Application of 3D printing in bacterial detection
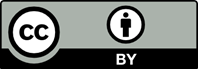
Diseases caused by bacterial infections, especially pathogen variations and drug-resistant bacteria, are a serious threat to human health globally. Accurate and rapid identification of bacterial pathogens is essential for early diagnosis of infections and timely treatment of disease. At present, the routine diagnostic methods for identifying bacterial pathogens in clinic are bacterial culture, immunological detection, genetic analysis, etc. These methods occupy foundational position for bacterial detection that favors for clinical diagnosis of pathogen infection in daily life. Notably, 3D printing technology, together with 3D bioprinting technology, provides more options for bacterial detection due to its personalized design and manufacturing. Compared with traditional methods, 3D printing provides a more convenient, rapid and accurate bacterial detection platform, thus meeting the urgent needs of clinical and scientific research. Here, we have summarized the research progress and given a comprehensive review of 3D printing technology in bacterial detection, including bacterial detection sensors, microfluidic chips, bioprinting microarray technology, AI and spectroscopy technology, providing a scientific reference and filling in gaps in 3D printing-assistance bacterial detection. At the same time, technical advantages, challenges and future development trends will also be discussed in related healthcare fields.

- Varadi L, Luo JL, Hibbs DE, et al. Methods for the detection and identification of pathogenic bacteria: past, present, and future. Chem Soc Rev. 2017;46(16):4818-4832. doi: 10.1039/c6cs00693k
- Huber F, Lang HP, Lang D, et al. Rapid and ultrasensitive detection of mutations and genes relevant to antimicrobial resistance in bacteria. Glob Chall. 2021;5(2):2000066. doi: 10.1002/gch2.202000066
- Tang JW, Yuan Q, Wen XR, et al. Label‐free surface‐enhanced Raman spectroscopy coupled with machine learning algorithms in pathogenic microbial identification: current trends, challenges, and perspectives. Interdiscip Med. 2024;2(3):e20230060. doi: 10.1002/inmd.20230060
- Santoni S, Gugliandolo SG, Sponchioni M, Moscatelli D, Colosimo BM. 3D bioprinting: current status and trends—a guide to the literature and industrial practice. Bio-Des Manuf. 2021;5(1):14-42. doi: 10.1007/s42242-021-00165-0
- Md Fadilah NI, Khairul Nizam NAA, Fauzi MB. Antibacterial compounds-incorporated functional biomaterials for chronic wound healing application via 3D bioprinting: the mechanism of action. Int J Bioprint. 2024; 10(4):3372. doi: 10.36922/ijb.3372
- Pramanik J, Brahma B, Pradhan S, et al. 3D printing application in biomedical—a review. Mater Today Proc. 2023. doi: 10.1016/j.matpr.2023.07.046
- Ryoo M, Kim D, Noh J, Ih Ahn S. 3D-printed electronics for biomedical applications. Int J Bioprint. 2024. doi: 10.36922/ijb.4139
- Liu Y, Sing S L. A review of advances in additive manufacturing and the integration of high-performance polymers, alloys, and their composites. Mater Sci Addit Manuf. 2023;2(3):1587. doi: 10.36922/msam.1587
- Manos J. The human microbiome in disease and pathology. APMIS. 2022;130(12):690-705. doi: 10.1111/apm.13225
- Ding X, Ma J, Fan T, et al. Inorganic nanoparticles‐based strategies for the microbial detection in infectious diseases. Interdiscip Med. 2024;2(2):e20230045. doi: 10.1002/inmd.20230045
- Sheikhzadeh E, Chamsaz M, Turner APF, Jager EWH, Beni V. Label-free impedimetric biosensor for Salmonella Typhimurium detection based on poly [pyrrole-co-3- carboxyl-pyrrole] copolymer supported aptamer. Biosens Bioelectron. 2016;80:194-200. doi: 10.1016/j.bios.2016.01.057
