Scaling liver bioprinting: A guide for usage of the hepatic extracellular matrix as a bioink
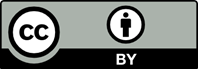
Hydrogels derived from the decellularized extracellular matrix (dECM) are widely used in three-dimensional (3D) bioprinting, mainly because they recapitulate the native tissue microenvironment and retain key growth factors and cytokines. Hence, they are characterized by adequate biocompatibility for use in 3D bioprinting. In liver tissue engineering, these materials, along with liver-derived cell types, can serve as appropriate in vitro hepatic models for drug efficiency testing and liver metabolism studies. These hydrogels can also be considered as good manufacturing practice-compliant systems for liver cell and organoid expansion, unlike routinely used basal membrane extract products derived from tumorigenic cell lines. Although weak mechanical properties and poor printability hinder the direct usage of dECM hydrogels as bioinks, various modifications of dECM and the bioprinting process are applied to overcome these problems. However, there are several complications regarding the scale-up and good laboratory practice-compliant manufacturing of these hydrogels: (i) the manufacturing standards for dECM hydrogels are not well established; (ii) the methods for obtaining these hydrogels are slightly varying, resulting in decreased reproducibility; and (iii) since these hydrogels are traditionally produced from animal tissue, the animal-to-animal variability, different harvesting conditions, and bioburden reduction need to be thoroughly considered. This review examines the essential properties of dECM hydrogels for biomedical applications, focusing on biocompatibility, mechanical strength, and bioactivity. Additionally, this review discusses production methods and modifications for 3D bioprinting, highlights case studies of dECM-based liver constructs, and addresses challenges in scalability and regulatory hurdles for clinical translation.

- Kaur S, Kaur I, Rawal P, Tripathi DM, Vasudevan A. Non-matrigel scaffolds for organoid cultures. Cancer Lett. 2021;504:58-66. doi: 10.1016/j.canlet.2021.01.025
- Bustamante-Torres M, Romero-Fierro D, Arcentales-Vera B, Palomino K, Magaña H, Bucio E. Hydrogels classification according to the physical or chemical interactions and as stimuli-sensitive materials. Gels. 2021;7(4):182. doi: 10.3390/gels7040182
- Chaplenko AA, Khorolsky MD, Melnikova EV, Merkulov VA. Current trends in the use of cell therapy in regenerative medicine. Bio Prod Prev Diag Treat. 2020;20(2):82–88. doi: 10.30895/2221-996X-2020-20-2-82-88
- Ruoß M, Vosough M, Königsrainer A, et al. Towards improved hepatocyte cultures: Progress and limitations. Food Chem Toxicol. 2020;138:111188. doi: 10.1016/j.fct.2020.111188
- Heydari Z, Zarkesh I, Ghanian MH, et al. Biofabrication of size-controlled liver microtissues incorporated with ECM-derived microparticles to prolong hepatocyte function. Bio-des Manuf. 2021;4:790-805. doi: 10.1007/s42242-021-00137-4
- Bikmulina P, Kosheleva N, Efremov Yu, et al. Building a tissue: gingiva- and adipose-derived mesenchymal cell spheroids’ survivability and functionality after 3D extrusion bioprinting. Bioprinting. 2023;32: e00279. doi: 10.1016/j.bprint.2023.e00279.
- Mirdamadi ES, Kalhori D, Zakeri N, Azarpira N, Solati- Hashjin M. Liver tissue engineering as an emerging alternative for liver disease treatment. Tissue Eng Part B Rev. 2020;26(2):145-163. doi: 10.1089/ten.TEB.2019.0233
- Ergun C, Parmaksiz M, Vurat MT, Elçin AE, Elçin YM. Decellularized liver ECM-based 3D scaffolds: compositional, physical, chemical, rheological, thermal, mechanical, and in vitro biological evaluations. Int J Biol Macromol. 2022;200:110-123. doi: 10.1016/j.ijbiomac.2021.12.086
- Mao Q, Wang Y, Li Y, et al. Fabrication of liver microtissue with liver decellularized extracellular matrix (dECM) bioink by digital light processing (DLP) bioprinting. Mater Sci Eng C Mater Biol Appl. 2020;109:110625. doi: 10.1016/j.msec.2020.110625
- Heydari Z, Najimi M, Mirzaei H, et al. Tissue engineering in liver regenerative medicine: insights into novel translational technologies. Cells. 2020;9(2):304. doi: 10.3390/cells9020304
