Revolutionizing regenerative rehabilitation: 3D printing for musculoskeletal regeneration
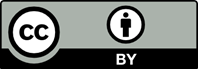
Musculoskeletal disorders, such as bone fractures, osteoarthritis, meniscus injuries, muscle diseases, and tendon injuries, are common disorders among individuals in need of rehabilitation. Trauma, sports-related injuries, and degeneration mainly cause these disorders. Regenerative rehabilitation medicine aims to enhance the regenerative capacity of musculoskeletal tissues and optimize functional recovery through the combination of regenerative medicine and rehabilitation medicine treatments. However, these treatments are insufficient for the complete restoration of damaged tissue structures. Three-dimensional (3D) printing technology, referred to as the additive manufacturing (AM) technique, can accelerate the rehabilitation process for musculoskeletal diseases, especially for developing regenerative rehabilitation and engineering rehabilitation. Recently, an increasing number of studies reported on the basic and clinical applications of 3D printing in assisting with the treatment of musculoskeletal disorders. Therefore, this review summarizes how 3D printing technologies are changing treatment and assessment methods, as well as improving therapeutic outcomes in regenerative rehabilitation for musculoskeletal diseases. Finally, this review discusses the current status of the clinical translation for 3D printing.

- Briggs AM, Cross MJ, Hoy DG, et al. Musculoskeletal health conditions represent a global threat to healthy aging: a report for the 2015 World Health Organization World Report on Ageing and Health. Gerontologist. 2016;56:S243-S255. doi: 10.1093/geront/gnw002
- Zheng YL, Song G, Guo JB. Interactions among lncRNA/ circRNA, miRNA, and mRNA in musculoskeletal degenerative diseases. Front Cell Dev Biol. 2021;9:753931. doi: 10.3389/fcell.2021.753931
- Cieza A, Causey K, Kamenov K, et al. Global estimates of the need for rehabilitation based on the Global Burden of Disease study 2019: a systematic analysis for the Global Burden of Disease Study 2019. Lancet. 2020;396(10267):2006-2017. doi: 10.1016/S0140-6736(20)32340-0
- Collins NJ, Hart HF, Mills KAG. Osteoarthritis year in review 2018: rehabilitation and outcomes. Osteoarthritis Cartilage. 2019;27(3):378-391. doi: 10.1016/j.joca.2018.11.010
- Zhu SY, Wang Z, Liang Q, et al. Chinese guidelines for the rehabilitation treatment of knee osteoarthritis: an CSPMR evidence-based practice guideline. J Evid-Based Med. 2023;16(3):376-393. doi: 10.1111/jebm.12555
- Cavanaugh JT, Killian SE. Rehabilitation following meniscal repair. Curr Rev Musculoskelet Med. 2012; 5(1):46-58. doi: 10.1007/s12178-011-9110-y
- Ambrosio F, Russell A. Regenerative rehabilitation: a call to action. J Rehabil Res Dev. 2010;47(3):Xi-Xv. doi: 10.1682/Jrrd.2010.03.0021
- Rando TA, Ambrosio F. Regenerative rehabilitation: applied biophysics meets stem cell therapeutics. Cell Stem Cell. 2018;22(3):306-309. doi: 10.1016/j.stem.2018.02.003
- Liaw CY, Guvendiren M. Current and emerging applications of 3D printing in medicine. Biofabrication. 2017; 9(2):024102. doi: 10.1088/1758-5090/aa7279
