Bioprinting with superelastic and fatigue-resistant bioinks for large-sized tissue delivery
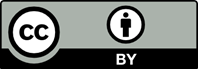
3D bioprinting technology is advancing rapidly to construct multi-scale preformed architectures that satisfy the demands of tissue regeneration. However, challenges remain in accurately delivering large-sized preformed architectures to the defect sites without being damaged by the mechanical environment in vivo. In this study, we proposed a versatile bioprinting strategy to fabricate large-sized architectures with remarkable injection capacity for geometry-independent minimally invasive tissue delivery. We design a novel hydrogel by mixing gelatin methacryloyl with poly(ethylene glycol) diacrylate (PEGDA) and photocrosslinking the mixture under a white light source. The hydrogel forms a reinforced crosslinking network, exhibiting superelasticity and fatigue resistance. We infer that the flexible chains of high-molecular-weight PEGDA and interconnected macropores of hydrogel contribute to the reinforcement mechanisms. With a decoupled bioprinting strategy, the bioink can be 3D printed into large-sized architectures with different geometries and tunable Poisson’s ratio. The printing architectures displayed excellent deformation and shape recovery capacity by compressing to less than 1% of the original size and fully recovering after injection. The injection capacity is geometry-independent, indicating the intrinsic properties of the hydrogel and allowing higher freedom for the structural design and geometry size of the architectures. The bioprinting process is compatible with genome-edited hepatic cells with high cell-bioprinting suitability, demonstrating high cell viability, a minimally altered transcriptomic profile after bioprinting, and a biocompatible microenvironment that supports cell survival and hepatic function maintenance. The cell-laden architectures express uncompromised injection capacity with unaffected cell viability after injection. This study presents a generalizable strategy for preparing and bioprinting with superelastic and fatigue-resistant bioinks into customized cell-laden architectures to facilitate minimally invasive large-sized tissue delivery.
- Zhang J, Eyisoylu H, Qin XH, Rubert M, Muller R. 3D bioprinting of graphene oxide-incorporated cell-laden bone mimicking scaffolds for promoting scaffold fidelity, osteogenic differentiation and mineralization. Acta Biomater. 2021;121:637-652. doi: 10.1016/j.actbio.2020.12.026
- Murphy SV, De Coppi P, Atala A. Opportunities and challenges of translational 3D bioprinting. Nat Biomed Eng. 2020;4(4):370-380. doi: 10.1038/s41551-019-0471-7
- Chen A, Wang W, Mao Z, et al. Multimaterial 3D and 4D bioprinting of heterogenous constructs for tissue engineering. Adv Mater. 2023:e2307686. doi: 10.1002/adma.202307686
- Eggermont LJ, Rogers ZJ, Colombani T, Memic A, Bencherif SA. Injectable cryogels for biomedical applications. Trends Biotechnol. 2020;38(4):418-431. doi: 10.1016/j.tibtech.2019.09.008
- Zarrintaj P, Khodadadi YM, Youssefi AM, et al. Injectable cell-laden hydrogels for tissue engineering: recent advances and future opportunities. Tissue Eng Part A. 2021; 27(11-12):821-843. doi: 10.1089/ten.TEA.2020.0341
- Chen R, Li L, Feng L, et al. Biomaterial-assisted scalable cell production for cell therapy. Biomaterials. 2020;230:119627. doi: 10.1016/j.biomaterials.2019.119627
- Huang X, Ma Y, Li Y, Han F, Lin W. Targeted drug delivery systems for kidney diseases. Front Bioeng Biotechnol. 2021;9:683247. doi: 10.3389/fbioe.2021.683247
- Ercan H, Durkut S, Koc-Demir A, Elcin AE, Elcin YM. Clinical applications of injectable biomaterials. Adv Exp Med Biol. 2018;1077:163-182. doi: 10.1007/978-981-13-0947-2_10
