sci. 2023;147:101755. doi: 10.1016/j.progpolymsci.2023.101755
- Whyte DJ, Doeven EH, Sutti A, et al. Volumetric additive manufacturing: a new frontier in layer-less 3D printing. Addit Manuf. 2024;84:104094. doi: 10.1016/j.addma.2024.104094
- Calamai PH, Moré JJ. Projected gradient methods for linearly constrained problems. Math Program. 1987;39:93-116. doi: 10.1007/BF02592073.
- Fei Y, Rong G, Wang B, et al. Parallel L-BFGS-B algorithm on gpu. Comput Graph. 2014;40:1–9. doi: 10.1016/j.cag.2014.01.002
- Dempster AP, Laird NM, Rubin DB. Maximum likelihood from incomplete data via the EM algorithm. J R Stat Soc B. 1977;39:1-22. doi: 10.1111/j.2517-6161.1977.tb01600.x
- Yi S, Liu Q, Luo Z, et al. Micropore-forming gelatin methacryloyl (GelMA) biolink toolbox 2.0: designable tunability and adaptability for 3D bioprinting applications. Small 2022;18:2106357. doi: 10.1002/smll.202106357
- Weisgraber TH, de Beer MP, Huang S, et al. Virtual volumetric additive manufacturing (VirtualVAM). Adv Mater Technol. 2023;8:2301054. doi: 10.1002/admt.202301054