- Zhang JM, Hu QP, Wang S, et al. Digital light processing based three-dimensional printing for medical applications. Int J Bioprinting. 2020;6(1):12-27. doi: 10.18063/ijb.v6i1.242
- Murphy SV, Atala A. 3D bioprinting of tissues and organs. Nat Biotechnol. 2014;32(8):773-785. doi: 10.1038/nbt.2958
- Trucco D, Sharma A, Manferdini C, et al. Modeling and fabrication of silk fibroin–gelatin-based constructs using extrusion-based three-dimensional bioprinting. ACS Biomater Sci Eng. 2021;7(7):3306-3320. doi: 10.1021/acsbiomaterials.1c00410
- Zhou ZY, Cui J, Wu SL, et al. Silk fibroin-based biomaterials for cartilage/osteochondral repair. Theranostics. 2022;12(11):5103-5124. doi: 10.7150/thno.74548
- Pinto RMR, Faraji M, Vinayakumar KB. A 3D-printed printhead: custom inkjet dispenser for medium viscosity fluids. Adv Eng Mater. 2024;26(12):14. doi: 10.1002/adem.202400205
- Li XD, Liu BX, Pei B, et al. Inkjet bioprinting of biomaterials. Chem Rev. 2020;120(19):10596-10636. doi: 10.1021/acs.chemrev.0c00008
- Rasaki SA, Xiong DY, Xiong SF, et al. Photopolymerization-based additive manufacturing of ceramics: a systematic review. J Adv Ceram. 2021;10(3):442-471. doi: 10.1007/s40145-021-0468-z
- Zhang J, Hu Q, Wang S, et al. Digital light processing based three-dimensional printing for medical applications. Int J Bioprint. 2020;6(1):242. doi: 10.18063/ijb.v6i1.242
- Zhang P, Gao Q, Yu KC, et al. Investigation on the temperature control accuracy of a print head for extrusion 3D printing and its improved design. Biomedicines. 2022; 10(6):13. doi: 10.3390/biomedicines10061233
- Grivet-Brancot A, Boffito M, Ciardelli G. Use of polyesters in fused deposition modeling for biomedical applications. Macromol Biosci. 2022;22(10):29. doi: 10.1002/mabi.202200039
- Tabriz AG, Kuofie H, Scoble J, et al. Selective laser sintering for printing pharmaceutical dosage forms. J Drug Deliv Sci Technol. 2023;86:13. doi: 10.1016/j.jddst.2023.104699
- Han W, Kong LB, Xu M. Advances in selective laser sintering of polymers. Int J Extreme Manuf. 2022;4(4):37. doi: 10.1088/2631-7990/ac9096
- Wu M, Zheng K, Li W, et al. Nature‐inspired strategies for the treatment of osteoarthritis. Adv Funct Mater. 2023;34(4):2305603. doi: 10.1002/adfm.202305603
- Baniasadi H, Madani Z, Ajdary R, et al. Ascorbic acid-loaded polyvinyl alcohol/cellulose nanofibril hydrogels as precursors for 3D printed materials. Mater Sci Eng C-Mater Biol Appl. 2021;130:12. doi: 10.1016/j.msec.2021.112424
- Caprioli M, Roppolo I, Chiappone A, et al. 3D-printed self-healing hydrogels via digital light processing. Nat Commun. 2021;12(1):9. doi: 10.1038/s41467-021-22802-z
- Kumar A, Han SS. PVA-based hydrogels for tissue engineering: a review. Int J Polym Mater Polym Biomat. 2017;66(4):159-182. doi: 10.1080/00914037.2016.1190930
- Gu XC, Cheng HG, Lu XY, et al. Plant-based biomass/ polyvinyl alcohol gels for flexible sensors. Chem-Asian J. 2023;18(18):18. doi: 10.1002/asia.202300483
- Tümer EH, Erbil HY. Extrusion-based 3D printing applications of PLA composites: a review. Coatings. 2021;11(4):42. doi: 10.3390/coatings11040390
- Murab S, Herold S, Hawk T, et al. Advances in additive manufacturing of polycaprolactone based scaffolds for bone regeneration. J Mat Chem B. 2023;11(31):7250-7279. doi: 10.1039/d2tb02052a