- Nguyen TT, Trinh KTL, Yoon WJ, Lee NY, Ju H. Integration of a microfluidic polymerase chain reaction device and surface plasmon resonance fiber sensor into an inline all-in-one platform for pathogenic bacteria detection. Sens Actuators B. 2017;242:1-8. doi: 10.1016/j.snb.2016.10.137
- Jackson N, Wu TZ, Adams-Sapper S, et al. A multiplexed, indirect enzyme-linked immunoassay for the detection and differentiation of E. coli from other Enterobacteriaceae and P. aeruginosa from other glucose non-fermenters. J Microbiol Methods. 2019;158(1):52-58. doi: 10.1016/j.mimet.2019.01.014
- Ellington M J, Ekelund O, Aarestrup FM, et al. The role of whole genome sequencing in antimicrobial susceptibility testing of bacteria: report from the EUCAST Subcommittee. Clin Microbiol Infect. 2017;23(1):2-22. doi: 10.1016/j.cmi.2016.11.012
- Shen W, Wang C, Zheng S, et al. Ultrasensitive multichannel immunochromatographic assay for rapid detection of foodborne bacteria based on two-dimensional film-like SERS labels. J Hazard Mater. 2022;437:129347. doi: 10.1016/j.jhazmat.2022.129347
- Gopinath SC, Tang TH, Chen Y, Citartan M, Lakshmipriya T. Bacterial detection: from microscope to smartphone. Biosens Bioelectron. 2014;60:332-342. doi: 10.1016/j.bios.2014.04.014
- Braga PAC, Tata A, Gonçalves dos Santos V, et al. Bacterial identification: from the agar plate to the mass spectrometer. RSC Adv. 2013;3(4):994-1008. doi: 10.1039/c2ra22063f
- Yang M, Wang Z, Su M, et al. Smart nanozymes for diagnosis of bacterial infection: the next frontier from laboratory to bedside testing. ACS Appl Mater Interfaces. 2024;16(34) 44361-44375. doi: 10.1021/acsami.4c07043
- Kabiraz MP, Majumdar PR, Mahmud MMC, Bhowmik S, Ali A. Conventional and advanced detection techniques of foodborne pathogens: a comprehensive review. Heliyon. 2023;9(4):e15482. doi: 10.1016/j.heliyon.2023.e15482
- Aladhadh M. A review of modern methods for the detection of foodborne pathogens. Microorganisms. 2023;11(5):1111. doi: 10.3390/microorganisms11051111
- Rodino Kyle G, Wolf Matthew J, Sheldon S, et al. Detection of tick-borne bacteria from whole blood using 16S ribosomal RNA gene PCR followed by next-generation sequencing. J Clin Microbiol. 2021;59(5):e03129-20. doi: 10.1128/JCM.03129-20
- Yang Q, Zu J, Zhang S, et al. An overview of rapid detection methods for Salmonella. Food Control. 2025;167:110771. doi: 10.1016/j.foodcont.2024.110771
- Cao X, Chen C, Zhu Q. Biosensors based on functional nucleic acids and isothermal amplification techniques. Talanta. 2023;253:123977. doi: 10.1016/j.talanta.2022.123977
- Liu D, Zhu Y, Li N, et al. A portable microfluidic analyzer for integrated bacterial detection using visible loop-mediated amplification. Sens Actuators B. 2020; 310:127834. doi: 10.1016/j.snb.2020.127834
- Lu Y, Zhang J, Lu X and Liu Q. Isothermal nucleic acid amplification based microfluidic “lab-on-a-chip” for the detection of pathogenic bacteria and viruses in agri-foods. Trends Food Sci Technol. 2024;148:104482. doi: 10.1016/j.tifs.2024.104482
- Gao D, Guo X, Yang Y, et al. Microfluidic chip and isothermal amplification technologies for the detection of pathogenic nucleic acid. J Biol Eng. 2022;16(1):33. doi: 10.1186/s13036-022-00312-w
- Kartsova LA, Makeeva DV, Kravchenko AV, Moskvichev D O. Polikarpova DA. Capillary electrophoresis as a powerful tool for the analyses of bacterial samples. Trends Anal Chem. 2021;134:116110. doi: 10.1016/j.trac.2020.116110