- Yao R, Zhang R, Lin F, Luan J. Biomimetic injectable HUVEC-adipocytes/collagen/alginate microsphere co-cultures for adipose tissue engineering. Biotechnol Bioeng. 2013;110(5):1430-1443. doi: 10.1002/bit.24784
- Yao R, Alkhawtani A, Chen R, Luan J, Xu M. Rapid and efficient in vivo angiogenesis directed by electro-assisted bioprinting of alginate/collagen microspheres with human umbilical vein endothelial cell coating layer. Int J Bioprint. 2019;5(2.1):194. doi: 10.18063/ijb.v5i2.1.194
- Chen TC, Wong CW, Hsu SH. Three-dimensional printing of chitosan cryogel as injectable and shape recoverable scaffolds. Carbohydr Polym. 2022;285:119228. doi: 10.1016/j.carbpol.2022.119228
- Beduer A, Piacentini N, Aeberli L, et al. Additive manufacturing of hierarchical injectable scaffolds for tissue engineering. Acta Biomater. 2018;76:71-79. doi: 10.1016/j.actbio.2018.05.056
- Ying G, Jiang N, Parra C, et al. Bioprinted injectable hierarchically porous gelatin methacryloyl hydrogel constructs with shape-memory properties. Adv Funct Mater. 2020;30(46):2003740. doi: 10.1002/adfm.202003740
- Porto ST, Cejas CM, Cunha RL. Microfluidics as a tool to assess and induce emulsion destabilization. Soft Matter. 2022;18(4):698-710. doi: 10.1039/d1sm01588e
- Tilton M, Camilleri ET, Astudillo PM, et al. Visible light-induced 3D bioprinted injectable scaffold for minimally invasive tissue regeneration. Biomater Adv. 2023;153:213539. doi: 10.1016/j.bioadv.2023.213539
- Jian X, Wang H, Jian X, et al. A flexible adhesive hydrogel dressing of embedded structure with pro-angiogenesis activity for wound repair at moving parts inspired by commercial adhesive bandages. Mater Today Adv. 2024;21:100452. doi: 10.1016/j.mtadv.2023.100452
- Lewis JB, Wataha JC, Messer RL, Caughman GB, Yamamoto T, Hsu SD. Blue light differentially alters cellular redox properties. J Biomed Mater Res B Appl Biomater. 2005;72(2):223-229. doi: 10.1002/jbm.b.30126
- Zhang MD, Huang X, Li Z, et al. White-light-induced synthesis of injectable alginate-based composite hydrogels for rapid hemostasis. Mil Med Res. 2023;10(1):47. doi: 10.1186/s40779-023-00483-7
- Feng L, Liang S, Zhou Y, et al. Three-dimensional printing of hydrogel scaffolds with hierarchical structure for scalable stem cell culture. Acs Biomater Sci Eng. 2020;6(5):2995-3004. doi: 10.1021/acsbiomaterials.9b01825
- Kumar A, Lee Y, Kim D, et al. Effect of crosslinking functionality on microstructure, mechanical properties, and in vitro cytocompatibility of cellulose nanocrystals reinforced poly (vinyl alcohol)/sodium alginate hybrid scaffolds. Int J Biol Macromol. 2017;95:962-973. doi: 10.1016/j.ijbiomac.2016.10.085
- Joyce K, Fabra GT, Bozkurt Y, Pandit A. Bioactive potential of natural biomaterials: identification, retention and assessment of biological properties. Signal Transduct Target Ther. 2021;6(1):122. doi: 10.1038/s41392-021-00512-8
- Ghavaminejad A, Ashammakhi N, Wu XY, Khademhosseini A. Crosslinking strategies for 3D bioprinting of polymeric hydrogels. Small. 2020;16(35):e2002931. doi: 10.1002/smll.202002931
- Yue K, Trujillo-De SG, Alvarez MM, Tamayol A, Annabi N, Khademhosseini A. Synthesis, properties, and biomedical applications of gelatin methacryloyl (GelMA) hydrogels. Biomaterials. 2015;73:254-271. doi: 10.1016/j.biomaterials.2015.08.045
- Sharifi S, Sharifi H, Akbari A, Chodosh J. Systematic optimization of visible light-induced crosslinking conditions of gelatin methacryloyl (GelMA). Sci Rep. 2021; 11(1):23276. doi: 10.1038/s41598-021-02830-x
- Bahney CS, Lujan TJ, Hsu CW, Bottlang M, West JL, Johnstone B. Visible light photoinitiation of mesenchymal stem cell-laden bioresponsive hydrogels. Eur Cell Mater. 2011;22:43-55. doi: 10.22203/ecm.v022a04
- Bryant SJ, Nuttelman CR, Anseth KS. Cytocompatibility of UV and visible light photoinitiating systems on cultured NIH/3T3 fibroblasts in vitro. J Biomater Sci Polym Ed. 2000;11(5):439-457. doi: 10.1163/156856200743805