- Yang XJ, Wang YT, Zhou Y, et al. The application of polycaprolactone in three-dimensional printing scaffolds for bone tissue engineering. Polymers. 2021;13(16):19. doi: 10.3390/polym13162754
- Hamedi E, Vahedi N, Sigaroodi F, et al. Recent progress of bio-printed PEGDA-based bioinks for tissue regeneration. Polym Adv Technol. 2023;34(11):3505-3517. doi: 10.1002/pat.6164
- Liang WQ, Zhou C, Zhang HW, et al. Recent advances in 3D printing of biodegradable metals for orthopaedic applications. J Biol Eng. 2023;17(1):24. doi: 10.1186/s13036-023-00371-7
- Martinelli A, Nitti A, Po RC, et al. 3D printing of layered structures of metal-ionic polymers: recent progress, challenges and opportunities. Materials. 2023; 16(15):16. doi: 10.3390/ma16155327
- Velásquez-García LF, Kornbluth Y. Biomedical applications of metal 3D printing. In: Yarmush ML, ed. Annual Review of Biomedical Engineering, vol. 23. Palo Alto: Annual Reviews 2021:307-338. doi: 10.1146/annurev-bioeng-082020-032402
- Ni J, Ling H, Zhang S, et al. Three-dimensional printing of metals for biomedical applications. Mater Today Bio. 2019;3:18. doi: 10.1016/j.mtbio.2019.100024
- Yuan XY, Xu YB, Lu TL, et al. Enhancing the bioactivity of hydroxyapatite bioceramic via encapsulating with silica-based bioactive glass sol. J Mech Behav Biomed Mater. 2022;128:9. doi: 10.1016/j.jmbbm.2022.105104
- Liu MY, Wang YE, Liu XW, et al. Comprehensive review on fabricating bioactive ceramic bone scaffold using vat photopolymerization. ACS Biomater Sci Eng. 2023;9(6):3032-3057. doi: 10.1021/acsbiomaterials.3c00051
- Vennam S, Vijayasankar KN, Pati F. 3D printed personalized assistive devices: a material, technique, and medical condition perspective. Appl Mater Today. 2024;40:15. doi: 10.1016/j.apmt.2024.102403
- Li JQ, Chen SG, Shang X, et al. Research progress of rehabilitation orthoses based on 3D printing technology. Adv Mater Sci Eng. 2022;2022:16. doi: 10.1155/2022/5321570
- Buchner TJK, Rogler S, Weirich S, et al. Vision-controlled jetting for composite systems and robots. Nature. 2023;623(7987):522-530. doi: 10.1038/s41586-023-06684-3
- Wang B, Feng CW, Pan JY, et al. The effect of 3D printing metal materials on osteoporosis treatment. Biomed Res Int. 2021;2021:7. doi: 10.1155/2021/9972867
- Wu D, Zhao XS, Xie JH, et al. Physical modulation of mesenchymal stem cell exosomes: a new perspective for regenerative medicine. Cell Prolif. 2024;57(8):16. doi: 10.1111/cpr.13630
- Liu S, Zhang LG, Li Z, et al. Materials-mediated in situ physical cues for bone regeneration. Adv Funct Mater. 2024;34(1):32. doi: 10.1002/adfm.202306534
- Shuai CJ, Yang WJ, Peng SP, et al. Physical stimulations and their osteogenesis-inducing mechanisms. Int J Bioprinting. 2018;4(2):20. doi: 10.18063/IJB.v4i2.138
- Huang X, Das R, Patel A, et al. Physical Stimulations for Bone and Cartilage Regeneration. Regen Eng Transl Med. 2018;4(4):216-237. doi: 10.1007/s40883-018-0064-0
- Liang WX, Liang BB, Yan KC, et al. Low-intensity pulsed ultrasound: a physical stimulus with immunomodulatory and anti-inflammatory potential. Ann Biomed Eng. 2024;52(8):1955-1981. doi: 10.1007/s10439-024-03523-y
- Luo TY, Tan BW, Liao JF, et al. A review on external physical stimuli with biomaterials for bone repair. Chem Eng J. 2024;496:18. doi: 10.1016/j.cej.2024.153749