- Ranucci CS, Kumar A, Batra SP, Moghe PV. Control of hepatocyte function on collagen foams: sizing matrix pores toward selective induction of 2-D and 3-D cellular morphogenesis. Biomaterials. 2000;21(8):783-793. doi: 10.1016/s0142-9612(99)00238-0
- Kobayashi M, Kadota J, Hashimoto Y, et al. Elastic modulus of ECM hydrogels derived from decellularized tissue affects capillary network formation in endothelial cells. Int J Mol Sci. 2020;21(17):6304. doi: 10.3390/ijms21176304
- Ishihara S, Kurosawa H, Haga H. Stiffness-modulation of collagen gels by genipin-crosslinking for cell culture. Gels. 2023; 9(2):148. doi: 10.3390/gels9020148
- Baptista PM, Siddiqui MM, Lozier G, Rodriguez SR, Atala A, Soker S. The use of whole organ decellularization for the generation of a vascularized liver organoid. Hepatology. 2011;53(2):604-617. doi: 10.1002/hep.24067
- Karamanos NK, Theocharis AD, Piperigkou Z, et al. A guide to the composition and functions of the extracellular matrix. FEBS J. 2021;288(24):6850-6912. doi: 10.1111/febs.15776
- Hussey GS, Pineda Molina C, Cramer MC, et al. Lipidomics and RNA sequencing reveal a novel subpopulation of nanovesicle within extracellular matrix biomaterials. Sci Adv. 2020;6(12):eaay4361. doi: 10.1126/sciadv.aay4361
- Arteel GE, Naba A. The liver matrisome - looking beyond collagens. JHEP Rep. 2020;2(4):100115. doi: 10.1016/j.jhepr.2020.100115
- Rozario T, DeSimone DW. The extracellular matrix in development and morphogenesis: a dynamic view. Dev Biol. 2010;341(1):126-140. doi: 10.1016/j.ydbio.2009.10.026
- Heydari Z., Pooyan P, Bikmulina P, et al. Mimicking the liver function in micro-patterned units: challenges and perspectives in 3D bioprinting. Bioprinting. 2022;27:e00208. doi: 10.1016/J.BPRINT.2022.E00208
- Whiteford JR, Arokiasamy S, De Rossi G. Translating the matrix. Br J Pharmacol. 2019;176(1):3-4. doi: 10.1111/bph.14517
- Kim J, Seki E. Hyaluronan in liver fibrosis: basic mechanisms, clinical implications, and therapeutic targets. Hepatol Commun. 2023;7(4):e0083. doi: 10.1097/HC9.0000000000000083
- Gudowska M, Cylwik B, Chrostek L. The role of serum hyaluronic acid determination in the diagnosis of liver fibrosis. Acta Biochim Pol. 2017;64(3):451-457. doi: 10.18388/abp.2016_1443
- Matsuda M, Seki E. The liver fibrosis niche: Novel insights into the interplay between fibrosis-composing mesenchymal cells, immune cells, endothelial cells, and extracellular matrix. Food Chem Toxicol. 2020;143:111556. doi: 10.1016/j.fct.2020.111556
- Shan L, Wang F, Zhai D, Meng X, Liu J, Lv X. Matrix metalloproteinases induce extracellular matrix degradation through various pathways to alleviate hepatic fibrosis [published correction appears in Biomed Pharmacother. 2023 Jun;162:114632]. Biomed Pharmacother. 2023;161:114472. doi: 10.1016/j.biopha.2023.114472
- Hintermann E, Christen U. The many roles of cell adhesion molecules in hepatic fibrosis. Cells. 2019;8(12):1503. doi: 10.3390/cells8121503
- Freytes DO, O’Neill JD, Duan-Arnold Y, Wrona EA, Vunjak-Novakovic G. Natural cardiac extracellular matrix hydrogels for cultivation of human stem cell-derived cardiomyocytes. Methods Mol Biol. 2014;1181:69-81. doi: 10.1007/978-1-4939-1047-2_7
- Voytik-Harbin SL, Brightman AO. Small intestinal submucosa: a tissue derived extracellular matrix that promotes tissue-specific growth and differentiation of cells in vitro. Tissue Eng. 1998;4:157-174. doi: 10.1089/ten.1998.4.157
- Uriel S, Huang JJ, Moya ML, et al. The role of adipose protein derived hydrogels in adipogenesis. Biomaterials. 2008;29(27):3712-3719. doi: 10.1016/j.biomaterials.2008.05.028
- Kao CY, Nguyen HQ, Weng YC. Characterization of Porcine Urinary Bladder Matrix Hydrogels from Sodium Dodecyl Sulfate Decellularization Method. Polymers (Basel). 2020;12(12):3007. doi: 10.3390/polym12123007
- Simsa R, Rothenbücher T, Gürbüz H, et al. Brain organoid formation on decellularized porcine brain ECM hydrogels. PLoS One. 2021;16(1):e0245685. doi: 10.1371/journal.pone.0245685
- Johnson TD, Lin SY, Christman KL. Tailoring material properties of a nanofibrous extracellular matrix derived hydrogel. Nanotechnology. 2011;22(49):494015. doi: 10.1088/0957-4484/22/49/494015
- Pouliot RA, Young BM, Link PA, et al. Porcine lung-derived extracellular matrix hydrogel properties are dependent on pepsin digestion time. Tissue Eng Part C Methods. 2020;26(6):332-346. doi: 10.1089/ten.TEC.2020.0042
