3D-printed artificial cornea featuring aligned fibrous structure and enhanced mechanical strength
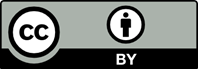
The global shortage of donor eye bank tissue has significantly impeded advancements in biomaterial for corneal implantation. To address this issue, we have developed a three-dimensional (3D)-printed artificial cornea using a composite scaffolds of sodium alginate (SA) and cellulose nanofibers (CNF), crosslinked with poly-L-lysine-co-L-glutamic acid (PLL80GA20, PG) and calcium chloride (CaCl2, CC). The 2 wt% SA/ CNF composite scaffolds offer several advantages, including low toxicity, cost-effectiveness, excellent printability, and high mechanical strength, even with low crosslinker concentrations. The PG was synthesized via ring-opening polymerization of L-glutamate N-carboxyl anhydride (BGNCA) and L-lysine N-carboxyl anhydride (CBZNCA). The purity of the monomers was verified through differential scanning calorimetry analysis, revealing a melting point of 97°C. The molecular weight of the synthesized PG was determined to be 47 kDa. A dual crosslinking strategy was employed, starting with electrostatic crosslinking, followed by ionic crosslinking using varying concentrations of PG and CC at different effective charge concentrations of 6.25 12.25, and 25 mM.The hydrogel and 3D-printed cornea were comprehensively evaluated for chemical structure, surface functional groups, water content, mechanical strength, orientation, cytotoxicity, biocompatibility, and transparency. Notably, the inclusion of PG significantly enhanced the mechanical properties of the 3D-printed cornea, with the hydrogel achieving a storage modulus of 2,360 kPa at 6.25 mM of PG/CC, while maintaining over 95% water content. The artificial cornea demonstrated 86% transparency, and the cell viability showed 96% viable on day 7 with degradation rate of 35.9% in 28 days. The superior hydrophilicity, transparency, and mechanical strength of the printed scaffolds highlights their potential for the development of full-thickness corneal structures, making them a promising candidate for future corneal implants.

- Kong B, Chen Y, Liu R, et al. Fiber reinforced GelMA hydrogel to induce the regeneration of corneal stroma. Nat Commun. 2020;11(1):1435. doi: 10.1038/s41467-020-14887-9.
- Ahn J-I, Kuffova L, Merrett K, et al. Crosslinked collagen hydrogels as corneal implants: effects of sterically bulky vs. non-bulky carbodiimides as crosslinkers. Acta biomaterialia. 2013;9(8):7796-7805. doi: 10.1016/j.actbio.2013.04.014.
- Griffith M, Hakim M, Shimmura S, et al. Artificial human corneas: scaffolds for transplantation and host regeneration. Cornea. 2002;21:S54-S61. doi: 10.1097/01.ico.0000263120.68768.f8.
- Gain P, Jullienne R, He Z, et al. Global survey of corneal transplantation and eye banking. JAMA ophthalmology. 2016;134(2):167-173. doi: 10.1001/jamaophthalmol.2015.4776.
- Bentley E, Murphy CJ, Li F, Carlsson DJ, Griffith M. Biosynthetic corneal substitute implantation in dogs. Cornea. 2010;29(8):910-916. doi: 10.1097/ICO.0b013e3181c846aa.
- Liu L, Kuffová L, Griffith M, et al. Immunological responses in mice to full-thickness corneal grafts engineered from porcine collagen. Biomaterials. 2007;28(26):3807-3814. doi: 10.1016/j.biomaterials.2007.04.025.
- San Choi J, Williams JK, Greven M, et al. Bioengineering endothelialized neo-corneas using donor-derived corneal endothelial cells and decellularized corneal stroma. Biomaterials. 2010;31(26):6738-6745. doi: 10.1016/j.biomaterials.2010.05.020.
- Armitage WJ, Goodchild C, Griffin MD, et al. High-risk corneal transplantation: recent developments and future possibilities. Transplantation. 2019;103(12):2468-2478. doi: 10.1097/TP.0000000000002938.