- Axtell LA, Yasuda YL. Assistive devices and home modifications in geriatric rehabilitation. Clin Geriatr Med. 1993;9(4):803-821. doi: 10.1016/S0749-0690(18)30378-1
- Nyberg EL, Farris AL, Hung B, et al. 3D-printing technologies for craniofacial rehabilitation, reconstruction, and regeneration. Ann Biomed Eng. 2017;45(1):45-57. doi: 10.1007/s10439-016-1668-5
- Cuellar JS, Smit G, Breedveld P, et al. Functional evaluation of a non-assembly 3D-printed hand prosthesis. P I Mech Eng H. 2019;233(11):1122-1131. doi: 10.1177/0954411919874523
- Rumfelt S, Mintz T, Brooks A. Rehabilitation and orthoses for adult hand fractures. In: Abzug JM, Gaston RG, Osterman AL, et al., eds. Pediatric and Adult Hand Fractures. Cham: Springer International Publishing; 2023: 389-413. doi: 10.1007/978-3-031-32072-9_22
- Walker KJ, Przestrzelski BT, Kaluf B, et al. Novel 3D-printed foot orthoses with variable hardness: a comfort comparison to traditional orthoses. Med Eng Phys. 2023;115:103978. doi: 10.1016/j.medengphy.2023.103978
- Xu GS, Gao L, Tao K, et al. Three-dimensional-printed upper limb prosthesis for a child with traumatic amputation of right wrist: a case report. Medicine. 2017;96(52):5. doi: 10.1097/md.0000000000009426
- Dombroski CE, Balsdon MER, Froats A. The use of a low cost 3D scanning and printing tool in the manufacture of custom-made foot orthoses: a preliminary study. BMC Res Notes. 2014;7(1):443. doi: 10.1186/1756-0500-7-443
- Silva K, Rand S, Cancel D, et al. Three-dimensional (3-D) printing: a cost-effective solution for improving global accessibility to prostheses. Pm&R. 2015;7(12):1312-1314. doi: 10.1016/j.pmrj.2015.06.438
- Stout A, Friedly J, Standaert CJ. Systemic absorption and side effects of locally injected glucocorticoids. Pm&R. 2019;11(4):409-419. doi: 10.1002/pmrj.12042
- Majrashi M, Kotowska A, Scurr D, et al. Sustained release of dexamethasone from 3D-printed scaffolds modulates macrophage activation and enhances osteogenic differentiation. ACS Appl Mater Interfaces. 2023;15(49):56623-56638. doi: 10.1021/acsami.3c09774
- Liao CD, Chen HC, Huang MH, et al. Comparative efficacy of intra-articular injection, physical therapy, and combined treatments on pain, function, and sarcopenia indices in knee osteoarthritis: a network meta-analysis of randomized controlled trials. Int J Mol Sci. 2023;24(7):24. doi: 10.3390/ijms24076078
- Park YB, Ha CW, Lee CH, et al. Cartilage regeneration in osteoarthritic patients by a composite of allogeneic umbilical cord blood-derived mesenchymal stem cells and hyaluronate hydrogel: results from a clinical trial for safety and proof-of-concept with 7 years of extended follow-up. Stem Cells Transl Med. 2017;6(2):613-621. doi: 10.5966/sctm.2016-0157
- Rim YA, Nam Y, Ju JH. The role of chondrocyte hypertrophy and senescence in osteoarthritis initiation and progression. Int J Mol Sci. 2020;21(7):2358. doi: 10.3390/ijms21072358
- Fu LL, Li L, Bian QY, et al. Cartilage-like protein hydrogels engineered via entanglement. Nature. 2023;618(7966):740-747. doi: 10.1038/s41586-023-06037-0
- Cho Y, Jeong S, Kim H, et al. Disease-modifying therapeutic strategies in osteoarthritis: current status and future directions. Exp Mol Med. 2021;53(11):1689-1696. doi: 10.1038/s12276-021-00710-y