- Lian DS, Zeng HS. Capillary electrophoresis based on nucleic acid detection as used in food analysis. Compr Rev Food Sci Food Saf. 2017;16(6):1281-1295. doi: 10.1111/1541-4337.12297
- Okuda K I, Yoshii Y, Yamada S, et al. Detection of bacterial DNA from central venous catheter removed from patients by next generation sequencing: a preliminary clinical study. Ann Clin Microbiol Antimicrob. 2018;17(1):44. doi: 10.1186/s12941-018-0297-2
- Song M, Han C, Liu L, et al. MIST: a microbial identification and source tracking system for next‐generation sequencing data. iMeta. 2023;2(4):e146. doi: 10.1002/imt2.146
- Xia H, Zhang Z, Luo C, et al. MultiPrime: a reliable and efficient tool for targeted next-generation sequencing. iMeta. 2023;2(4):e143. doi: 10.1002/imt2.143
- Botan A, Campisciano G, Zerbato V, et al. Performance of 16S rRNA gene next-generation sequencing and the culture method in the detection of bacteria in clinical specimens. Diagnostics. 2024;14(13):1318. doi: 10.3390/diagnostics14131318
- Zhang M, Wu J, Shi Z, et al. Molecular methods for identification and quantification of foodborne pathogens. Molecules. 2022;27(23):8262. doi: 10.3390/molecules27238262
- Yin G, Bie S, Gu H, et al. Application of gene chip technology in the diagnostic and drug resistance detection of Helicobacter pylori in children. J Gastroenterol Hepatol. 2020;35(8):1331-1339. doi: 10.1111/jgh.14980
- Ding Q, Zhang W, Guo Y, et al. β-lactamase sensitive probe for rapid detection of antibiotic-resistant bacteria with gas chromatography-tandem mass spectrometry. Anal Chem. 2023;95(14):6098-6106. doi: 10.1021/acs.analchem.3c00381
- Olajide OE, Yi Y, Zheng J, Hamid AM. Strain-level discrimination of bacteria by liquid chromatography and paper spray ion mobility mass spectrometry. J Am Soc Mass Spectrom. 2023;34(6):1125-1135. doi: 10.1021/jasms.3c00070
- Boardman AK, Wong WS, Premasiri WR, et al. Rapid detection of bacteria from blood with surface-enhanced raman spectroscopy. Anal Chem. 2016;88(16): 8026-8035. doi: 10.1021/acs.analchem.6b01273
- Bullock A J, Garcia M, Shepherd J, Rehman I, Sheila M. Bacteria induced pH changes in tissue-engineered human skin detected non-invasively using Raman confocal spectroscopy. Appl Spectrosc Rev. 2019;55(2);158-171. doi: 10.1080/05704928.2018.1558232
- Usman M, Tang J W, Li F, et al. Recent advances in surface enhanced Raman spectroscopy for bacterial pathogen identifications. J Adv Res. 2023;51:91-107. doi: 10.1016/j.jare.2022.11.010
- Zhu J, Wu P, Chao Y, et al. Recent advances in 3D printing for catalytic applications. Chem Eng J. 2022;433:134341. doi: 10.1016/j.cej.2021.134341
- Liu N, Zhang X, Guo Q, Wu T, Wang Y. 3D bioprinted scaffolds for tissue repair and regeneration. Front Mater. 2022;9:925321. doi: 10.3389/fmats.2022.925321
- Aimar A, Palermo A, Innocenti B. The role of 3D printing in medical applications: a state of the art. J Healthc Eng. 2019;2019(10):5340616. doi: 10.1155/2019/5340616
- Cui M, Pan H, Su Y, et al. Opportunities and challenges of three-dimensional printing technology in pharmaceutical formulation development. Acta Pharm Sin B. 2021;11(8):2488-2504. doi: 10.1016/j.apsb.2021.03.015
- Nadagouda MN, Rastogi V, Ginn M. A review on 3D printing techniques for medical applications. Curr Opin Chem Eng. 2020;28:152-157. doi: 10.1016/j.coche.2020.05.007
- Alami A H, Ghani Olabi A, Alashkar A, et al. Additive manufacturing in the aerospace and automotive industries: recent trends and role in achieving sustainable development goals. Ain Shams Eng J. 2023;14(11):102516. doi: 10.1016/j.asej.2023.102516