- Uriel S, Labay E, Francis-Sedlak M, et al. Extraction and assembly of tissue-derived gels for cell culture and tissue engineering. Tissue Eng Part C Methods. 2009;15(3):309-321. doi: 10.1089/ten.tec.2008.0309
- Giobbe GG, Crowley C, Luni C, et al. Extracellular matrix hydrogel derived from decellularized tissues enables endodermal organoid culture. Nat Commun. 2019;10(1):5658. doi: 10.1038/s41467-019-13605-4
- Ijima H, Nakamura S, Bual R, Shirakigawa N, Tanoue S. Physical properties of the extracellular matrix of decellularized porcine liver. Gels. 2018;4(2):39. doi: 10.3390/gels4020039
- Hsieh DJ, Srinivasan P. Protocols for accelerated production and purification of collagen scaffold and atelocollagen from animal tissues. Biotechniques. 2020;69(3):220-225. doi: 10.2144/btn-2020-0070
- Hussey GS, Nascari DG, Saldin LT, et al. Ultrasonic cavitation to prepare ECM hydrogels. Acta Biomater. 2020;108:77-86. doi: 10.1016/j.actbio.2020.03.036
- Hulmes DJS. Collagen Diversity, Synthesis, and Assembly. New York: Springer; 2008. doi: 10.1007/978-0-387-73906-9_2
- Kadler KE, Hill A, Canty-Laird EG. Collagen fibrillogenesis: fibronectin, integrins, and minor collagens as organizers and nucleators. Curr Opin Cell Biol. 2008;20(5):495-501. doi: 10.1016/j.ceb.2008.06.008
- Kayabölen A. Development of Vascularized Adipose Tissue Construct Based on Adipose Tissue Extracellular Matrix and Silk Fibroin. M.S. - Master of Science, Middle East Technical University; 2015.
- Stepanovska J, Otahal M, Hanzalek K, Supova M, Matejka R. pH modification of high-concentrated collagen bioinks as a factor affecting cell viability, mechanical properties, and printability. Gels. 2021;7(4):252. doi: 10.3390/gels7040252
- Riacci L, Sorriento A, Ricotti L. Genipin-based crosslinking of jellyfish collagen 3D hydrogels. Gels. 2021;7(4):238. doi: 10.3390/gels7040238
- Utami Nike D, Md Fadilah NI, Sallehuddin N, et al. Genipin-crosslinking effects on biomatrix development for cutaneous wound healing: a concise review. Front Bioeng Biotechnol. 2022;10:865014. doi: 10.3389/fbioe.2022.865014
- Boso D, Carraro E, Maghin E, et al. Porcine decellularized diaphragm hydrogel: a new option for skeletal muscle malformations. Biomedicines. 2021;9(7):709. doi: 10.3390/biomedicines9070709
- Zhu H, Cai X, Wu L, Gu Z. A facile one-step gelation approach simultaneously combining physical and chemical cross-linking for the preparation of injectable hydrogels. J Mater Chem B. 2017;5(17):3145-3153. doi: 10.1039/c7tb00396j
- Cécile E, Laurine V, Jean M, Ahmad M, Gilles S. Chemical cross-linking methods for cell encapsulation in hydrogels. Materials Today Commun. 2019; 20:100536, ISSN 2352-4928. doi: 10.1016/j.mtcomm.2019.05.012
- Fedorovich NE, Oudshoorn MH, van Geemen D, Hennink WE, Alblas J, Dhert WJ. The effect of photopolymerization on stem cells embedded in hydrogels. Biomaterials. 2009;30(3):344-353. doi: 10.1016/j.biomaterials.2008.09.037
- Rouillard AD, Berglund CM, Lee JY, et al. Methods for photocrosslinking alginate hydrogel scaffolds with high cell viability. Tissue Eng Part C Methods. 2011;17(2):173-179. doi: 10.1089/ten.TEC.2009.0582
- Roberts JJ, Bryant SJ. Comparison of photopolymerizable thiol-ene PEG and acrylate-based PEG hydrogels for cartilage development. Biomaterials. 2013;34(38):9969-9979. doi: 10.1016/j.biomaterials.2013.09.020
- Rydholm AE, Bowman CN, Anseth KS. Degradable thiol-acrylate photopolymers: polymerization and degradation behavior of an in situ forming biomaterial. Biomaterials. 2005;26(22):4495-4506. doi: 10.1016/j.biomaterials.2004.11.046
- Gwon K, Kim E, Tae G. Heparin-hyaluronic acid hydrogel in support of cellular activities of 3D encapsulated adipose derived stem cells. Acta Biomater. 2017;49:284-295. doi: 10.1016/j.actbio.2016.12.001
- Brew L. Development of a Crosslinkable Hydrogel Derived from Placental Tissue. Master of Philosophy thesis, Queensland University of Technology; 2019.
- Shi J, Yao H, Wang B, et al. Construction of a decellularized multicomponent extracellular matrix interpenetrating network scaffold by gelatin microporous hydrogel 3d cell culture system. Macromol Rapid Commun. 2024;45(5):e2300508. doi: 10.1002/marc.202300508.