- Grässel S, Aszodi A. Osteoarthritis and cartilage regeneration: focus on pathophysiology and molecular mechanisms. Int J Mol Sci. 2019;20(24):6156. doi: 10.3390/ijms20246156
- Schroeppel JP, Crist JD, Anderson HC, et al. Molecular regulation of articular chondrocyte function and its significance in osteoarthritis. Histol Histopathol. 2011;26(3):377-394. doi: 10.14670/HH-26.377
- Li Q, Yu H, Zhao F, et al. 3D printing of microenvironment‐specific bioinspired and exosome‐reinforced hydrogel scaffolds for efficient cartilage and subchondral bone regeneration. Adv Sci. 2023;10(26):2303650. doi: 10.1002/advs.202303650
- Jiang GY, Li SH, Yu K, et al. A 3D-printed PRP-GelMA hydrogel promotes osteochondral regeneration through M2 macrophage polarization in a rabbit model. Acta Biomater. 2021;128:150-162. doi: 10.1016/j.actbio.2021.04.010
- Zhang H, Huang H, Hao G, et al. 3D printing hydrogel scaffolds with nanohydroxyapatite gradient to effectively repair osteochondral defects in rats. Adv Funct Mater. 2020;31(1):2006697. doi: 10.1002/adfm.202006697
- Sardroud HA, Chen XB, Eames BF. Applied compressive strain governs hyaline-like cartilage versus fibrocartilage-like ECM produced within hydrogel constructs. Int J Mol Sci. 2023;24(8):17. doi: 10.3390/ijms24087410
- Gong YP, Wang F, Al-Furjan MSH, et al. Experimental investigation and optimal 3d bioprinting parameters of SA-Gel porous cartilage scaffold. Appl Sci-Basel. 2020;10(3):768. doi: 10.3390/app10030768
- Shojarazavi N, Mashayekhan S, Pazooki H, et al. Alginate/cartilage extracellular matrix-based injectable interpenetrating polymer network hydrogel for cartilage tissue engineering. J Biomater Appl. 2021;36(5):803-817. doi: 10.1177/08853282211024020
- Ma FB, Ge YM, Liu N, et al. fabrication of a composite hydrogel with tunable mechanical properties for cartilage tissue engineering. J Mat Chem B. 2019;7(15):2463-2473. doi: 10.1039/c8tb01331d
- Deng CX, Yang J, He HT, et al. 3D bio-printed biphasic scaffolds with dual modification of silk fibroin for the integrated repair of osteochondral defects. Biomater Sci. 2021;9(14):4891-4903. doi: 10.1039/d1bm00535a
- Sadeghianmaryan A, Naghieh S, Yazdanpanah Z, et al. Fabrication of chitosan/alginate/hydroxyapatite hybrid scaffolds using 3D printing and impregnating techniques for potential cartilage regeneration. Int J Biol Macromol. 2022;204:62-75. doi: 10.1016/j.ijbiomac.2022.01.201
- Cheng K, Xia P, Lin Q, et al. Effects of low-intensity pulsed ultrasound on integrin-FAK-PI3K/Akt mechanochemical transduction in rabbit osteoarthritis chondrocytes. Ultrasound Med Biol. 2014;40(7):1609-1618. doi: 10.1016/j.ultrasmedbio.2014.03.002
- Prasopthum A, Deng ZX, Khan IM, et al. Three dimensional printed degradable and conductive polymer scaffolds promote chondrogenic differentiation of chondroprogenitor cells. Biomater Sci. 2020;8(15):4287-4298. doi: 10.1039/d0bm00621a
- Gee SM, Posner M. Meniscus anatomy and basic science. Sports Med Arthrosc. 2021;29(3):E18-E23. doi: 10.1097/Jsa.0000000000000327
- Makris EA, Hadidi P, Athanasiou KA. The knee meniscus: structure–function, pathophysiology, current repair techniques, and prospects for regeneration. Biomaterials. 2011;32(30):7411-7431. doi: 10.1016/j.biomaterials.2011.06.037
