Emerging frontiers in 3D bioprinting: Harnessing decellularized matrix bioink for advancements in musculoskeletal tissue engineering
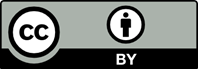
The musculoskeletal system plays a pivotal role in maintaining posture, safeguarding organs, facilitating flexible movement, and supporting cellular metabolic functions, thereby enabling active participation in various aspects of life. Conventional treatment modalities, such as autologous and allogeneic transplantation, face significant challenges, including donor scarcity, complications, rejection, and infection. The emergence of three-dimensional (3D) bioprinting technology, coupled with bioink sourced from the decellularized extracellular matrix (dECM), offers a promising approach for repairing and regenerating musculoskeletal tissue. This article meticulously examines common methods of musculoskeletal tissue bioprinting, the properties and functions of dECM, techniques for preparing dECM bioinks, recent advancements, and applications of dECM bioinks in 3D printing of musculoskeletal tissues (e.g., heart, skeletal muscle, tendon, interfaces between tissues, bone, and cartilage). The review concludes by delineating current research limitations, aiming to catalyze future investigations in this domain.
- Potyondy T, Uquillas JA, Tebon P, Byambaa B. Recent advances in 3D bioprinting of musculoskeletal tissues. Biofabrication. 2021; 13(2):2-3. doi: 10.1088/1758-5090/abc8de
- Briggs AM, Woolf AD, Dreinhöfer K, et al. Reducing the global burden of musculoskeletal conditions. Bull World Health Organ. 2018;96(5):366-368. doi: 10.2471/BLT.17.204891
- Shibuya N, Jupiter DC. Bone graft substitute: allograft and xenograft. Clin Podiatr Med Surg. 2015;32:21-34. doi: 10.1016/j.cpm.2014.09.011
- Wang MO, Vorwald CE, Dreher ML, et al. Evaluating 3D-printed biomaterials as scaffolds for vascularized bone tissue engineering. Adv Mater. 2015;27(1):138-144. doi: 10.1002/adma.201403943
- Hoffman T, Khademhosseini A, Langer R. Chasing the paradigm: clinical translation of 25 years of tissue engineering. Tissue Eng Part A. 2019;25:679-687. doi: 10.1089/ten.TEA.2019.0032
- Golebiowska AA, Intravaia JT, Sathe VM, Kumbar SG, Nukavarapu SP. Decellularized extracellular matrix biomaterials for regenerative therapies: Advances, challenges and clinical prospects. Bioact Mater. 2023;32:98-123. doi: 10.1016/j.bioactmat.2023.09.017
- Zorlutuna P, Vrana NE, Khademhosseini A. The expanding world of tissue engineering: the building blocks and new applications of tissue engineered constructs. IEEE Rev Biomed Eng. 2013;6:47-62. doi: 10.1109/RBME.2012.2233468
- Zhe M, Wu X, Yu P, et al. Recent advances in decellularized extracellular matrix-based bioinks for 3D bioprinting in tissue engineering. Materials (Basel). 2023;16(8):3197. doi: 10.3390/ma16083197
- Tao O, Kort-Mascort J, Lin Y, et al. The applications of 3D printing for craniofacial tissue engineering. Micromachines (Basel). 2019;10(7):480. doi: 10.3390/mi10070480
- Mao AS, Mooney DJ. Regenerative medicine: current therapies and future directions. Proc Natl Acad Sci U S A. 2015;112(47):14452-14459. doi: 10.1073/pnas.1508520112
- Matai I, Kaur G, Seyedsalehi A, McClinton A, Laurencin CT. Progress in 3D bioprinting technology for tissue/ organ regenerative engineering. Biomaterials. 2020;226: 119536. doi: 10.1016/j.biomaterials.2019.119536
- Luo Z, Tang G, Ravanbakhsh H, et al. Vertical extrusion cryo(bio)printing for anisotropic tissue manufacturing. Adv Mater. 2022;34(12):e2108931. doi: 10.1002/adma.202108931
- Hospodiuk M, Dey M, Sosnoski D, Ozbolat IT. The bioink: a comprehensive review on bioprintable materials. Biotechnol Adv. 2017;35(2):217-239. doi: 10.1016/j.biotechadv.2016.12.006
- Zhang J, Wehrle E, Adamek P, et al. Optimization of mechanical stiffness and cell density of 3D bioprinted cell-laden scaffolds improves extracellular matrix mineralization and cellular organization for bone tissue engineering. Acta Biomater. 2020;114:307-322. doi: 10.1016/j.actbio.2020.07.016
- Dzobo K, Thomford NE, Senthebane DA, et al. Advances in regenerative medicine and tissue engineering: innovation and transformation of medicine. Stem Cells Int. 2018;2018:2495848. doi: 10.1155/2018/2495848
- Radhakrishnan J, Subramanian A, Krishnan UM, Sethuraman S. Injectable and 3D bioprinted polysaccharide hydrogels: from cartilage to osteochondral tissue engineering. Biomacromolecules. 2017;18(1):1-26. doi: 10.1021/acs.biomac.6b01619
- Ahlfeld T, Mateo NC, Cometta S, Guduric VTN. A novel plasma-based bioink stimulates cell proliferation and differentiation in bioprinted, mineralized constructs. ACS Appl Mater Interfaces. 2020;12:12557-12572. doi: 10.1021/acsami.0c00710
- Cheng L, Yao B, Hu T, et al. Properties of an alginate-gelatin-based bioink and its potential impact on cell migration, proliferation, and differentiation. Int J Biol Macromol. 2019;135:1107-1113. doi: 10.1016/j.ijbiomac.2019.06.017
- Ding S, Feng L, Wu J, et al. Bioprinting of stem cells: interplay of bioprinting process, bioinks, and stem cell properties. ACS Biomater Sci Eng. 2018;4(9):3108-3124. doi: 10.1021/acsbiomaterials.8b00399
- Murphy SV, Atala A. 3D bioprinting of tissues and organs. Nat Biotechnol. 2014;32:773-785. doi: 10.1038/nbt.2958
- Zheng Z, Wu J, Liu M, et al. 3D bioprinting of self-standing silk-based bioink. Adv Healthc Mater. 2018;7(6): e1701026. doi: 10.1002/adhm.201701026
- Rutz AL, Hyland KE, Jakus AE, Burghardt WR, Shah RN. A multimaterial bioink method for 3D printing tunable, cell-compatible hydrogels. Adv Mater. 2015;27:1607-1614. doi: 10.1002/adma.201405076