- Crosby CO, Hillsley A, Kumar S, et al. Phototunable interpenetrating polymer network hydrogels to stimulate the vasculogenesis of stem cell-derived endothelial progenitors. Acta Biomater. 2021;122:133-144. doi: 10.1016/j.actbio.2020.12.041
- Ma X, Qu X, Zhu W, et al. Deterministically patterned biomimetic human iPSC-derived hepatic model via rapid 3D bioprinting. Proc Natl Acad Sci U S A. 2016;113(8):2206-2211. doi: 10.1073/pnas.1524510113
- Kim Y, Kang K, Jeong J, et al. Three-dimensional (3D) printing of mouse primary hepatocytes to generate 3D hepatic structure. Ann Surg Treat Res. 2017;92(2):67-72. doi: 10.4174/astr.2017.92.2.67
- Hiller T, Berg J, Elomaa L, et al. Generation of a 3D liver model comprising human extracellular matrix in an alginate/ gelatin-based bioink by extrusion bioprinting for infection and transduction studies. Int J Mol Sci. 2018;19(10):3129. doi: 10.3390/ijms19103129
- Lewis PL, Green RM, Shah RN. 3D-printed gelatin scaffolds of differing pore geometry modulate hepatocyte function and gene expression. Acta Biomater. 2018;69:63-70. doi: 10.1016/j.actbio.2017.12.042
- Yang H, Sun L, Pang Y, et al. Three-dimensional bioprinted hepatorganoids prolong survival of mice with liver failure. Gut. 2021;70(3):567-574. doi: 10.1136/gutjnl-2019-319960
- Lewis PL, Yan M, Su J, Shah RN. Directing the growth and alignment of biliary epithelium within extracellular matrix hydrogels. Acta Biomater. 2019;85:84-93. doi: 10.1016/j.actbio.2018.12.039
- Goulart E, de Caires-Junior LC, Telles-Silva KA, et al. 3D bioprinting of liver spheroids derived from human induced pluripotent stem cells sustain liver function and viability in vitro. Biofabrication. 2019;12(1):015010. doi: 10.1088/1758-5090/ab4a30
- Kang HK, Sarsenova M, Kim DH, et al. Establishing a 3D in vitro hepatic model mimicking physiologically relevant to in vivo state. Cells. 2021;10(5):1268. doi: 10.3390/cells10051268
- Grix T, Ruppelt A, Thomas A, et al. Bioprinting perfusion-enabled liver equivalents for advanced organ-on-a-chip applications. Genes (Basel). 2018;9(4):176. doi: 10.3390/genes9040176
- Liu Tsang V, Chen AA, Cho LM, et al. Fabrication of 3D hepatic tissues by additive photopatterning of cellular hydrogels. FASEB J. 2007;21(3):790-801. doi: 10.1096/fj.06-7117com
- Gibert-Ramos A, Sanfeliu-Redondo D, Aristu-Zabalza P, et al. The hepatic sinusoid in chronic liver disease: the optimal milieu for cancer. Cancers (Basel). 2021;13(22):5719. doi: 10.3390/cancers13225719
- Murphy SV, Atala A. 3D bioprinting of tissues and organs. Nat Biotechnol. 2014;32(8):773-785. doi: 10.1038/nbt.2958
- Saunders RE, Derby B. Inkjet printing biomaterials for tissue engineering: bioprinting. Int Mater Rev. 2014;59(8):430-448. doi: 10.1179/1743280414Y.0000000040
- Cui X, Breitenkamp K, Finn MG, Lotz M, D’Lima DD. Direct human cartilage repair using three-dimensional bioprinting technology. Tissue Eng Part A. 2012;18(11-12):1304-1312. doi: 10.1089/ten.TEA.2011.0543
- Matsusaki M, Sakaue K, Kadowaki K, Akashi M. Three-dimensional human tissue chips fabricated by rapid and automatic inkjet cell printing. Adv Healthc Mater. 2013;2(4):534-539. doi: 10.1002/adhm.201200299
- Dababneh AB, Ozbolat IT. Bioprinting technology: a current state-of-the-art review. J Manuf Sci Eng. 2014;136(6):061016. doi: 10.1115/1.4028512
- Jungst T, Smolan W, Schacht K, Scheibel T, Groll J. Strategies and molecular design criteria for 3D printable hydrogels. Chem Rev. 2016;116(3):1496-1539. doi: 10.1021/acs.chemrev.5b00303
- Chua CK, Yeong WY. Bioprinting: Principles and Applications. World Scientific Publishing Co Inc; 2014.