- Li L, Yang LF, Zhang KJ, et al. Three-dimensional finite-element analysis of aggravating medial meniscus tears on knee osteoarthritis. J Orthop Transl. 2020; 20:47-55. doi: 10.1016/j.jot.2019.06.007
- Tarafder S, Gulko J, Kim D, et al. Effect of dose and release rate of CTGF and TGFβ3 on avascular meniscus healing. J Orthop Res. 2019;37(7):1555-1562. doi: 10.1002/jor.24287
- Pauli C, Grogan SP, Patil S, et al. Macroscopic and histopathologic analysis of human knee menisci in aging and osteoarthritis. Osteoarthritis Cartilage. 2011;19(9):1132-1141. doi: 10.1016/j.joca.2011.05.008
- Murphy CA, Garg AK, Silva-Correia J, et al. The meniscus in normal and osteoarthritic tissues: facing the structure property challenges and current treatment trends. Annu Rev Biomed Eng. 2019;21(1):495-521. doi: 10.1146/annurev-bioeng-060418-052547
- Wang B, Barceló X, Von Euw S, et al. 3D printing of mechanically functional meniscal tissue equivalents using high concentration extracellular matrix inks. Mater Today Bio. 2023;20:12. doi: 10.1016/j.mtbio.2023.100624
- Gunja NJ, Huey DJ, James RA, et al. Effects of agarose mould compliance and surface roughness on self-assembled meniscus-shaped constructs. J Tissue Eng Regen Med. 2009;3(7):521-530. doi: 10.1002/term.191
- Jian Z, Zhuang T, Qinyu T. 3D bioprinting of a biomimetic meniscal scaffold for application in tissue engineering. Bioact Mater. 2021;6(6):1711-1726. doi: 10.1016/j.bioactmat.2020.11.027
- Zhang Q, Xu Z, Zhang X, et al. 3D printed high‐strength supramolecular polymer hydrogel‐cushioned radially and circumferentially oriented meniscus substitute. Adv Funct Mater. 2022;32(23):2200360. doi: 10.1002/adfm.202200360
- Chevrier A, Nelea M, Hurtig MB, et al. Meniscus structure in human, sheep, and rabbit for animal models of meniscus repair. J Orthop Res. 2009;27(9):1197-1203. doi: 10.1002/jor.20869
- Cheung HS. Distribution of Type-I, Type-Ii, Type-Iii, and Type-V in the pepsin solubilized collagens in bovine menisci. Connect Tissue Res. 1987;16(4):343-356. doi: 10.3109/03008208709005619
- Yan WQ, Maimaitimin M, Wu Y, et al. Meniscal fibrocartilage regeneration inspired by meniscal maturational and regenerative process. Sci Adv. 2023;9(45):eadg8138. doi: 10.1126/sciadv.adg8138
- Chae S, Lee S-S, Choi Y-J, et al. 3D cell-printing of biocompatible and functional meniscus constructs using meniscus‐derived bioink. Biomaterials. 2021;267:120466. doi: 10.1016/j.biomaterials.2020.120466
- Grogan SP, Duffy SF, Pauli C, et al. Gene expression profiles of the meniscus avascular phenotype: a guide for meniscus tissue engineering. J Orthop Res. 2018;36(7):1947-1958. doi: 10.1002/jor.23864
- Whitney NP, Lamb AC, Louw TM, et al. Integrin-mediated mechanotransduction pathway of low-intensity continuous ultrasound in human chondrocytes. Ultrasound Med Biol. 2012;38(10):1734-1743. doi: 10.1016/j.ultrasmedbio.2012.06.002
- Babaei M, Jamshidi N, Amiri F, et al. Effects of low-intensity pulsed ultrasound stimulation on cell seeded 3D hybrid scaffold as a novel strategy for meniscus regeneration: an in vitro study. J Tissue Eng Regen Med. 2022;16(9):812-824. doi: 10.1002/term.3331
- Ebner N, Sliziuk V, Scherbakov N, et al. Muscle wasting in ageing and chronic illness. ESC Heart Fail. 2015;2(2): 58-68. doi: 10.1002/ehf2.12033