- Grigoryan B, Paulsen SJ, Corbett DC, et al. Multivascular networks and functional intravascular topologies within biocompatible hydrogels. Science. 2019;364(6439):458-464. doi: 10.1126/science.aav9750
- Orash Mahmoud Salehi A, Heidari-Keshel S, Poursamar SA, et al. Bioprinted membranes for corneal tissue engineering: a review. Pharmaceutics. 2022;14(12):2797. doi: 10.3390/pharmaceutics14122797.
- Alam F, Elsherif M, AlQattan B, et al. 3D printed contact lenses. ACS Biomater Sci Eng. 2021;7(2):794-803. doi: 10.1021/acsbiomaterials.0c01470
- Alam F, Alsharif A, AlModaf FO, El-Atab N. 3D-printed smartwatch fabricated via vat photopolymerization for UV and temperature sensing applications. ACS Omega. 2024;9(13):14830-14839. doi: 10.1021/acsomega.3c07411.
- Bernal PN, Delrot P, Loterie D, et al. Volumetric bioprinting of complex living‐tissue constructs within seconds. Adv Mater. 2019;31(42):1904209. doi: 10.1002/adma.201904209
- Patel AP, Wu EI, Ritterband DC, Seedor JA. Boston type 1 keratoprosthesis: the New York Eye and Ear experience. Eye (Lond). 2012;26(3):418-425. doi: 10.1038/eye.2011.325
- Zhang X, Zhang X, Li Y, Zhang Y, et. Al. Applications of Light-Based 3D Bioprinting and Photoactive Biomaterials for Tissue Engineering. Materials. 2023;16(23):7461 doi: 10.3390/ma16237461
- Riau AK, Venkatraman SS, Dohlman CH, Mehta JS. Surface modifications of the PMMA optic of a keratoprosthesis to improve biointegration. Cornea. 2017;36:S15-S25. doi: 10.1097/ICO.0000000000001352
- Ma T-L, Yang S-C, Cheng T, et al. Exploration of biomimetic poly (γ-benzyl-l-glutamate) fibrous scaffolds for corneal nerve regeneration. J Mater Chemy B. 2022;10(33): 6372-6379. doi: 10.1038/s41536-017-0038-8
- Islam MM, Buznyk O, Reddy JC, et al. Biomaterials-enabled cornea regeneration in patients at high risk for rejection of donor tissue transplantation. NPJ Regen Med. 2018;3(1):2. doi: 10.1038/s41536-017-0038-8
- Mimura T, Amano S, Yokoo S, et al. Tissue engineering of corneal stroma with rabbit fibroblast precursors and gelatin hydrogels. Mol Vis. 2008;14:1819.
- Crabb RA, Chau EP, Evans MC, Barocas VH, Hubel A. Biomechanical and microstructural characteristics of a collagen film-based corneal stroma equivalent. Tissue Eng. 2006;12(6):1565-1575. doi: 10.1089/ten.2006.12.1565
- Lawrence BD, Marchant JK, Pindrus MA, Omenetto FG, Kaplan DL. Silk film biomaterials for cornea tissue engineering. Biomaterials. 2009;30(7):1299-1308. doi: 10.1016/j.biomaterials.2008.11.018
- Gouveia RM, González-Andrades E, Cardona JC, et al. Controlling the 3D architecture of Self-Lifting Auto-generated Tissue Equivalents (SLATEs) for optimized corneal graft composition and stability. Biomaterials. 2017;121:205-219. doi: 10.1016/j.biomaterials.2016.12.023.