- You S, Li J, Zhu W, Yu C, Mei D, Chen S. Nanoscale 3D printing of hydrogels for cellular tissue engineering. J Mater Chem B. 2018;6(15):2187-2197. doi: 10.1039/C8TB00301G
- Grigoryan B, Paulsen SJ, Corbett DC, et al. Multivascular networks and functional intravascular topologies within biocompatible hydrogels. Science. 2019;364(6439): 458-464. doi: 10.1126/science.aav9750
- Kim MK, Jeong W, Lee SM, Kim JB, Jin S, Kang HW. Decellularized extracellular matrix-based bio-ink with enhanced 3D printability and mechanical properties. Biofabrication. 2020;12(2):025003. doi: 10.1088/1758-5090/ab5d80
- Lee H, Han W, Kim H, et al. Development of liver decellularized extracellular matrix bioink for three-dimensional cell printing-based liver tissue engineering. Biomacromolecules. 2017;18(4):1229-1237. doi: 10.1021/acs.biomac.6b01908
- Skardal A, Devarasetty M, Kang HW, et al. A hydrogel bioink toolkit for mimicking native tissue biochemical and mechanical properties in bioprinted tissue constructs. Acta Biomater. 2015;25:24-34. doi: 10.1016/j.actbio.2015.07.030
- Mazzocchi A, Devarasetty M, Huntwork R, Soker S, Skardal A. Optimization of collagen type I-hyaluronan hybrid bioink for 3D bioprinted liver microenvironments. Biofabrication. 2018;11(1):015003. doi: 10.1088/1758-5090/aae543
- Jeon H, Kang K, Park SA, et al. Generation of multilayered 3D structures of HepG2 cells using a bio-printing technique. Gut Liver. 2017;11(1):121-128. doi: 10.5009/gnl16010
- Lee JW, Choi YJ, Yong WJ, et al. Development of a 3D cell printed construct considering angiogenesis for liver tissue engineering. Biofabrication. 2016;8(1):015007. doi: 10.1088/1758-5090/8/1/015007
- Bernal PN, Delrot P, Loterie D, et al. Volumetric bioprinting of complex living-tissue constructs within seconds. Adv Mater. 2019;31(42):e1904209. doi: 10.1002/adma.201904209
- Leva V, Chatzipetrou M, Alexopoulos L, Tzeranis DS, Zergioti I. Direct laser printing of liver cells on porous collagen scaffolds. J Laser Micro Nanoeng. 2018;13(3):234-237. doi: 10.2961/jlmn.2018.03.0015
- Yu C, Ma X, Zhu W, et al. Scanningless and continuous 3D bioprinting of human tissues with decellularized extracellular matrix. Biomaterials. 2019;194:1-13. doi: 10.1016/j.biomaterials.2018.12.009
- Antoshin AA, Churbanov SN, Minaev NV, et al. LIFT-bioprinting, is it worth it? Bioprinting. 2019;15,2019, doi: 10.1016/j.bprint.2019.e00052.
- Antoshin A, Minaeva E, Koteneva P, et al. LIFT of cell spheroids: proof of concept. Bioprinting. 2023;34: e00297. doi: 10.1016/j.bprint.2023.e00297.
- Catros S, Fricain JC, Guillotin B, et al. Laser-assisted bioprinting for creating on-demand patterns of human osteoprogenitor cells and nano-hydroxyapatite. Biofabrication. 2011;3(2):025001. doi: 10.1088/1758-5082/3/2/025001
- Koch L, Deiwick A, Schlie S, et al. Skin tissue generation by laser cell printing. Biotechnol Bioeng. 2012;109(7):1855-1863. doi: 10.1002/bit.24455
- Miri AK, Mirzaee I, Hassan S, et al. Effective bioprinting resolution in tissue model fabrication. Lab Chip. 2019;19(11):2019-2037. doi: 10.1039/c8lc01037d
- Ma X, Yu C, Wang P, et al. Rapid 3D bioprinting of decellularized extracellular matrix with regionally varied mechanical properties and biomimetic microarchitecture. Biomaterials. 2018;185:310-321. doi: 10.1016/j.biomaterials.2018.09.026
- Zhu W, Qu X, Zhu J, et al. Direct 3D bioprinting of prevascularized tissue constructs with complex microarchitecture. Biomaterials. 2017;124:106-115. doi: 10.1016/j.biomaterials.2017.01.042
- Zhang X, Zhang X, Li Y, Zhang Y. Applications of light-bBased 3D bioprinting and photoactive biomaterials for tissue engineering. Materials (Basel). 2023;16(23):7461. doi: 10.3390/ma16237461
- Ovsianikov A, Mühleder S, Torgersen J, et al. Laser photofabrication of cell-containing hydrogel constructs. Langmuir. 2014;30(13):3787-3794. doi: 10.1021/la402346z
- Traverse JH, Henry TD, Dib N, et al. First-in-man study of a cardiac extracellular matrix hydrogel in early and late myocardial infarction patients. JACC Basic Transl Sci. 2019;4(6):659-669. doi: 10.1016/j.jacbts.2019.07.012
- Cai D, Weng W. Development potential of extracellular matrix hydrogels as hemostatic materials. Front Bioeng Biotechnol. 2023;11:1187474. doi: 10.3389/fbioe.2023.1187474
- Fong AH, Romero-López M, Heylman CM, et al. Three-dimensional adult cardiac extracellular matrix promotes maturation of human induced pluripotent stem cell-derived cardiomyocytes. Tissue Eng Part A. 2016;22(15-16):1016-1025. doi: 10.1089/ten
- Gaetani R, Yin C, Srikumar N, et al. Cardiac-derived extracellular matrix enhances cardiogenic properties of human cardiac progenitor cells. Cell Transplant. 2016;25(9):1653-1663. doi: 10.3727/096368915X689794