- Voet NBM, van der Kooi EL, Riphagen II, et al. Strength training and aerobic exercise training for muscle disease. Cochrane Database Syst Rev. 2013;(7):CD003907. doi: 10.1002/14651858.CD003907.pub4
- Grogan BF, Hsu JR, Consortium STR. Volumetric muscle loss. J Am Acad Orthop Surg. 2011;19:S35-S37. doi: 10.5435/00124635-201102001-00007
- Basurto IM, Passipieri JA, Gardner GM, et al. Photoreactive hydrogel stiffness influences volumetric muscle loss repair. Tissue Eng Part A. 2022;28(7-8):312-329. doi: 10.1089/ten.tea.2021.0137
- Shayan M, Huang NF. Pre-clinical cell therapeutic approaches for repair of volumetric muscle loss. Bioeng Basel. 2020;7(3):97. doi: 10.3390/bioengineering7030097
- Nakayama KH, Quarta M, Paine P, et al. Treatment of volumetric muscle loss in mice using nanofibrillar scaffolds enhances vascular organization and integration. Commun Biol. 2019;2(1):170. doi: 10.1038/s42003-019-0416-4
- Eugenis I, Wu D, Rando TA. Cells, scaffolds, and bioactive factors: engineering strategies for improving regeneration following volumetric muscle loss. Biomaterials. 2021;278:121173. doi: 10.1016/j.biomaterials.2021.121173
- Shen S, Chen MX, Guo WM, et al. Three dimensional printing-based strategies for functional cartilage regeneration. Tissue Eng Part B Rev. 2019;25(3):187-201. doi: 10.1089/ten.teb.2018.0248
- Hwangbo H, Lee H, Jin EJ, et al. Bio-printing of aligned GelMa-based cell-laden structure for muscle tissue regeneration. Bioact Mater. 2022;8:57-70. doi: 10.1016/j.bioactmat.2021.06.031
- Gokyer S, Yilgor E, Yilgor I, et al. 3D printed biodegradable polyurethaneurea elastomer recapitulates skeletal muscle structure and function. ACS Biomater Sci Eng. 2021;7(11):5189-5205. doi: 10.1021/acsbiomaterials.1c00703
- Latroche C, Gitiaux C, Chrétien F, et al. Skeletal muscle microvasculature: a highly dynamic lifeline. Physiology. 2015;30(6):417-427. doi: 10.1152/physiol.00026.2015
- Luo ZY, Tang GS, Ravanbakhsh H, et al. Vertical extrusion cryo(bio)printing for anisotropic tissue manufacturing. Adv Mater. 2022;34(12):2108931. doi: 10.1002/adma.202108931
- Kim JH, Seol YJ, Ko IK, et al. 3D bioprinted human skeletal muscle constructs for muscle function restoration. Sci Rep. 2018;8(1):12307. doi: 10.1038/s41598-018-29968-5
- Andarawis‐Puri N, Flatow EL, Soslowsky LJ. Tendon basic science: development, repair, regeneration, and healing. J Orthop Res. 2015;33(6):780-784. doi: 10.1002/jor.22869
- Zhang YJ, Lei TY, Tang CQ, et al. 3D printing of chemical-empowered tendon stem/progenitor cells for functional tissue repair. Biomaterials. 2021;271:120722. doi: 10.1016/j.biomaterials.2021.120722
- Mccormick A, Charlton J, Fleming D. Assessing health needs in primary-care - morbidity study from general-practice provides another source of information. Br Med J. 1995;310(6993):1534. doi: 10.1136/bmj.310.6993.1534d
- Murray MM. Current status and potential of primary ACL repair. Clin Sports Med. 2009;28(1):51-61. doi: 10.1016/j.csm.2008.08.005
- Li CS, Wang J, Yang WA, et al. 3D-printed hydrogel particles containing PRP laden with TDSCs promote tendon repair in a rat model of tendinopathy. J Nanobiotechnol. 2023;21(1):177. doi: 10.1186/s12951-023-01892-5