- Gungor-Ozkerim PS, Inci I, Zhang YS, Khademhosseini A, Dokmeci MR. Bioinks for 3D bioprinting: an overview. Biomater Sci. 2018;6:915-946. doi: 10.1039/c7bm00765e
- Pati F, Jang J, Ha DH, et al. Printing three-dimensional tissue analogues with decellularized extracellular matrix bioink. Nat Commun. 2014;5:3935. doi: 10.1038/ncomms4935
- Zhang X, Liu Y, Luo C, et al. Crosslinker-free silk/ decellularized extracellular matrix porous bioink for 3D bioprinting-based cartilage tissue engineering. Mater Sci Eng C Mater Biol Appl. 2021;118:111388. doi: 10.1016/j.msec.2020.111388
- Pati F, Cho DW. Bioprinting of 3D tissue models using decellularized extracellular matrix bioink. Methods Mol Biol. 2017;1612:381-390. doi: 10.1007/978-1-4939-7021-6_27
- Shin YJ, Shafranek RT, Tsui JH, Walcott J, Nelson A, Kim DH. 3D bioprinting of mechanically tuned bioinks derived from cardiac decellularized extracellular matrix. Acta Biomater. 2021;119:75-88. doi: 10.1016/j.actbio.2020.11.006
- Oropeza BP, Adams JR, Furth ME, Chessa J, Boland T. Bioprinting of decellularized porcine cardiac tissue for large-scale aortic models. Front Bioeng Biotechnol. 2022;10: 855186. doi: 10.3389/fbioe.2022.855186
- Park W, Gao G, Cho DW. Tissue-specific decellularized extracellular matrix bioinks for musculoskeletal tissue regeneration and modeling using 3D bioprinting technology. Int J Mol Sci. 2021;22(15):7837. doi: 10.3390/ijms22157837
- Lee J, Lee H, Jin EJ, Ryu D, Kim GH. 3D bioprinting using a new photo-crosslinking method for muscle tissue restoration. NPJ Regen Med. 2023;8:18. doi: 10.1038/s41536-023-00292-5
- Zhu W, Cao L, Song C, Pang Z, Jiang H, Guo C. Cell-derived decellularized extracellular matrix scaffolds for articular cartilage repair. Int J Artif Organs. 2021;44(4):269-281. doi: 10.1177/0391398820953866
- Sang S, Mao X, Cao Y, et al. 3D bioprinting using synovium-derived MSC-Laden photo-cross-linked ECM bioink for cartilage regeneration. ACS Appl Mater Interfaces. 2023;15(7):8895-8913. doi: 10.1021/acsami.2c19058
- Hwang DG, Jo Y, Kim M, et al. A 3D bioprinted hybrid encapsulation system for delivery of human pluripotent stem cell-derived pancreatic islet-like aggregates. Biofabrication. 2021;14(1):1-3. doi: 10.1088/1758-5090/ac23ac
- Kim H, Park MN, Kim J, Jang J, Kim HK, Cho DW. Characterization of cornea-specific bioink: high transparency, improved in vivo safety. J Tissue Eng. 2019; 10:2041731418823382. doi: 10.1177/2041731418823382
- Kim BS, Kwon YW, Kong JS, et al. 3D cell printing of in vitro stabilized skin model and in vivo pre-vascularized skin patch using tissue-specific extracellular matrix bioink: a step towards advanced skin tissue engineering. Biomaterials. 2018;168:38-53. doi: 10.1016/j.biomaterials.2018.03.040
- Yeleswarapu S, Dash A, Chameettachal S, Pati F. 3D bioprinting of tissue constructs employing dual crosslinking of decellularized extracellular matrix hydrogel. Biomater Adv. 2023;152:213494. doi: 10.1016/j.bioadv.2023.213494
- Xu P, Kankala RK, Wang S, Chen A. Decellularized extracellular matrix-based composite scaffolds for tissue engineering and regenerative medicine. Regen Biomater. 2014;11:rbad107. doi: 10.1093/rb/rbad107
- Das S, Kim SW, Choi YJ, et al. Decellularized extracellular matrix bioinks and the external stimuli to enhance cardiac tissue development in vitro. Acta Biomater. 2019;95:188-200. doi: 10.1016/j.actbio.2019.04.026
- Choi YJ, Kim TG, Jeong J, et al. 3D cell printing of functional skeletal muscle constructs using skeletal muscle-derived bioink. Adv Healthc Mater. 2016;5(20):2636-2645. doi: 10.1002/adhm.201600483
- Yang SS, Choi WH, Song B, Jin H. Fabrication of an osteochondral graft with using a solid freeform fabrication system. Tissue Eng Regener Med. 2015;12:239-248. doi: 10.1007/s13770-015-0001-y
- Tsui JH, Leonard A, Camp ND, et al. Tunable electroconductive decellularized extracellular matrix hydrogels for engineering human cardiac microphysiological systems. Biomaterials. 2021;272:120764. doi: 10.1016/j.biomaterials.2021.120764
- Heinrich MA, Liu W, Jimenez A, et al. 3D bioprinting: from Benches to translational applications. Small. 2019;15(23):e1805510. doi: 10.1002/smll.201805510
- Tuan RS, Boland G, Tuli R. Adult mesenchymal stem cells and cell-based tissue engineering. Arthritis Res Ther. 2003;5(1):32-45. doi: 10.1186/ar614
- Singh M, Haverinen HM, Dhagat P, Jabbour GE. Inkjet printing-process and its applications. Adv Mater. 2010;22:673-685. doi: 10.1002/adma.200901141
- Stringer J, Derby B. Formation and stability of lines produced by inkjet printing. Langmuir. 2010;26:10365-10372. doi: 10.1021/la101296e
- Roth EA, Xu T, Das M, Gregory C, Hickman JJ, Boland T. Inkjet printing for high-throughput cell patterning. Biomaterials. 2004;25(17):3707-3715. doi: 10.1016/j.biomaterials.2003.10.052
- Cui X, Gao G, Qiu Y. Accelerated myotube formation using bioprinting technology for biosensor applications. Biotechnol Lett. 2013;35:315-321. doi: 10.1007/s10529-012-1087-0
- Gao Q, He Y, Fu JZ, Liu A, Ma L. Coaxial nozzle-assisted 3D bioprinting with built-in microchannels for nutrients delivery. Biomaterials. 2015;61:203-215. doi: 10.1016/j.biomaterials.2015.05.031
- Ihalainen P, Määttänen A, Sandler N. Printing technologies for biomolecule and cell-based applications. Int J Pharm. 2015;494:585-592. doi: 10.1016/j.ijpharm.2015.02.033
- Kim BS, Das S, Jang J, Cho DW. Decellularized extracellular matrix-based bioinks for engineering tissue- and organ-specific microenvironments. Chem Rev. 2020;120:10608-10661. doi: 10.1021/acs.chemrev.9b00808
- Wang Y, Yuan X, Yao B, Zhu S, Zhu P, Huang S. Tailoring bioinks of extrusion-based bioprinting for cutaneous wound healing. Bioact Mater. 2022;17:178-194. doi: 10.1016/j.bioactmat.2022.01.024.