- Wang RM, Johnson TD, He J, et al. Humanized mouse model for assessing the human immune response to xenogeneic and allogeneic decellularized biomaterials. Biomaterials. 2017;129:98-110. doi: 10.1016/j.biomaterials.2017.03.016
- Pok S, Stupin IV, Tsao C, et al. Full-thickness heart repair with an engineered multilayered myocardial patch in rat model. Adv Healthc Mater. 2017;6(5):10.1002/adhm.201600549. doi: 10.1002/adhm.201600549
- Efraim Y, Sarig H, Cohen Anavy N, et al. Biohybrid cardiac ECM-based hydrogels improve long term cardiac function post myocardial infarction. Acta Biomater. 2017;50:220-233. doi: 10.1016/j.actbio.2016.12.015
- Jang J, Park HJ, Kim SW, et al. 3D printed complex tissue construct using stem cell-laden decellularized extracellular matrix bioinks for cardiac repair. Biomaterials. 2017;112:264-274. doi: 10.1016/j.biomaterials.2016.10.026
- Francis MP, Breathwaite E, Bulysheva AA, et al. Human placenta hydrogel reduces scarring in a rat model of cardiac ischemia and enhances cardiomyocyte and stem cell cultures. Acta Biomater. 2017;52:92-104. doi: 10.1016/j.actbio.2016.12.027
- Barajaa MA, Otsuka T, Ghosh D, Kan HM, Laurencin CT. Development of porcine skeletal muscle extracellular matrix-derived hydrogels with improved properties and low immunogenicity. Proc Natl Acad Sci USA. 2024;121(19):e2322822121. doi: 10.1073/pnas.2322822121
- Ungerleider JL, Johnson TD, Hernandez MJ, et al. Extracellular matrix hydrogel promotes tissue remodeling, arteriogenesis, and perfusion in a rat hindlimb ischemia Model. JACC Basic Transl Sci. 2016;1(1-2):32-44. doi: 10.1016/j.jacbts.2016.01.009
- Wolf MT, Daly KA, Brennan-Pierce EP, et al. A hydrogel derived from decellularized dermal extracellular matrix. Biomaterials. 2012;33(29):7028-7038. doi: 10.1016/j.biomaterials.2012.06.051
- Long C, Galvez MG, Legrand A, et al. Intratendinous injection of hydrogel for reseeding decellularized human flexor tendons. Plast Reconstr Surg. 2017;139(6):1305e-1314e. doi: 10.1097/PRS.0000000000003359
- Burnsed OA, Schwartz Z, Marchand KO, Hyzy SL, Olivares- Navarrete R, Boyan BD. Hydrogels derived from cartilage matrices promote induction of human mesenchymal stem cell chondrogenic differentiation. Acta Biomater. 2016;43:139-149. doi: 10.1016/j.actbio.2016.07.034
- Shimomura K, Rothrauff BB, Tuan RS. Region-specific effect of the decellularized meniscus extracellular matrix on mesenchymal stem cell-based meniscus tissue engineering. Am J Sports Med. 2017;45(3):604-611. doi: 10.1177/0363546516674184
- Ravichandran A, Murekatete B, Moedder D., et al. Photocrosslinkable liver extracellular matrix hydrogels for the generation of 3D liver microenvironment models. Sci Rep. 2021;11:15566. doi: 10.1038/s41598-021-94990-z
- Willemse J, van Tienderen G, van Hengel E, et al. Hydrogels derived from decellularized liver tissue support the growth and differentiation of cholangiocyte organoids. Biomaterials. 2022;284:121473. doi: 10.1016/j.biomaterials.2022.121473
- Faust A, Kandakatla A, van der Merwe Y, et al. Urinary bladder extracellular matrix hydrogels and matrix-bound vesicles differentially regulate central nervous system neuron viability and axon growth and branching. J Biomater Appl. 2017;31(9):1277-1295. doi: 10.1177/088532821769806
- Kočí Z, Výborný K, Dubišová J, et al. Extracellular matrix hydrogel derived from human umbilical cord as a scaffold for neural tissue repair and its comparison with extracellular matrix from porcine tissues. Tissue Eng Part C Methods. 2017;23(6):333-345. doi: 10.1089/ten.TEC.2017.0089
- van Dongen JA, Getova V, Brouwer LA, et al. Adipose tissue-derived extracellular matrix hydrogels as a release platform for secreted paracrine factors. J Tissue Eng Regen Med. 2019;13(6):973-985. doi: 10.1002/term.2843
- Mahoney CM, Kelmindi-Doko A, Snowden MJ, Peter Rubin J, Marra KG. Adipose derived delivery vehicle for encapsulated adipogenic factors. Acta Biomater. 2017;58:26-33. doi: 10.1016/j.actbio.2017.05.046
- Kayabolen A, Keskin D, Aykan A, Karslıoglu Y, Zor F, Tezcaner A. Native extracellular matrix/fibroin hydrogels for adipose tissue engineering with enhanced vascularization. Biomed Mater. 2017;12(3):035007. doi: 10.1088/1748-605X/aa6a63
- Neishabouri A, Soltani Khaboushan A, Daghigh F, Kajbafzadeh AM, Majidi Zolbin M. Decellularization in tissue engineering and regenerative medicine: evaluation, modification, and application methods. Front Bioeng Biotechnol. 2022;10:805299. doi: 10.3389/fbioe.2022.805299
- Hernandez MJ. Translation of an Injectable Decellularized Extracellular Matrix Hydrogel for Promoting Skeletal Muscle Regeneration. University of California San Diego; 2019.