- Kim D, Kim G. Bioprinted hASC-laden cell constructs with mechanically stable and cell alignment cue for tenogenic differentiation. Biofabrication. 2023;15(4):045006. doi: 10.1088/1758-5090/ace740
- Patel M, Amini MH. Management of acute rotator cuff tears. Orthop Clin North Am. 2022;53(1):69-76. doi: 10.1016/j.ocl.2021.08.003
- Keener JD, Patterson BM, Orvets N, et al. Degenerative rotator cuff tears: refining surgical indications based on natural history data. J Am Acad Orthop Surg. 2019;27(5):156-165. doi: 10.5435/Jaaos-D-17-00480
- Huang Y, He B, Wang L, et al. Bone marrow mesenchymal stem cell-derived exosomes promote rotator cuff tendon-bone healing by promoting angiogenesis and regulating M1 macrophages in rats. Stem Cell Res Ther. 2020;11(1):496. doi: 10.1186/s13287-020-02005-x
- Rothrauff BB, Tuan RS. Cellular therapy in bone-tendon interface regeneration. Organogenesis. 2014;10(1):13-28. doi: 10.4161/org.27404
- Cao Y, Yang SB, Zhao DY, et al. Three-dimensional printed multiphasic scaffolds with stratified cell-laden gelatin methacrylate hydrogels for biomimetic tendon-to-bone interface engineering. J Orthop Transl. 2020;23:89-100. doi: 10.1016/j.jot.2020.01.004
- Gaffney LS, Davis ZG, Mora-Navarro C, et al. Extracellular matrix hydrogels promote expression of muscle-tendon junction proteins. Tissue Eng Part A. 2022;28(5-6):270-282. doi: 10.1089/ten.tea.2021.0070
- Hou YB, Gao M, Gao JW, et al. 3D printed conformal strain and humidity sensors for human motion prediction and health monitoring via machine learning. Adv Sci. 2023;10(36):e2304132. doi: 10.1002/advs.202304132
- Paterson TE, Hagis N, Boufidis D, et al. Monitoring of hand function enabled by low complexity sensors printed on textile. Flex Print Electron. 2022;7(3):035003. doi: 10.1088/2058-8585/ac7dd1
- Chen RY, Pye JS, Li JR, et al. Multiphasic scaffolds for the repair of osteochondral defects: outcomes of preclinical studies. Bioact Mater. 2023;27:505-545. doi: 10.1016/j.bioactmat.2023.04.016
- Caliogna L, Bina V, Brancato AM, et al. The Role of PEMFs on Bone Healing: An In Vitro Study. Int J Mol Sci. 2022;23(22):17. doi: 10.3390/ijms232214298
- Lang S, Ma J, Gong S, et al. Pulse electromagnetic field for treating postmenopausal osteoporosis: a systematic review and meta‐analysis of randomized controlled trials. Bioelectromagnetics. 2022;43(6):381-393. doi: 10.1002/bem.22419
- Tong J, Chen ZY, Sun GH, et al. The efficacy of pulsed electromagnetic fields on pain, stiffness, and physical function in osteoarthritis: a systematic review and meta-analysis. Pain Res Manag. 2022;2022:9939891. doi: 10.1155/2022/9939891
- Stefani RM, Barbosa S, Tan ARR, et al. Pulsed electromagnetic fields promote repair of focal articular cartilage defects with engineered osteochondral constructs. Biotechnol Bioeng. 2020;117(5):1584-1596. doi: 10.1002/bit.27287
- Liang Q, Ma Y, Yao X, et al. Advanced 3D-printing bioinks for articular cartilage repair. Int J Bioprinting. 2022;8(3):511. doi: 10.18063/ijb.v8i3.511
- Pugliese R, Regondi S. Artificial intelligence-empowered 3d and 4d printing technologies toward smarter biomedical materials and approaches. Polymers. 2022;14(14):2794. doi: 10.3390/polym14142794
- Gao B, Yang QZ, Zhao X, et al. 4D bioprinting for biomedical applications. Trends Biotechnol. 2016;34(9):746-756. doi: 10.1016/j.tibtech.2016.03.004