- Mironov V. Printing technology to produce living tissue. Expert Opin Biol Ther. 2003;3:701-704. doi: 10.1517/14712598.3.5.701
- Chrisey DB. Materials processing: the power of direct writing. Science. 2000;289:879-881. doi: 10.1126/science.289.5481.879
- Bohandy J, Kim BF, Adrian FJ. Metal deposition from a supported metal film using an excimer laser. J Appl Phys. 1986;60:1538-1539. doi: 10.1063/1.337287
- Catros S, Fricain JC, Guillotin B, et al. Laser-assisted bioprinting for creating on-demand patterns of human osteoprogenitor cells and nano-hydroxyapatite. Biofabrication. 2011;3(2):025001. doi: 10.1088/1758-5082/3/2/025001
- Colina M, Serra P, Fernández-Pradas JM, Sevilla L, Morenza JL. DNA deposition through laser induced forward transfer. Biosens Bioelectron. 2005;20:1638-1642. doi: 10.1016/j.bios.2004.08.047
- Dinca V, Kasotakis E, Catherine J, et al.. Directed three-dimensional patterning of self-assembled peptide fibrils. Nano Lett. 2008;8(2):538-543. doi: 10.1021/nl072798r
- Mandrycky C, Wang Z, Kim K, Kim DH. 3D bioprinting for engineering complex tissues. Biotechnol Adv. 2016;34: 422-434. doi: 10.1016/j.biotechadv.2015.12.011
- Paruli EI, Montagna V, García-Soto M, Haupt K, Gonzato C. A general photoiniferter approach to the surface functionalization of acrylic and methacrylic structures written by two-photon stereolithography. Nanoscale. 2023;15:2860-2870. doi: 10.1039/d2nr06627k
- Li W, Wang M, Ma H, et al. Stereolithography apparatus and digital light processing-based 3D bioprinting for tissue fabrication. iScience. 2023;26:106039. doi: 10.1016/j.mser.2017.07.001
- Gauvin R, Chen YC, Lee JW, et al. Microfabrication of complex porous tissue engineering scaffolds using 3D projection stereolithography. Biomaterials. 2012;33(15):3824-3834. doi: 10.1016/j.biomaterials.2012.01.048
- Melchels FP, Feijen J, Grijpma DW. A review on stereolithography and its applications in biomedical engineering. Biomaterials 2010;31:6121-6130. doi: 10.1016/j.biomaterials.2010.04.050
- Peng W, Unutmaz D, Ozbolat IT. Bioprinting towards physiologically relevant tissue models for pharmaceutics. Trends Biotechnol. 2016;34:722-732. doi: 10.1016/j.tibtech.2016.05.013
- Kara A, Distler T, Polley C. et al. 3D printed gelatin/ decellularized bone composite scaffolds for bone tissue engineering: fabrication, characterization and cytocompatibility study. Mater Today Bio. 2022;15:100309. doi: 10.1016/j.mtbio.2022.100309
- Isaeva EV, Beketov EE, Demyashkin GA, et al. Cartilage formation in vivo using high concentration collagen-based bioink with MSC and decellularized ECM granules. Int J Mol Sci. 2022;23(5):2703. doi: 10.3390/ijms23052703
- Sorkio A, Koch L, Koivusalo L, et al. Human stem cell based corneal tissue mimicking structures using laser-assisted 3D bioprinting and functional bioinks. Biomaterials. 2018;171:57-71. doi: 10.1016/j.biomaterials.2018.04.034
- Koch L, Deiwick A, Chichkov B. Capillary-like formations of endothelial cells in defined patterns generated by laser bioprinting. Micromachines (Basel). 2021;12(12):1538. doi: 10.3390/mi12121538
- Yu C, Ma X, Zhu W, et al. Scanningless and continuous 3D bioprinting of human tissues with decellularized extracellular matrix. Biomaterials. 2019;194:1-13. doi: 10.1016/j.biomaterials.2018.12.009
- Chen P, Zheng L, Wang Y, et al. Desktop-stereolithography 3D printing of a radially oriented extracellular matrix/ mesenchymal stem cell exosome bioink for osteochondral defect regeneration. Theranostics. 2019;9(9):2439-2459. doi: 10.7150/thno.31017
- Choudhury D, Tun HW, Wang T, Naing MW. Organ-derived decellularized extracellular matrix: a game changer for bioink manufacturing? Trends Biotechnol. 2018;36(8): 787-805. doi: 10.1016/j.tibtech.2018.03.003
- Aamodt JM, Grainger DW. Extracellular matrix-based biomaterial scaffolds and the host response. Biomaterials. 2016;86:68-82. doi: 10.1016/j.biomaterials.2016.02.003
- Huang K, Li Q, Li Y, et al. Cartilage tissue regeneration: the roles of cells, stimulating factors and scaffolds. Curr Stem Cell Res Ther. 2018;13(7):547-567. doi: 10.2174/1574888X12666170608080722
- Katebifar S, Jaiswal D, Arul MR, et al. Natural polymer-based micronanostructured scaffolds for bone tissue engineering. Methods Mol Biol. 2022;2394:669-691. doi: 10.1007/978-1-0716-1811-0_35
- Miszuk J, Liang Z, Hu J, et al. An elastic mineralized 3D electrospun PCL nanofibrous scaffold for drug release and bone tissue engineering. ACS Appl Bio Mater. 2021;4(4):3639-3648. doi: 10.1021/acsabm.1c00134
- Miszuk JM, Hu J, Sun H. Biomimetic nanofibrous 3D materials for craniofacial bone tissue engineering. ACS Appl Bio Mater. 2020;3:6538-6545. doi: 10.1021/acsabm.0c00946
- Wu YA, Chiu YC, Lin YH, Ho CC, Shie MY, Chen YW. 3D-printed bioactive calcium silicate/poly-ε-caprolactone bioscaffolds modified with biomimetic extracellular matrices for bone regeneration. Int J Mol Sci. 2019;20(4):942. doi: 10.3390/ijms20040942