- Slaughter BV, Khurshid SS, Fisher OZ, Khademhosseini A, Peppas NA. Hydrogels in regenerative medicine. Adv Mater. 2009;21(32-33):3307-3329. doi: 10.1002/adma.200802106
- Wang Z, Kumar H, Tian Z, et al. Visible light photoinitiation of cell-adhesive gelatin methacryloyl hydrogels for stereolithography 3D bioprinting. ACS Appl Mater Interfaces. 2018;10(32):26859-26869. doi: 10.1021/acsami.8b06607
- Wang Y, Ma M, Wang J, et al. Development of a photo-crosslinking, biodegradable GelMA/PEGDA hydrogel for guided bone regeneration materials. Materials (Basel). 2018;11(8):1345. doi: 10.3390/ma11081345
- Nguyen AK, Goering PL, Reipa V, Narayan RJ. Toxicity and photosensitizing assessment of gelatin methacryloyl-based hydrogels photoinitiated with lithium phenyl- 2,4,6-trimethylbenzoylphosphinate in human primary renal proximal tubule epithelial cells. Biointerphases. 2019;14(2):021007. doi: 10.1116/1.5095886
- Grigoryan B, Paulsen SJ, Corbett DC, et al. Multivascular networks and functional intravascular topologies within biocompatible hydrogels. Science. 2019;364(6439):458-464. doi: 10.1126/science.aav9750
- He B, Wang J, Xie M, et al. 3D printed biomimetic epithelium/ stroma bilayer hydrogel implant for corneal regeneration. Bioact Mater. 2022;17:234-247. doi: 10.1016/j.bioactmat.2022.01.034
- Li W, Hu X, Liu H, et al. 3D light-curing printing to construct versatile octopus-bionic patches. J Mater Chem B. 2023;11(22):5010-5020. doi: 10.1039/d3tb00590a
- Qiu L, Liu JZ, Chang SLY, Wu Y, Li D. Biomimetic superelastic graphene-based cellular monoliths. Nat Commun. 2012;3:1241. doi: 10.1038/ncomms2251
- Zhao Y, Li Y, Mao S, Sun W, Yao R. The influence of printing parameters on cell survival rate and printability in microextrusion-based 3D cell printing technology. Biofabrication. 2015;7(4):45002. doi: 10.1088/1758-5090/7/4/045002
- Ouyang L, Yao R, Zhao Y, Sun W. Effect of bioink properties on printability and cell viability for 3D bioplotting of embryonic stem cells. Biofabrication. 2016;8(3):35020. doi: 10.1088/1758-5090/8/3/035020
- Gripon P, Rumin S, Urban S, et al. Infection of a human hepatoma cell line by hepatitis B virus. Proc Natl Acad Sci USA. 2002;99(24):15655–15660. doi: 10.1073/pnas.232137699
- Roulot D, Czernichow S, Le Clesiau H, Costes JL, Vergnaud AC, Beaugrand M. Liver stiffness values in apparently healthy subjects: influence of gender and metabolic syndrome. J Hepatol. 2008;48(4):606-613. doi: 10.1016/j.jhep.2007.11.020
- Tringides CM, Vachicouras N, de Lazaro I, et al. Viscoelastic surface electrode arrays to interface with viscoelastic tissues. Nat Nanotechnol. 2021;16(9):1019-1029. doi: 10.1038/s41565-021-00926-z
- Han D, Dara L, Win S, et al. Regulation of drug-induced liver injury by signal transduction pathways: critical role of mitochondria. Trends Pharmacol Sci. 2013;34(4):243-253. doi: 10.1016/j.tips.2013.01.009
- Haapaniemi E, Botla S, Persson J, Schmierer B, Taipale J. CRISPR-Cas9 genome editing induces a p53-mediated DNA damage response. Nat Med. 2018;24(7):927-930. doi: 10.1038/s41591-018-0049-z
- Zhu W, Cui H, Boualam B, et al. 3D bioprinting mesenchymal stem cell-laden construct with core-shell nanospheres for cartilage tissue engineering. Nanotechnology. 2018;29(18):185101. doi: 10.1088/1361-6528/aaafa1
- Duan J, Cao Y, Shen Z, et al. 3D bioprinted GelMA/ PEGDA hybrid scaffold for establishing an in vitro model of melanoma. J Microbiol Biotechn. 2022;32(4):531-540. doi: 10.4014/jmb.2111.11003
- Wang Z, Abdulla R, Parker B, Samanipour R, Ghosh S, Kim K. A simple and high-resolution stereolithography-based 3D bioprinting system using visible light crosslinkable bioinks. Biofabrication. 2015;7(4):45009. doi: 10.1088/1758-5090/7/4/045009