- Bici A and Yunitsyna A. Analysis of 3D printing techniques for building construction: a review. Constr Robot. 2023;7(2):107-123. doi: 10.1007/s41693-023-00108-4
- Hussain S, Malakar S, Arora VK. Extrusion-based 3D food printing: technological approaches, material characteristics, printing stability, and post-processing. Food Eng. Rev. 2022;14(1):100-119. doi: 10.1007/s12393-021-09293-w
- Wang S, Zhao S, Yu J, Gu Z, Zhang Y. Advances in translational 3D printing for cartilage, bone, and osteochondral tissue engineering. Small. 2022;18(36):e2201869. doi: 10.1002/smll.202201869
- Cresswell-Boyes AJ, Davis GR, Krishnamoorthy M, Mills D, Barber AH. Composite 3D printing of biomimetic human teeth. Sci Rep. 2022;12(1):7830. doi: 10.1038/s41598-022-11658-y
- Vernon MJ, Mela P, Dilley RJ, et al. 3D printing of heart valves. Trends Biotechnol. 2024;42(5):612-630. doi: 10.1016/j.tibtech.2023.11.001
- Huang G, Zhao Y, Chen D, et al. Applications, advancements, and challenges of 3D bioprinting in organ transplantation. Biomater Sci. 2024;12(6):1425-1448. doi: 10.1039/d3bm01934a
- Herzog J, Franke L, Lai Y, et al. 3D bioprinting of microorganisms: principles and applications. Bioprocess Biosyst Eng. 2024;47(4):443-461. doi: 10.1007/s00449-023-02965-3
- Fu Z, Naghieh S, Xu C, et al. Printability in extrusion bioprinting. Biofabrication. 2021;13(3):033001. doi: 10.1088/1758-5090/abe7ab
- Bartolo P, Malshe A, Ferraris E, Koc B. 3D bioprinting: materials, processes, and applications. CIRP Ann. 2022;71(2):577-597. doi: 10.1016/j.cirp.2022.06.001
- Ng WL, Shkolnikov V. Jetting-based bioprinting: process, dispense physics, and applications. Bio-Des Manuf. 2024;7(5):771-799. doi: 10.1007/s42242-024-00285-3
- Choudary R, Saini N, Chopra DS, Singh D, Singh N. A comprehensive review of 3D bioprinting biomaterials: properties, strategies and wound healing application. J Mater Res. 2023;38(13):3264-3300. doi: 10.1557/s43578-023-01078-7
- McMillan A, McMillan N, Gupta N, Kanotra S P, Salem AK. 3D bioprinting in otolaryngology: a review. Adv Healthcare Mater. 2023;12(19):e2203268. doi: 10.1002/adhm.202203268
- Jentsch S, Nasehi R, Kuckelkorn C, et al. Multiscale 3D bioprinting by nozzle-free acoustic droplet ejection. Small Methods. 2021;5(6):e2000971. doi: 10.1002/smtd.202000971
- Ng WL, Lee JM, Yeong WY, Win Naing M. Microvalve-based bioprinting - process, bio-inks and applications. Biomater Sci. 2017;5(4):632-647. doi: 10.1039/c6bm00861e
- Li Y, Zhang X, Zhang X, Zhang Y, Hou D. Recent progress of the vat photopolymerization technique in tissue engineering: a brief review of mechanisms, methods, materials, and applications. Polymers. 2023;15(19):3940. doi: 10.3390/polym15193940
- Frankowski J, Kurzatkowska M, Sobczak M, Piotrowska U. Utilization of 3D bioprinting technology in creating human tissue and organoid models for preclinical drug research— State-of-the-art. Int J Pharm. 2023;644(11):123313. doi: 10.1016/j.ijpharm.2023.123313
- Seo JW, Kim GM, Choi Y, Cha JM, Bae H. Improving printability of digital-light-processing 3D bioprinting via photoabsorber pigment adjustment. Int J Mol Sci. 2022;23(10):5428. doi: 10.3390/ijms23105428
- Xu HQ, Liu JC, Zhang ZY, Xu CX. A review on cell damage, viability, and functionality during 3D bioprinting. Mil Med Res. 2022;9(1):70. doi: 10.1186/s40779-022-00429-5
- Lepowsky E, Muradoglu M, Tasoglu S. Towards preserving post-printing cell viability and improving the resolution: past, present, and future of 3D bioprinting theory. Bioprinting. 2018;11:e00034. doi: 10.1016/j.bprint.2018.e00034