- Wei W, Li J, Chen S, et al. In vitro osteogenic induction of bone marrow mesenchymal stem cells with a decellularized matrix derived from human adipose stem cells and in vivo implantation for bone regeneration. J Mater Chem B. 2017;5:2468-2482. doi: 10.1039/c6tb03150a
- Yi S, Ding F, Gong L, Gu X. Extracellular matrix scaffolds for tissue engineering and regenerative medicine. Curr Stem Cell Res Ther. 2017;12:233-246. doi: 10.2174/1574888x11666160905092513
- Mahdy MAA. Skeletal muscle fibrosis: an overview. Cell Tissue Res. 2019;375:575-588. doi: 10.1007/s00441-018-2955-2
- Satyam A, Tsokos MG, Tresback JS, Zeugolis DI, Tsokos GC. Cell derived extracellular matrix-rich biomimetic substrate supports podocyte proliferation, differentiation and maintenance of native phenotype. Adv Funct Mater. 2020;30(44):1908752. doi: 10.1002/adfm.201908752
- Chan TM, Lin HP, Lin SZ. In situ altering of the extracellular matrix to direct the programming of endogenous stem cells. Stem Cells. 2014;32:1989-1990. doi: 10.1002/stem.1693
- Navaee F, Renaud P, Kleger A, Braschler T. Highly efficient cardiac differentiation and maintenance by thrombin-coagulated fibrin hydrogels enriched with decellularized porcine heart extracellular matrix. Int J Mol Sci. 2023;24(3):2842. doi: 10.3390/ijms24032842
- Lu P, Takai K, Weaver VM, Werb Z. Extracellular matrix degradation and remodeling in development and disease. Cold Spring Harb Perspect Biol. 2011;3(12): a005058. doi: 10.1101/cshperspect.a005058
- Brizzi MF, Tarone G, Defilippi P. Extracellular matrix, integrins, and growth factors as tailors of the stem cell niche. Curr Opin Cell Biol. 2012;24:645-651. doi: 10.1016/j.ceb.2012.07.001
- Velleman SG. Recent developments in breast muscle myopathies associated with growth in poultry. Annu Rev Anim Biosci. 2019;7:289-308. doi: 10.1146/annurev-animal-020518-115311
- Yu H, Mouw JK, Weaver VM. Forcing form and function: biomechanical regulation of tumor evolution. Trends Cell Biol 2011;21:47-56. doi: 10.1016/j.tcb.2010.08.015
- Shih YR, Tseng KF, Lai HY, Lin CH, Lee OK. Matrix stiffness regulation of integrin-mediated mechanotransduction during osteogenic differentiation of human mesenchymal stem cells. J Bone Miner Res. 2011;26:730-738. doi: 10.1002/jbmr.278
- Gobaa S, Hoehnel S, Roccio M, Negro A, Kobel S, Lutolf MP. Artificial niche microarrays for probing single stem cell fate in high throughput. Nat Methods. 2011;8(11):949-955. doi: 10.1038/nmeth.1732
- Sart S, Jeske R, Chen X, Ma T, Li Y. Engineering stem cell-derived extracellular matrices: decellularization, characterization, and biological function. Tissue Eng Part B Rev. 2020;26:402-422. doi: 10.1089/ten.TEB.2019.0349
- Wanjare M, Agarwal N, Gerecht, S. Biomechanical strain induces elastin and collagen production in human pluripotent stem cell-derived vascular smooth muscle cells. Am J Physiol Cell Physiol. 2015;309:C271-C281. doi: 10.1152/ajpcell.00366.2014
- Crapo PM, Gilbert TW, Badylak SF. An overview of tissue and whole organ decellularization processes. Biomaterials. 2011;32(12):3233-3243. doi: 10.1016/j.biomaterials.2011.01.057
- Nagata S, Hanayama R, Kawane K. Autoimmunity and the clearance of dead cells. Cell. 2010;140(5):619-630. doi: 10.1016/j.cell.2010.02.014
- Zheng MH, Chen J, Kirilak Y, Willers C, Xu J, Wood D. Porcine small intestine submucosa (SIS) is not an acellular collagenous matrix and contains porcine DNA: possible implications in human implantation. J Biomed Mater Res B Appl Biomater. 2005;73(1):61-67. doi: 10.1002/jbm.b.30170
- Badylak SF, Taylor D, Uygun K. Whole-organ tissue engineering: decellularization and recellularization of three-dimensional matrix scaffolds. Annu Rev Biomed Eng. 2011;13:27-53. doi: 10.1146/annurev-bioeng-071910-124743
- Buckenmeyer MJ, Meder TJ, Prest TA, Brown BN. Decellularization techniques and their applications for the repair and regeneration of the nervous system. Methods. 2020;171:41-61. doi: 10.1016/j.ymeth.2019.07.023
- Gilbert TW, Sellaro TL, Badylak SF. Decellularization of tissues and organs. Biomaterials. 2006;27:3675-3683. doi: 10.1016/j.biomaterials.2006.02.014
- Mendibil U, Ruiz-Hernandez R, Retegi-Carrion S, Garcia- Urquia N, Olalde-Graells B, Abarrategi A. Tissue-specific decellularization methods: rationale and strategies to achieve regenerative compounds. Int J Mol Sci. 2020;21(15):5447. doi: 10.3390/ijms21155447
- Hung SH, Su CH, Lee FP, Tseng H. Larynx decellularization: combining freeze-drying and sonication as an effective method. J Voice. 2013;27:289-294. doi: 10.1016/j.jvoice.2013.01.018
- Kim BS, Das S, Jang J, Cho D.-W. Decellularized extracellular matrix-based bioinks for engineering tissue-and organ-specific microenvironments. Chem Rev. 2020;120:10608-10661.