- Zhang W, Du A, Liu S, Lv M, Chen S. Research progress in decellularized extracellular matrix-derived hydrogels. Regen Ther. 2021;18:88-96. doi: 10.1016/j.reth.2021.04.002
- Zhu W, Cao L, Song C, Pang Z, Jiang H, Guo C. Cell-derived decellularized extracellular matrix scaffolds for articular cartilage repair. Int J Artif Organs. 2021;44(4):269-281. doi: 10.1177/0391398820953866
- Peshkova M, Korneev A, Revokatova D, et al. Four sides to the story: A proteomic comparison of liquid-phase and matrix-bound extracellular vesicles in 2D and 3D cell cultures. Proteomics. 2024;24(18):e2300375. doi: 10.1002/pmic.202300375
- Assunção M, Dehghan-Baniani D, Yiu CHK, Später T, Beyer S, Blocki A. Cell-derived extracellular matrix for tissue engineering and regenerative medicine. Front Bioeng Biotechnol. 2020;8:602009. doi: 10.3389/fbioe.2020.602009
- Sharma D, Ferguson M, Zhao F. A step-by-step protocol for generating human fibroblast cell-derived completely biological extracellular matrix scaffolds. Methods Cell Biol. 2020;156:3-13. doi: 10.1016/bs.mcb.2019.10.010
- Lee JS, Nah H, Lee D, et al. Immediately implantable extracellular matrix-enriched osteoinductive hydrogel-laden 3D-printed scaffold for promoting vascularized bone regeneration in vivo. Mater Des Vol. 2022; 219:110801. doi: 10.1016/j.matdes.2022.110801
- Shin YJ, Shafranek RT, Tsui JH, Walcott J, Nelson A, Kim DH. 3D bioprinting of mechanically tuned bioinks derived from cardiac decellularized extracellular matrix. Acta Biomater. 2021;119:75-88. doi: 10.1016/j.actbio.2020.11.006
- Casarin M, Fortunato TM, Imran S, et al. Porcine small intestinal submucosa (SIS) as a Suitable Scaffold for the creation of a tissue-engineered urinary conduit: decellularization, biomechanical and biocompatibility characterization using new approaches. Int J Mol Sci. 2022;23(5):2826. doi: 10.3390/ijms23052826
- Toprakhisar B, Nadernezhad A, Bakirci E, Khani N, Skvortsov GA, Koc B. Development of bioink from decellularized tendon extracellular matrix for 3D bioprinting. Macromol Biosci. 2018;18(10):e1800024. doi: 10.1002/mabi.201800024
- Vishwakarma SK, Bhavani PG, Bardia A, et al. Preparation of natural three-dimensional goat kidney scaffold for the development of bioartificial organ. Indian J Nephrol. 2014;24(6):372-375. doi: 10.4103/0971-4065.133008
- Geerts S, Ozer S, Jaramillo M, Yarmush ML, Uygun BE. Nondestructive methods for monitoring cell removal during rat liver decellularization. Tissue Eng Part C Methods. 2016;22(7):671-678. doi: 10.1089/ten.TEC.2015.0571
- Ren X, Moser PT, Gilpin SE, et al. Engineering pulmonary vasculature in decellularized rat and human lungs. Nat Biotechnol. 2015;33(10):1097-1102. doi: 10.1038/nbt.3354
- Di Francesco D, Marcello E, Casarella S, et al. Characterization of a decellularized pericardium extracellular matrix hydrogel for regenerative medicine: insights on animal-to-animal variability. Front Bioeng Biotechnol. 2024;12:1452965. doi: 10.3389/fbioe.2024.1452965
- Tottey S, Johnson SA, Crapo PM, et al. The effect of source animal age upon extracellular matrix scaffold properties. Biomaterials. 2011;32(1):128-136. doi: 10.1016/j.biomaterials.2010.09.006
- Porzionato A, Stocco E, Barbon S, Grandi F, Macchi V, De Caro R. Tissue-engineered grafts from human decellularized extracellular matrices: a systematic review and future perspectives. Int J Mol Sci. 2018;19(12):4117. doi: 10.3390/ijms19124117
- Meran L, Massie I, Campinoti S, et al. Engineering transplantable jejunal mucosal grafts using patient-derived organoids from children with intestinal failure. Nat Med. 2020;26(10):1593-1601. doi: 10.1038/s41591-020-1024-z
- Garreta E, de Oñate L, Fernández-Santos ME, et al. Myocardial commitment from human pluripotent stem cells: rapid production of human heart grafts. Biomaterials. 2016;98:64-78. doi: 10.1016/j.biomaterials.2016.04.003
- Chen Y, Chen J, Zhang Z, et al. Current advances in the development of natural meniscus scaffolds: innovative approaches to decellularization and recellularization. Cell Tissue Res. 2017;370(1):41-52. doi: 10.1007/s00441-017-2605-0