- Wang S, Ghezzi CE, Gomes R, Pollard RE, Funderburgh JL, Kaplan DL. In vitro 3D corneal tissue model with epithelium, stroma, and innervation. Biomaterials. 2017;112:1-9. doi: 10.1016/j.biomaterials.2016.09.030
- Dong H, Snyder JF, Williams KS, Andzelm JW. Cation-induced hydrogels of cellulose nanofibrils with tunable moduli. Biomacromolecules. 2013;14(9):3338-3345. doi: 10.1021/bm400993f
- Yang J, Han C-R, Duan J-F, et al. Studies on the properties and formation mechanism of flexible nanocomposite hydrogels from cellulose nanocrystals and poly (acrylic acid). J Mater Chem. 2012;22(42):22467-22480. doi: 10.1039/C2JM35498E
- Yu TY, Tseng YH, Wang CC, et al. Three‐level hierarchical 3D network formation and structure elucidation of wet hydrogel of tunable‐high‐strength nanocomposites. Macromol Mater Eng. 2022;307(5):2100871. doi: 10.1002/mame.202100871
- Rafat M, Li F, Fagerholm P, et al. PEG-stabilized carbodiimide crosslinked collagen–chitosan hydrogels for corneal tissue engineering. Biomaterials. 2008;29(29):3960-3972. doi: 10.1016/j.biomaterials.2008.06.017
- Wang J, Gao C, Zhang Y, Wan Y. Preparation and in vitro characterization of BC/PVA hydrogel composite for its potential use as artificial cornea biomaterial. Mater Sci Eng C. 2010;30(1):214-218. doi: 10.1016/j.msec.2009.10.006
- Brunette I, Roberts CJ, Vidal F, et al. Alternatives to eye bank native tissue for corneal stromal replacement. Prog Retin Eye Res. 2017;59:97-130. doi: 10.1016/j.preteyeres.2017.04.002
- Nie X, Tang Y, Wu T, et al. 3D printing sequentially strengthening high-strength natural polymer hydrogel bilayer scaffold for cornea regeneration. Regen Biomater. 2024;11:rbae012.
- Romo-Valera C, Guerrero P, Arluzea J, Etxebarria J, de la Caba K, Andollo N. Cytocompatibility and suitability of protein-based biomaterials as potential candidates for corneal tissue engineering. Int J Mol Sci. 2021;22(7):3648.
- Liu J, Huang Y, Yang W, et al. Sutureless transplantation using a semi-interpenetrating polymer network bioadhesive for ocular surface reconstruction. Acta Biomater. 2022;153:273-286. doi: 10.1016/j.actbio.2022.09.049
- Rastogi P, Kandasubramanian B. Review of alginate-based hydrogel bioprinting for application in tissue engineering. Biofabrication. 2019;11(4):042001. doi: 10.1088/1758-5090/ab331e
- Logan CM, Fernandes-Cunha GM, Chen F, et al. In situ-forming collagen hydrogels crosslinked by multifunctional polyethylene glycol as a matrix therapy for corneal defects: 2-month follow-up in vivo. Cornea. 2023;42(1):97-104. doi: 10.1097/ICO.0000000000003104
- Raus RA, Nawawi WMFW, Nasaruddin RR. Alginate and alginate composites for biomedical applications. Asian J Pharm Sci. 2021;16(3):280-306. doi: 10.1016/j.ajps.2020.10.001
- Zarrintaj P, Ghorbani S, Barani M, et al. Polylysine for skin regeneration: a review of recent advances and future perspectives. Bioeng Transl Med. 2022;7(1):e10261. doi: 10.1002/btm2.10261
- Wang L, Zhang HJ, Liu X, et al. A physically cross-linked sodium alginate–gelatin hydrogel with high mechanical strength. ACS Appl Polym Mater. 2021;3(6):3197-3205. doi: 10.1021/acsapm.1c00404
- Reddy MSB, Ponnamma D, Choudhary R, Sadasivuni KK. A comparative review of natural and synthetic biopolymer composite scaffolds. Polymers. 2021;13(7):1105. doi: 10.3390/polym13071105
- Yue X, Deng W, Zhou Z, Xu Y, He J, Wang Z. Reinforced and flame retarded cellulose nanofibril/sodium alginate compound aerogel fabricated via boric acid/Ca2+ double cross-linking. J Polym Environ. 2023;31(3):1038-1050.