- Nonaka PN, Campillo N, Uriarte JJ, et al. Effects of freezing/ thawing on the mechanical properties of decellularized lungs. J Biomed Mater Res A. 2014;102(2):413-419. doi: 10.1002/jbm.a.34708
- Funamoto S, Nam K, Kimura T, et al. The use of high-hydrostatic pressure treatment to decellularize blood vessels. Biomaterials. 2010;31(13):3590-3595. doi: 10.1016/j.biomaterials.2010.01.073
- Hashimoto Y, Funamoto S, Sasaki S, et al. Preparation and characterization of decellularized cornea using high-hydrostatic pressurization for corneal tissue engineering. Biomaterials. 2010;31(14):3941-3948. doi: 10.1016/j.biomaterials.2010.01.122
- Naso F, Gandaglia A. Can heart valve decellularization be standardized? A review of the parameters used for the quality control of decellularization processes. Front Bioeng Biotechnol. 2022;10:830899. doi: 10.3389/fbioe.2022.830899
- Zubarevich A, Osswald A, Amanov L, et al. Development and evaluation of a novel combined perfusion decellularization heart-lung model for tissue engineering of bioartificial heart-lung scaffolds. Artif Organs. 2023;47(3):481-489. doi: 10.1111/aor.14419
- Kim YS, Majid M, Melchiorri AJ, Mikos AG. Applications of decellularized extracellular matrix in bone and cartilage tissue engineering. Bioeng Transl Med. 2019;4: 83-95. doi: 10.1002/btm2.10110
- Zhang CY, Fu CP, Li XY, et al. Three-dimensional bioprinting of decellularized extracellular matrix-based bioinks for tissue engineering. Molecules. 2022;27(11): 3442. doi: 10.3390/molecules27113442
- Procházková A, Poláchová M, Dítě J, Netuková M, Studený P. Chemical, physical, and biological corneal decellularization methods: a review of literature. J Ophthalmol. 2024;2024:1191462. doi: 10.1155/2024/1191462
- Wang B, Qinglai T, Yang Q, et al. Functional acellular matrix for tissue repair. Mater Today Bio. 2022;18:100530. doi: 10.1016/j.mtbio.2022.100530
- Reing JE, Brown BN, Daly KA, et al. The effects of processing methods upon mechanical and biologic properties of porcine dermal extracellular matrix scaffolds. Biomaterials. 2010;31(33):8626-8633. doi: 10.1016/j.biomaterials.2010.07.083
- Belviso I, Sacco AM, Cozzolino D, et al. Cardiac-derived extracellular matrix: A decellularization protocol for heart regeneration. PLoS One. 2022;17(10):e0276224. doi: 10.1371/journal.pone.0276224
- Willemse J, Verstegen MMA, Vermeulen A, et al. Fast, robust and effective decellularization of whole human livers using mild detergents and pressure controlled perfusion. Mater Sci Eng C Mater Biol Appl. 2020;108:110200. doi: 10.1016/j.msec.2019.110200
- Bongolan T, Whiteley J, Castillo-Prado J, et al. Decellularization of porcine kidney with submicellar concentrations of SDS results in the retention of ECM proteins required for the adhesion and maintenance of human adult renal epithelial cells. Biomater Sci. 2022;10(11):2972-2990
- Alshaikh AB, Padma AM, Dehlin M, et al. Decellularization and recellularization of the ovary for bioengineering applications; studies in the mouse. Reprod Biol Endocrinol. 2020;18:75. doi: 10.1186/s12958-020-00630-y
- Naik A, Griffin M, Szarko M, Butler PE. Optimizing the decellularization process of an upper limb skeletal muscle; implications for muscle tissue engineering. Artif Organs. 2020;44:178-183. doi: 10.1111/aor.13575
- Kasravi M, Ahmadi A, Babajani A, et al. Immunogenicity of decellularized extracellular matrix scaffolds: a bottleneck in tissue engineering and regenerative medicine. Biomater Res. 2023;27:10. doi: 10.1186/s40824-023-00348-z
- Abaci A, Guvendiren M. Designing decellularized extracellular matrix-based bioinks for 3D bioprinting. Adv Healthc Mater. 2020;9:e2000734. doi: 10.1002/adhm.202000734
- Giraldo-Gomez DM, Leon-Mancilla B, Del Prado- Audelo ML, et al. Trypsin as enhancement in cyclical tracheal decellularization: morphological and biophysical characterization. Mater Sci Eng C Mater Biol Appl. 2016;59:930-937. doi: 10.1016/j.msec.2015.10.094
- Yang Y, Lin H, Shen H, Wang B, Lei G, Tuan RS. Mesenchymal stem cell-derived extracellular matrix enhances chondrogenic phenotype of and cartilage formation by encapsulated chondrocytes in vitro and in vivo. Acta Biomater. 2018;69:71-82. doi: 10.1016/j.actbio.2017.12.043
- Liguori GR, Liguori TTA, de Moraes SR, et al. Molecular and biomechanical clues from cardiac tissue decellularized extracellular matrix drive stromal cell plasticity. Front Bioeng Biotechnol. 2020;8:520. doi: 10.3389/fbioe.2020.00520
- Jang J, Park HJ, Kim SW, et al. 3D printed complex tissue construct using stem cell-laden decellularized extracellular matrix bioinks for cardiac repair. Biomaterials. 2017;112: 264-274. doi: 10.1016/j.biomaterials.2016.10.026
- Wang X, Pierre V, Liu C, Senapati S, Park PS, Senyo SE. Exogenous extracellular matrix proteins decrease cardiac fibroblast activation in stiffening microenvironment through CAPG. J Mol Cell Cardiol. 2021;159:105-119. doi: 10.1016/j.yjmcc.2021.06.001
- Mesquita FCP, Morrissey J, Lee P-F, et al. Cues from human atrial extracellular matrix enrich the atrial differentiation of human induced pluripotent stem cell-derived cardiomyocytes. Biomater Sci. 2021;9:3737-3749. doi: 10.1039/d0bm01686a
- Choi YJ, Park SJ, Yi H-G, et al. Muscle-derived extracellular matrix on sinusoidal wavy surfaces synergistically promotes myogenic differentiation and maturation. J Mater Chem B. 2018;6:5530-5539. doi: 10.1039/c8tb01475b
- Choi YJ, Jun YJ, Kim DY, et al. A 3D cell printed muscle construct with tissue-derived bioink for the treatment of volumetric muscle loss. Biomaterials. 2019;206: 160-169. doi: 10.1016/j.biomaterials.2019.03.036