- Hassanpour A, Talaei-Khozani T, Kargar-Abarghouei E, Razban V, Vojdani Z. Decellularized human ovarian scaffold based on a sodium lauryl ester sulfate (SLES)- treated protocol, as a natural three-dimensional scaffold for construction of bioengineered ovaries. Stem Cell Res Ther. 2018;9(1):252. doi: 10.1186/s13287-018-0971-5
- Brown CF, Yan J, Han TT, Marecak DM, Amsden BG, Flynn LE. Effect of decellularized adipose tissue particle size and cell density on adipose-derived stem cell proliferation and adipogenic differentiation in composite methacrylated chondroitin sulphate hydrogels. Biomed Mater. 2015;10(4):045010. doi: 10.1088/1748-6041/10/4/045010
- Tremmel DM, Sackett SD, Feeney AK, et al. A human pancreatic ECM hydrogel optimized for 3-D modeling of the islet microenvironment. Sci Rep. 2022;12(1):7188. doi: 10.1038/s41598-022-11085-z
- Peloso A, Petrosyan A, Da Sacco S, et al. Renal extracellular matrix scaffolds from discarded kidneys maintain glomerular morphometry and vascular resilience and retains critical growth factors. Transplantation. 2015;99(9):1807-1816. doi: 10.1097/TP.0000000000000811.
- Milan PB, Lotfibakhshaiesh N, Joghataie MT, et al. Accelerated wound healing in a diabetic rat model using decellularized dermal matrix and human umbilical cord perivascular cells. Acta Biomater. 2016;45:234-246. doi: 10.1016/j.actbio.2016.08.053
- Matoug-Elwerfelli M, Duggal MS, Nazzal H, Esteves F, Raïf E. A biocompatible decellularized pulp scaffold for regenerative endodontics. Int Endod J. 2018;51(6):663-673. doi: 10.1111/iej.12882
- Nichols JE, La Francesca S, Vega SP, et al. Giving new life to old lungs: methods to produce and assess whole human paediatric bioengineered lungs. J Tissue Eng Regen Med. 2017;11(7):2136-2152. doi: 10.1002/term.2113
- Yamanaka H, Morimoto N, Yamaoka T. Decellularization of submillimeter-diameter vascular scaffolds using peracetic acid. J Artif Organs. 2020;23(2):156-162. doi: 10.1007/s10047-019-01152-0
- Jiwangga D, Mahyudin F, Mastutik G, Juliana, Meitavany EN. Current Strategies for Tracheal Decellularization: A Systematic Review. Int J Biomater. 2024;2024:3355239. doi: 10.1155/2024/3355239
- Sackett SD, Tremmel DM, Ma F, et al. Extracellular matrix scaffold and hydrogel derived from decellularized and delipidized human pancreas. Sci Rep. 2018;8:10452. doi: 10.1038/s41598-018-28857-1
- Pankajakshan D, Voytik-Harbin SL, Nör JE, Bottino MC. Injectable highly tunable oligomeric collagen matrices for dental tissue regeneration. ACS Appl Bio Mater. 2020;3(2):859-868. doi: 10.1021/acsabm.9b00944
- Kojima H, Kushige H, Yagi H, et al. Combinational treatment involving decellularized extracellular matrix hydrogels with mesenchymal stem cells increased the efficacy of cell therapy in pancreatitis. Cell Transplant. 2023;32:9636897231170437. doi: 10.1177/09636897231170437
- Davidenko N, Schuster CF, Bax DV, et al. Control of crosslinking for tailoring collagen-based scaffolds stability and mechanics. Acta Biomater. 2015;25:131-142. doi: 10.1016/j.actbio.2015.07.034
- Shie MY, Lee JJ, Ho CC, Yen SY, Ng HY, Chen YW. Effects of gelatin methacrylate bio-ink concentration on mechano-physical properties and human dermal fibroblast behavior. Polymers (Basel). 2020;12(9):1930. doi: 10.3390/polym12091930
- Singh RK, Seliktar D, Putnam AJ. Capillary morphogenesis in PEG-collagen hydrogels. Biomaterials. 2013;34(37):9331-9340. doi: 10.1016/j.biomaterials.2013.08.016
- Antebi B, Zhang Z, Wang Y, Lu Z, Chen XD, Ling J. Stromal-cell-derived extracellular matrix promotes the proliferation and retains the osteogenic differentiation capacity of mesenchymal stem cells on three-dimensional scaffolds. Tissue Eng Part C Methods. 2015;21(2):171-181. doi: 10.1089/ten.TEC.2014.0092
- Madrid-Wolff J, Boniface A, Loterie D, Delrot P, Moser C. Controlling light in scattering materials for volumetric additive manufacturing. Adv Sci (Weinh). 2022;9(22):e2105144. doi: 10.1002/advs.202105144