- Wang J, Cui Z, Maniruzzaman M. Bioprinting: a focus on improving bioink printability and cell performance based on different process parameters. Int J Pharm. 2023;640:123020. doi: 10.1016/j.ijpharm.2023.123020
- Zhao T, Liu Y, Wu Y, Zhao M, Zhao Y. Controllable and biocompatible 3D bioprinting technology for microorganisms: fundamental, environmental applications and challenges. Biotechnol Adv. 2023;69(2):108243. doi: 10.1016/j.biotechadv.2023.108243
- Prabhakaran P, Palaniyandi T, Kanagavalli B, et al. Prospect and retrospect of 3D bio-printing. Acta Histochem. 2022;124(7):151932. doi: 10.1016/j.acthis.2022.151932
- Gungor-Ozkerim PS, Inci I, Zhang Y S, Khademhosseini A, Dokmeci MR. Bioinks for 3D bioprinting: an overview. Biomater Sci. 2018;6(5):915-946. doi: 10.1039/c7bm00765e
- Jia W, Yang X, Liu Z, et al. Nasal cartilage tissue engineering materials based on 3D bioprinting: seed cells and dECM. Appl Mater Today. 2024;40(1):102364. doi: 10.1016/j.apmt.2024.102364
- Sasikumar SC, Goswami U, Raichur AM. 3D bioprinting with visible light cross-linkable mucin-hyaluronic acid composite bioink for lung tissue engineering. ACS Appl Bio Mater. 2024;7(8):5411-5422. doi: 10.1021/acsabm.4c00579
- Breathwaite E, Weaver J, Odanga J, Dela Pena-Ponce M, Lee JB. 3D bioprinted osteogenic tissue models for in vitro drug screening. Molecules. 2020;25(15):3442. doi: 10.3390/molecules25153442
- Salles GR, Dall’Agnol Ferreira N, Pacheco‐Soares C, Porcionatto MA. 3D bioprinted human neuron‐like spheroids as an in vitro model for neurodegenerative diseases. Alzheimers Dement. 2023;19(S13):e075956. doi: 10.1002/alz.075956
- Chua CK, An J, Fan S, et al. A perspective on transformative bioprinting. Int J Bioprint. 2024. doi: 10.36922/ijb.3525
- Mori S, Ishiguro S, Miyazaki S, et al. Usefulness of a 3D-printing air sampler for capturing live airborne bacteria and exploring the environmental factors that can influence bacterial dynamics. Res Microbiol. 2021;172(6):103864. doi: 10.1016/j.resmic.2021.103864
- Joskowiak A, Nogueira CL, Costa SP, et al. A magnetic nanoparticle-based microfluidic device fabricated using a 3D-printed mould for separation of Escherichia coli from blood. Microchim Acta. 2023;190(9):356. doi: 10.1007/s00604-023-05924-7
- Lee W, Kwon D, Choi W, Jung GY, Jeon S. 3D-printed microfluidic device for the detection of pathogenic bacteria using size-based separation in helical channel with trapezoid cross-section. Sci Rep. 2015;5(1):7717. doi: 10.1038/srep07717
- Pahlow S, Hentschel S, Horbert P, et al. Isolation of pathogenic bacteria from sputum samples using a 3D-printed cartridge system. Anal Methods. 2021;13(41):4884-4895. doi: 10.1039/d1ay00924a
- Feng S, Mo K, Song X. 3D printed microfluidic chip integrated with nanointerferometer for multiplex detection of foodborne pathogens. AIP Adv. 2024;14(6):065033. doi: 10.1063/5.0208274
- Malhotra S, Pham DS, Lau MPH, Nguyen AH, Cao H. A low-cost, 3D-printed biosensor for rapid detection of Escherichia coli. Sensors. 2022;22(6):2382. doi: 10.3390/s22062382
- Aliyazdi S, Frisch S, Hidalgo A, et al. 3D bioprinting of E. coli MG1655 biofilms on human lung epithelial cells for building complexin vitroinfection models.Biofabrication. 2023;15(3):035019. doi: 10.1088/1758-5090/acd95e
- Xu Y, Zhang Q, Li Y, Pang X, Cheng N. A 3D-printed integrated handheld biosensor for the detection of vibrio parahaemolyticus. Foods. 2024;13(11):1775. doi: 10.3390/foods13111775
- Angelopoulou M, Kourti D, Mertiri M, et al. A 3D-printed electrochemical immunosensor employing Cd/Se ZnS QDs as labels for the rapid and ultrasensitive detection of Salmonella typhimurium in poultry samples. Chemosensors. 2023;11(9):475. doi: 10.3390/chemosensors11090475