- Kim W, Lee H, Lee J, et al. Efficient myotube formation in 3D bioprinted tissue construct by biochemical and topographical cues. Biomaterials. 2020;230:119632. doi: 10.1016/j.biomaterials.2019.119632
- Zhao F, Cheng J, Sun M, et al. Digestion degree is a key factor to regulate the printability of pure tendon decellularized extracellular matrix bio-ink in extrusion-based 3D cell printing. Biofabrication. 2020;12:045011. doi: 10.1088/1758-5090/aba411
- Zhao F, Cheng J, Zhang J, et al. Comparison of three different acidic solutions in tendon decellularized extracellular matrix bio-ink fabrication for 3D cell printing. Acta Biomater. 2021;131:262-275. doi: 10.1016/j.actbio.2021.06.026
- Zhang X, Song W, Han K, et al. Three-dimensional bioprinting of a structure-, composition-, and mechanics-graded biomimetic scaffold coated with specific decellularized extracellular matrix to improve the tendon-to-bone healing. ACS Appl Mater Interfaces. 2023;15:28964-28980. doi: 10.1021/acsami.3c03793
- Lee J, Hong J, Kim W, Kim GH. Bone-derived dECM/ alginate bioink for fabricating a 3D cell-laden mesh structure for bone tissue engineering. Carbohydr Polym. 2020;250:116914. doi: 10.1016/j.carbpol.2020.116914
- Parthiban SP, Athirasala A, Tahayeri A, et al. BoneMA-synthesis and characterization of a methacrylated bone-derived hydrogel for bioprinting ofin-vitrovascularized tissue constructs. Biofabrication. 2021;13(3):10.1088/1758- 5090/abb11f.doi: 10.1088/1758-5090/abb11f
- Zhang X, Liu Y, Zuo Q, et al. 3D bioprinting of biomimetic bilayered scaffold consisting of decellularized extracellular matrix and silk fibroin for osteochondral repair. Int J Bioprint. 2021;7(4):401. doi: 10.18063/ijb.v7i4.401
- Hwangbo H, Lee J, Kim G. Mechanically and biologically enhanced 3D-printed HA/PLLA/dECM biocomposites for bone tissue engineering. Int J Biol Macromol. 2022; 218:9-21. doi: 10.1016/j.ijbiomac.2022.07.040
- Zhu S, Chen P, Chen Y, Li M, Chen C, Lu H. 3D-printed extracellular matrix/polyethylene glycol diacrylate hydrogel incorporating the anti-inflammatory phytomolecule honokiol for regeneration of osteochondral defects. Am J Sports Med. 2020;48(11):2808-2818. doi: 10.1177/0363546520941842
- Chae S, Lee SS, Choi YJ, et al. 3D cell-printing of biocompatible and functional meniscus constructs using meniscus-derived bioink. Biomaterials. 2021;267:120466. doi: 10.1016/j.biomaterials.2020.120466
- Setayeshmehr M, Hafeez S, van Blitterswijk C, Moroni L, Mota C, Baker MB. Bioprinting via a dual-gel bioink based on poly(vinyl alcohol) and solubilized extracellular matrix towards cartilage engineering. Int J Mol Sci. 2021;22(8):3901. doi: 10.3390/ijms22083901
- Visscher DO, Lee H, van Zuijlen PPM, et al. A photo-crosslinkable cartilage-derived extracellular matrix bioink for auricular cartilage tissue engineering. Acta Biomater. 2021;121:193-203. doi: 10.1016/j.actbio.2020.11.029
- Lu J, Huang J, Jin J, et al. The design and characterization of a strong bio-ink for meniscus regeneration. Int J Bioprint. 2022;8(4):600. doi: 10.18063/ijb.v8i4.600
- Nouri Barkestani M, Naserian S, Uzan G, Shamdani S. Post-decellularization techniques ameliorate cartilage decellularization process for tissue engineering applications. J Tissue Eng. 2021;12:2041731420983562. doi: 10.1177/2041731420983562
- McInnes AD, Moser MAJ, Chen X. Preparation and use of decellularized extracellular matrix for tissue engineering. J Funct Biomater. 2022;13(4):240. doi: 10.3390/jfb13040240
- Al-Hakim Khalak F, García-Villén F, Ruiz-Alonso S, Pedraz JL, Saenz-Del-Burgo L. Decellularized extracellular matrix-based bioinks for tendon regeneration in three-dimensional bioprinting. Int J Mol Sci. 2022;23(21):12930. doi: 10.3390/ijms232112930
- Li S, Liu Z, Gao X, et al. Preparation and properties of a 3D printed nHA/PLA bone tissue engineering scaffold loaded with a β-CD-CHX combined dECM hydrogel. RSC Adv. 2024;14:9848-9859. doi: 10.1039/d4ra00261j
- Hussain Z, Ding P, Zhang L, Zhang Y. Multifaceted tannin crosslinked bioinspired dECM decorated nanofibers modulating cell-scaffold biointerface for tympanic membrane perforation bioengineering. Biomed Mater 2022;17(3):1. doi: 10.1088/1748-605X/ac6125
- Wu J, Han Y, Fu Q, et al. Application of tissue-derived bioink for articular cartilage lesion repair. Chem Eng J. 2022;450:8-9. doi: 10.1016/j.cej.2022.138292
- Yoo SJ, Hussein N, Peel B, et al. 3D modeling and printing in congenital heart surgery: entering the stage of maturation. Front Pediatr. 2021;9:621672. doi: 10.3389/fped.2021.621672
- Zhe M, Wu X, Yu P, et al. Recent advances in decellularized extracellular matrix-based bioinks for 3D bioprinting in tissue engineering. Materials (Basel). 2023;16(8):3197. doi: 10.3390/ma16083197
- Xiang Y, Miller K, Guan J, Kiratitanaporn W, Tang M, Chen S. 3D bioprinting of complex tissues in vitro: state-of-the-art and future perspectives. Arch Toxicol. 2022;96(3):691-710. doi: 10.1007/s00204-021-03212-y
- Jang J, Kim TG, Kim BS, Kim SW, Kwon SM, Cho DW. Tailoring mechanical properties of decellularized extracellular matrix bioink by vitamin B2-induced photo-crosslinking. Acta Biomater. 2016;33:88-95. doi: 10.1016/j.actbio.2016.01.013
- Sung K, Patel NR, Ashammakhi N, Nguyen KL. 3-Dimensional bioprinting of cardiovascular tissues: emerging technology. JACC Basic Transl Sci. 2021;6: 467-482. doi: 10.1016/j.jacbts.2020.12.006
- Sanz-Fraile H, Herranz-Diez C, Ulldemolins A, et al. Characterization of bioinks prepared via gelifying extracellular matrix from decellularized porcine myocardia. Gels. 2023;9(9):745. doi: 10.3390/gels9090745
- Ostrovidov S, Hosseini V, Ahadian S, et al. Skeletal muscle tissue engineering: methods to form skeletal myotubes and their applications. Tissue Eng Part B Rev. 2014;20(5): 403-436. doi: 10.1089/ten.TEB.2013.0534