- Zander ZK, Hua G, Wiener CG, Vogt BD, Becker ML. Control of mesh size and modulus by kinetically dependent cross-linking in hydrogels. Adv Mater. 2015;27(40):6283. doi: 10.1002/adma.201501822
- Kim S-S, Park MS, Jeon O, Choi CY, Kim B-S. Poly (lactide-co-glycolide)/hydroxyapatite composite scaffolds for bone tissue engineering. Biomaterials. 2006;27(8):1399-1409. doi: 10.1016/j.biomaterials.2005.08.016
- 42 Donnaloja F, Jacchetti E, Soncini M, Raimondi MT. Natural and synthetic polymers for bone scaffolds optimization. Polymers. 2020;12(4):905. doi: 10.3390/polym12040905
- Cui Y, Liu Y, Cui Y, Jing X, Zhang P, Chen X. The nanocomposite scaffold of poly (lactide-co-glycolide) and hydroxyapatite surface-grafted with L-lactic acid oligomer for bone repair. Acta Biomater. 2009;5(7):2680-2692. doi: 10.1016/j.actbio.2009.03.024
- Wang S, Li T, Chen C, et al. Transparent, anisotropic biofilm with aligned bacterial cellulose nanofibers. Adv Funct Mater. 2018;28(24):1707491. doi: 10.1002/adfm.201707491
- Wang X, Majumdar S, Soiberman U, et al. Multifunctional synthetic Bowman’s membrane-stromal biomimetic for corneal reconstruction. Biomaterials. 2020;241:119880. doi: 10.1016/j.biomaterials.2020.119880
- Kong B, Sun W, Chen G, et al. Tissue-engineered cornea constructed with compressed collagen and laser-perforated electrospun mat. Sci Rep. 2017;7(1):970. doi: 10.1038/s41598-017-01072-0.
- Majumdar S, Wang X, Sommerfeld SD, et al. Cyclodextrin modulated type I collagen self‐assembly to engineer biomimetic cornea implants. Adv Funct Mater. 2018;28(41):1804076. doi: 10.1002/adfm.201804076
- Lei X, Jia Y-G, Song W, et al. Mechanical and optical properties of reinforced collagen membranes for corneal regeneration through polyrotaxane cross-linking. ACS Appl Bio Mater. 2019;2(9):3861-3869. doi: 10.1021/acsabm.9b00464
- Zhang J, Sisley AM, Anderson AJ, Taberner AJ, McGhee CN, Patel DV. Characterization of a novel collagen scaffold for corneal tissue engineering. Tissue Eng Part C Methods. 2016;22(2):165-172. doi: 10.1089/ten.TEC.2015.0304
- Long Y, Zhao X, Liu S, et al. Collagen–hydroxypropyl methylcellulose membranes for corneal regeneration. ACS Omega. 2018;3(1):1269-1275. doi: 10.1021/acsomega.7b01511
- Fernandes-Cunha GM, Chen KM, Chen F, et al. In situ-forming collagen hydrogel crosslinked via multi-functional PEG as a matrix therapy for corneal defects. Sci Rep. 2020;10(1):16671. doi: 10.1038/s41598-020-72978-5
- Lei M, Zhang S, Zhou H, et al. Electrical signal initiates kinetic assembly of collagen to construct optically transparent and geometry customized artificial cornea substitutes. ACS Nano. 2022;16(7):10632-10646. doi: 10.1021/acsnano.2c02291
- Mallet JD, Rochette PJ. Wavelength-dependent ultraviolet induction of cyclobutane pyrimidine dimers in the human cornea. Photochem Photobiol Sci. 2013;12(8):1310-1318. doi: 10.1039/c3pp25408a.
- Paul A, Eun C-J, Song JM. Cytotoxicity mechanism of non-viral carriers polyethylenimine and poly-l-lysine using real time high-content cellular assay. Polymer. 2014;55(20):5178-5188. doi: 10.1016/j.polymer.2014.08.043
- Arnold Jr L, Dagan A, Gutheil J, Kaplan N. Antineoplastic activity of poly (L-lysine) with some ascites tumor cells. Proc Natl Acad Sci. 1979;76(7):3246-3250.
- Liu S, Huang D, Hu Y, et al. Sodium alginate/collagen composite multiscale porous scaffolds containing poly (ε-caprolactone) microspheres fabricated based on additive manufacturing technology. RSC Adv. 2020;10(64):39241-39250. doi: 10.1039/d0ra04581k
- Formisano N, van der Putten C, Grant R, et al. Mechanical properties of bioengineered corneal stroma. Adv Healthc Mater. 2021;10(20):2100972. doi: 10.1002/adhm.202100972.