- Yin P, Wang J, Li T, et al. A smartphone-based fluorescent sensor for rapid detection of multiple pathogenic bacteria. Biosens Bioelectron. 2023;242(3):115744. doi: 10.1016/j.bios.2023.115744
- Zhang T, Monia Kabandana GK, Ratajczak AM, Chen C. A quantitative sensing system based on a 3D-printed ion-selective electrode for rapid and sensitive detection of bacteria in biological fluid. Talanta. 2022;238(Pt 2):123040. doi: 10.1016/j.talanta.2021.123040
- Panda S, Hajra S, Kim HG, et al. Carbohydrate-protein interaction-based detection of pathogenic bacteria using a biodegradable self-powered biosensor. J Mater Chem B. 2023;11(42):10147-10157. doi: 10.1039/d3tb01820b
- Park C, Lee J, Kim Y, et al. 3D-printed microfluidic magnetic preconcentrator for the detection of bacterial pathogen using an ATP luminometer and antibody-conjugated magnetic nanoparticles. J Microbiol Methods. 2017;132:128-133. doi: 10.1016/j.mimet.2016.12.001
- Kim Y, Lee J, Park S. A 3D-printed millifluidic platform enabling bacterial preconcentration and DNA purification for molecular detection of pathogens in blood. Micromachines. 2018;9(9):472. doi: 10.3390/mi9090472
- Yin K, Ding X, Xu Z, et al. Multiplexed colorimetric detection of SARS-CoV-2 and other pathogens in wastewater on a 3D printed integrated microfluidic chip. Sens Actuators B. 2021;344:130242. doi: 10.1016/j.snb.2021.130242
- Srikanth S, Jayapiriya US, Dubey SK, Javed A, Goel S. A lab-on-chip platform for simultaneous culture and electrochemical detection of bacteria. iScience. 2022;25(11):105388. doi: 10.1016/j.isci.2022.105388
- Dey P, Fabri-Faja N, Calvo-Lozano O, et al. Label-free bacteria quantification in blood plasma by a bioprinted microarray based interferometric point-of-care device. ACS Sens. 2019;4(1):52-60. doi: 10.1021/acssensors.8b00789
- Al-Taie A, Han X, Williams CM, et al. 3-D printed polyvinyl alcohol matrix for detection of airborne pathogens in respiratory bacterial infections. Microbiol Res. 2020;241(20):126587. doi: 10.1016/j.micres.2020.126587
- Sharma A, Sharma A, Malhotra R, et al. An accurate artificial intelligence system for the detection of pulmonary and extra pulmonary Tuberculosis. Tuberculosis. 2021;131: 102143. doi: 10.1016/j.tube.2021.102143
- Nehal SA, Roy D, Devi M, Srinivas T. Highly sensitive lab-on-chip with deep learning AI for detection of bacteria in water. Int J Inf Technol. 2019;12(2):495-501. doi: 10.1007/s41870-019-00363-1
- Cui R, Tang H, Huang Q, et al. AI-assisted smartphone-based colorimetric biosensor for visualized, rapid and sensitive detection of pathogenic bacteria. Biosens Bioelectron. 2024;259(8):116369. doi: 10.1016/j.bios.2024.116369
- Safir F, Vu N, Tadesse LF, et al. Combining acoustic bioprinting with AI-assisted Raman spectroscopy for high-throughput identification of bacteria in blood. Nano Lett. 2023;23(6):2065-2073. doi: 10.1021/acs.nanolett.2c03015
- Law JW-F, Ab Mutalib N-S, Chan K-G, Lee L-H. Rapid methods for the detection of foodborne bacterial pathogens: principles, applications, advantages and limitations. Front Microbiol. 2015;5(770):770. doi: 10.3389/fmicb.2014.00770
- Kim SO, Kim SS. Bacterial pathogen detection by conventional culture‐based and recent alternative (polymerase chain reaction, isothermal amplification, enzyme linked immunosorbent assay, bacteriophage amplification, and gold nanoparticle aggregation) methods in food samples: a review. J Food Saf. 2020;41(1):e12870. doi: 10.1111/jfs.12870