- Behre A, Tashman JW, Dikyol C, et al. 3D bioprinted patient-specific extracellular matrix scaffolds for soft tissue defects. Adv Healthc Mater. 2022;11(24):e2200866. doi: 10.1002/adhm.202200866
- Wang D, Zhang X, Huang S, et al. Engineering multi-tissue units for regenerative medicine: bone-tendon-muscle units of the rotator cuff. Biomaterials. 2021;272:120789. doi: 10.1016/j.biomaterials.2021.120789
- Yoshimoto Y, Oishi Y. Mechanisms of skeletal muscle-tendon development and regeneration/healing as potential therapeutic targets. Pharmacol Ther. 2023;243:108357. doi: 10.1016/j.pharmthera.2023.108357
- Xu Y, Murrell GA. The basic science of tendinopathy. Clin Orthop Relat Res. 2008;466:1528-1538. doi: 10.1007/s11999-008-0286-4
- Andres BM, Murrell GA. Treatment of tendinopathy: what works, what does not, and what is on the horizon. Clin Orthop Relat Res. 2008;466:1539-1554. doi: 10.1007/s11999-008-0260-1
- Lui PP. Stem cell technology for tendon regeneration: current status, challenges, and future research directions. Stem Cells Cloning. 2015;8:163-174. doi: 10.2147/sccaa.S60832
- Migliorini F, Tingart M, Maffulli N. Progress with stem cell therapies for tendon tissue regeneration. Expert Opin Biol Ther. 2020;20:1373-1379. doi: 10.1080/14712598.2020.1786532
- No YJ, Castilho M, Ramaswamy Y, Zreiqat, H. Role of biomaterials and controlled architecture on tendon/ligament repair and regeneration. Adv Mater. 2020;32:e1904511. doi: 10.1002/adma.201904511
- Liu Y., Ramanath HS, Wang DA. Tendon tissue engineering using scaffold enhancing strategies. Trends Biotechnol. 2008;26:201-209. doi: 10.1016/j.tibtech.2008.01.003
- Anjum S, Li T, Saeed M, Ao Q. Exploring polysaccharide and protein-enriched decellularized matrix scaffolds for tendon and ligament repair: a review. Int J Biol Macromol. 2024;254:127891. doi: 10.1016/j.ijbiomac.2023.127891
- Toprakhisar B, Nadernezhad A, Bakirci E, Khani N, Skvortsov GA, Koc B. Development of bioink from decellularized tendon extracellular matrix for 3d bioprinting. Macromol Biosci. 2018;18(10):e1800024. doi: 10.1002/mabi.201800024
- Chae S, Choi YJ, Cho DW. Mechanically and biologically promoted cell-laden constructs generated using tissue-specific bioinks for tendon/ligament tissue engineering applications. Biofabrication. 2022;14(2):025013. doi: 10.1088/1758-5090/ac4fb6
- Kim D, Kim GH. Bioprinted hASC-laden cell constructs with mechanically stable and cell alignment cue for tenogenic differentiation. Biofabrication. 2023;15(4):1. doi: 10.1088/1758-5090/ace740
- Balestri W, Morris RH, Hunt JA, Reinwald Y. Current advances on the regeneration of musculoskeletal interfaces. Tissue Eng Part B Rev. 2021;27:548-571. doi: 10.1089/ten.TEB.2020.0112
- Bonnevie ED, Mauck RL. Physiology and engineering of the graded interfaces of musculoskeletal junctions. Annu Rev Biomed Eng. 2018;20:403-429. doi: 10.1146/annurev-bioeng-062117-121113
- Chae S, Sun Y, Choi YJ, Ha DH, Jeon I, Cho DW. 3D cell-printing of tendon-bone interface using tissue-derived extracellular matrix bioinks for chronic rotator cuff repair. Biofabrication. 2021;13(3):1-2,27. doi: 10.1088/1758-5090/abd159
- Chae S, Yong U, Park W, et al. 3D cell-printing of gradient multi-tissue interfaces for rotator cuff regeneration. Bioact Mater. 2022;19:611-625. doi: 10.1016/j.bioactmat.2022.05.004
- Liu H, Yang L, Zhang E, et al. Biomimetic tendon extracellular matrix composite gradient scaffold enhances ligament-to-bone junction reconstruction. Acta Biomater. 2017;56:129-140. doi: 10.1016/j.actbio.2017.05.027
- Shengnan Q, Bennett S, Wen W, Aiguo L, Jiake X. The role of tendon derived stem/progenitor cells and extracellular matrix components in the bone tendon junction repair. Bone. 2021;153:116172. doi: 10.1016/j.bone.2021.116172
- Tong S, Sun Y, Kuang B, et al. A comprehensive review of muscle-tendon junction: structure, function, injury and repair. Biomedicines. 2024;12(2):423. doi: 10.3390/biomedicines12020423
- Kim WJ, Kim GH. A bioprinted complex tissue model for myotendinous junction with biochemical and biophysical cues. Bioeng Transl Med. 2022;7(3):e10321. doi: 10.1002/btm2.10321
- Badar W, Ali H, Brooker ON, et al. Collagen pre-strain discontinuity at the bone-Cartilage interface. PLoS One. 2022;17(9):e0273832. doi: 10.1371/journal.pone.0273832
- Xu J, Ji J, Jiao J, et al. 3D printing for bone-cartilage interface regeneration. Front Bioeng Biotechnol. 2022;10:828921. doi: 10.3389/fbioe.2022.828921
- Terpstra ML, Li J, Mensinga A, et al. Bioink with cartilage-derived extracellular matrix microfibers enables spatial control of vascular capillary formation in bioprinted constructs. Biofabrication. 2022;14(3). doi: 10.1088/1758-5090/ac6282
- Zhang Y, Wu D, Zhao X, et al. Stem cell-friendly scaffold biomaterials: applications for bone tissue engineering and regenerative medicine. Front Bioeng Biotechnol. 2020;8:598607. doi: 10.3389/fbioe.2020.598607
- Kim D, Lee H, Lee GH, Hoang TH, Kim HR, Kim GH. Fabrication of bone-derived decellularized extracellular matrix/ceramic-based biocomposites and their osteo/ odontogenic differentiation ability for dentin regeneration. Bioeng Transl Med. 2022;7(3):e10317. doi: 10.1002/btm2.10317
- Hung BP, Naved BA, Nyberg EL, et al. Three-dimensional printing of bone extracellular matrix for craniofacial regeneration. ACS Biomater Sci Eng. 2016;2(10):1806-1816. doi: 10.1021/acsbiomaterials.6b00101
- Kang Y, Xu J, Meng L, et al. 3D bioprinting of dECM/Gel/ QCS/nHAp hybrid scaffolds laden with mesenchymal stem cell-derived exosomes to improve angiogenesis and osteogenesis. Biofabrication. 2023;15(2). doi: 10.1088/1758-5090/acb6b8.
- Lammi MJ, Piltti J, Prittinen J, Qu C. Challenges in fabrication of tissue-engineered cartilage with correct cellular colonization and extracellular matrix assembly. Int J Mol Sci. 2018;19(9):2700. doi: 10.3390/ijms19092700
- Ahmed TA, Hincke MT. Strategies for articular cartilage lesion repair and functional restoration. Tissue Eng Part B Rev. 2010;16:305-329. doi: 10.1089/ten.TEB.2009.0590
- Hogan KJ, Öztatlı H, Perez MR, et al. Development of photoreactive demineralized bone matrix 3D printing colloidal inks for bone tissue engineering. Regen Biomater. 2023;10:rbad090. doi: 10.1093/rb/rbad090
- Yuan Z, Lyu Z, Liu X, Zhang J, Wang Y. Mg-BGNs/DCECM composite scaffold for cartilage regeneration: a preliminary in vitro study. Pharmaceutics. 2021;13(10):1550. doi: 10.3390/pharmaceutics13101550
- Behan K, Dufour A, Garcia O, Kelly D. Methacrylated cartilage ECM-based hydrogels as injectables and bioinks for cartilage tissue engineering. Biomolecules. 2022; 12(2):216. doi: 10.3390/biom12020216
- Yang Z, Zhao T, Gao C, et al. 3D-bioprinted difunctional scaffold for in situ cartilage regeneration based on aptamer-directed cell recruitment and growth factor-enhanced cell chondrogenesis. ACS Appl Mater Interfaces. 2021;13(20):23369-23383
- Meng X, Zhou Z, Chen X, et al. A sturgeon cartilage extracellular matrix-derived bioactive bioink for tissue engineering applications. Int J Bioprint. 2023;9(5):768. doi: 10.18063/ijb.768
- Yang Z, Cao F, Li H, et al. Microenvironmentally optimized 3D-printed TGFβ-functionalized scaffolds facilitate endogenous cartilage regeneration in sheep. Acta Biomater. 2022;150:181-198. doi: 10.1016/j.actbio.2022.07.029
- Zhang H, Wang Y, Zheng Z, et al. Strategies for improving the 3D printability of decellularized extracellular matrix bioink. Theranostics. 2023;13(8):2562-2587. doi: 10.7150/thno.81785
- Seok JM, Ahn M, Kim D, Lee JS. Decellularized matrix bioink with gelatin methacrylate for simultaneous improvements in printability and biofunctionality. Int J Biol Macromol. 2024;262:130194. doi: 10.1016/j.ijbiomac.2024.130194
- Won JY, Lee MH, Kim MJ, et al. A potential dermal substitute using decellularized dermis extracellular matrix derived bio-ink. Artif Cells Nanomed Biotechnol. 2019;47(1): 644-649. doi: 10.1080/21691401.2019.1575842
- Tan YH, Helms HR, Nakayama KH. Decellularization strategies for regenerating cardiac and skeletal muscle tissues. Front Bioeng Biotechnol. 2022;10:831300. doi: 10.3389/fbioe.2022.831300
- Saldin LT, Cramer MC, Velankar SS, White LJ, Badylak SF. Extracellular matrix hydrogels from decellularized tissues: structure and function. Acta Biomater. 2017;49:1-15. doi: 10.1016/j.actbio.2016.11.068
- Yuan Z, Liu S, Hao C, et al. AMECM/DCB scaffold prompts successful total meniscus reconstruction in a rabbit total meniscectomy model. Biomaterials. 2016;111:13-26. doi: 10.1016/j.biomaterials.2016.09.017
- Silva AC, Rodrigues SC, Caldeira J, et al. Three-dimensional scaffolds of fetal decellularized hearts exhibit enhanced potential to support cardiac cells in comparison to the adult. Biomaterials. 2016;104:52-64. doi: 10.1016/j.biomaterials.2016.06.062
- Robertson MJ, Dries-Devlin JL, Kren SM, Burchfield JS, Taylor DA. Optimizing recellularization of whole decellularized heart extracellular matrix. PLoS One. 2014;9(2):e90406. doi: 10.1371/journal.pone.0090406
- Kobayashi M, Kadota J, Hashimoto Y, et al. Elastic modulus of ECM hydrogels derived from decellularized tissue affects capillary network formation in endothelial cells. Int J Mol Sci. 2020;21(17):6304. doi: 10.3390/ijms21176304
- Radeke C, Pons R, Mihajlovic M, et al. Transparent and cell-guiding cellulose nanofiber 3d printing bioinks. ACS Appl Mater Interfaces. 2023;15(2): 2564-2577. doi: 10.1021/acsami.2c16126
- Arezoo N, Mohammad H, Malihezaman M. Tissue engineering of mouse uterus using menstrual blood stem cells (MenSCs) and decellularized uterine scaffold. Stem Cell Res Ther. 2021;12(1):475. doi: 10.1186/s13287-021-02543-y.
- Brown M, Li J, Moraes C, Tabrizian M, Li-Jessen NYK. Decellularized extracellular matrix: new promising and challenging biomaterials for regenerative medicine. Biomaterials. 2022;289:121786. doi: 10.1016/j.biomaterials.2022.12178
- Han H, Kim M, Yong U, et al. Tissue-specific gelatin bioink as a rheology modifier for high printability and adjustable tissue properties. Biomater Sci. 2024;12: 2599-2613. doi: 10.1039/d3bm02111d
- Lian L, Xie M, Luo Z, et al. Rapid volumetric bioprinting of decellularized extracellular matrix bioinks. Adv Mater. 2024;e2304846. doi: 10.1002/adma.202304846
- Lee H, Ju YM, Kim I, et al. A novel decellularized skeletal muscle-derived ECM scaffolding system for in situ muscle regeneration. Methods. 2020;171:77-85. doi: 10.1016/j.ymeth.2019.06.027
- Witt R, Weigand A, Boos AM, et al. Mesenchymal stem cells and myoblast differentiation under HGF and IGF-1 stimulation for 3D skeletal muscle tissue engineering. BMC Cell Biol. 2017;18(1):15. doi: 10.1186/s12860-017-0131-2
- Sani M, Hosseinie R, Latifi M, et al. Engineered artificial articular cartilage made of decellularized extracellular matrix by mechanical and IGF-1 stimulation. Biomater Adv. 2022;139:213019. doi: 10.1016/j.bioadv.2022.213019
- Su X, Wang T, Guo S. Applications of 3D printed bone tissue engineering scaffolds in the stem cell field. Regen Ther. 2021;16:63-72. doi: 10.1016/j.reth.2021.01.007
- Iwasaki N, Roldo M, Karali A, Blunn G. In vitro development of a muscle-tendon junction construct using decellularised extracellular matrix: effect of cyclic tensile loading. Biomater Adv. 2024;161:213873. doi: 10.1016/j.bioadv.2024.213873
- Li J, Zhang J, Ye H, et al. Pulmonary decellularized extracellular matrix (dECM) modified polyethylene terephthalate three-dimensional cell carriers regulate the proliferation and paracrine activity of mesenchymal stem cells. Front Bioeng Biotechnol. 2024;11:1324424. doi: 10.3389/fbioe.2023.1324424
- Potere F, Belgio B, Croci GA, et al. 3D bioprinting of multi-layered segments of a vessel-like structure with ECM and novel derived bioink. Front Bioeng Biotechnol. 2022;10:918690. doi: 10.3389/fbioe.2022.918690
- Ong CS, Nam L, Ong K, et al. 3D and 4D bioprinting of the myocardium: current approaches, challenges, and future prospects. Biomed Res Int. 2018;2018:6497242. doi: 10.1155/2018/6497242