A perspective on transformative bioprinting
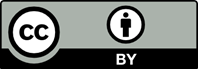
Our understanding of bioprinting originated from tissue engineering and 3D printing. Over the last two decades, 3D printing has been serving tissue engineering to fulfill its goal of solving the worldwide challenge of human organ shortage. Fundamental research and translation to clinical settings are currently the mainstream and have led to the emergence of translational bioprinting. However, as bioprinting evolves with more refined capabilities such as spatial and temporal controls of multiple biological materials at the microscale, perhaps it is time to reflect on a reciprocal approach, i.e., tissue engineering, to serve 3D printing with the hope to address problems more than or other than organ shortage. Recent examples of cultivated meat, digital bioprinting, bioelectronics, and space exploration may have just revealed the tip of the iceberg, though some still overlap with the goal of tissue engineering. Whenever a new material is introduced to the realm of 3D printing and becomes printable, it always leads to versatile or even unforeseen applications. This has happened to metals, ceramics, composite, and electronics. Although it has been known for a long time that biological cells are 3D-printable, the application of such concept has been always perceived from the angle of tissue engineering with human organ shortage as the sole motivation. Therefore, in this review based on existing evidence, we offer an innovative perspective of bioprinting, termed “transformative bioprinting,” to emphasize the role of an enabling tool of bioprinting and its alternative motivations.
- Yeong W-Y, Chua C-K, Leong K-F, Chandrasekaran M. Rapid prototyping in tissue engineering: challenges and potential. Trends Biotechnol. 2004;22(12):643-652. doi: 10.1016/j.tibtech.2004.10.004
- Yeong W, Sudarmadji N, Yu H, et al. Porous polycaprolactone scaffold for cardiac tissue engineering fabricated by selective laser sintering. Acta Biomater. 2010;6(6):2028-2034. doi: 10.1016/j.actbio.2009.12.033
- Mironov V, Kasyanov V, Drake C, Markwald RR. Organ printing: promises and challenges Regen Med. 2008; 3(1):93-103. doi: 10.2217/17460751.3.1.93
- Mironov V, Boland T, Trusk T, Forgacs G, Markwald RR. Organ printing: computer-aided jet-based 3D tissue engineering. Trends Biotechnol. 2003;21(4):157-161. doi: 10.1016/S0167-7799(03)00033-7
- Ng WL, An J, Chua CK. Process, material, and regulatory considerations for 3D printed medical devices and tissue constructs. Engineering. 2024. doi: 10.1016/j.eng.2024.01.028
- Murphy SV, De Coppi P, Atala A. Opportunities and challenges of translational 3D bioprinting. Nat Biomed Eng. 2020;4(4):370-380. doi: 10.1038/s41551-019-0471-7
- O’Connell CD, Dalton PD, Hutmacher DW. Why bioprinting in regenerative medicine should adopt a rational technology readiness assessment. Trends Biotechnol. 2024. doi: 10.1016/j.tibtech.2024.03.006
- He J, Mao M, Li X, Chua CK. Bioprinting of 3D functional tissue constructs. Int J Bioprint. 2021;7(3):1-2. doi: 10.18063/ijb.v7i3.395
- Bertassoni LE. Bioprinting of complex multicellular organs with advanced functionality—recent progress and challenges ahead. Adv Mater. 2022;34(3):e2101321. doi: 10.1002/adma.202101321
- Lee J, Park D, Seo Y, Chung JJ, Jung Y, Kim SH. Organ‐level functional 3D tissue constructs with complex compartments and their preclinical applications. Adv Mater. 2020;32(51):e2002096. doi: 10.1002/adma.202002096
- Dutta SD, Ganguly K, Jeong M-S, et al. Bioengineered lab-grown meat-like constructs through 3D bioprinting of antioxidative protein hydrolysates. ACS Appl Mater Interfaces. 2022;14(30):34513-34526. doi: 10.1021/acsami.2c10620
- Beckwith AL, Borenstein JT, Velásquez-García LF. Physical, mechanical, and microstructural characterization of novel, 3D-printed, tunable, lab-grown plant materials generated from Zinnia elegans cell cultures. Mater Today. 2022;54:27-41. doi: 10.1016/j.mattod.2022.02.012
- Simon DT, Gabrielsson EO, Tybrandt K, Berggren M. Organic bioelectronics: bridging the signaling gap between biology and technology. Chem. Rev. 2016;116(21): 13009-13041. doi: 10.1021/acs.chemrev.6b00146
- Zhang H, Wen H, Zhu J, Xia Z, Zhang Z. 3D printing dielectric elastomers for advanced functional structures: a mini‐review. J Appl Polym Sci. 2024; 141(15):e55015. doi: 10.1002/app.55015
- Sarabi MR, Yetisen AK, Tasoglu S. Magnetic levitation for space exploration. Trends Biotechnol. 2022;40(8):915-917. doi: 10.1016/j.tibtech.2022.03.010
- Landerneau S, Lemarié L, Marquette C, Petiot E. Green 3D bioprinting of plant cells: a new scope for 3D bioprinting. Bioprinting. 2022;27:e00216. doi: 10.1016/j.bprint.2022.e00216
- Whenish R, Ramakrishna S, Jaiswal AK, Manivasagam G. A framework for the sustainability implications of 3D bioprinting through nature-inspired materials and structures. Bio-Design Manuf. 2022;5(2):412-423. doi: 10.1007/s42242-021-00168-x
- Zhou D, Dou B, Kroh F, Wang C, Ouyang L. Biofabrication strategies with single-cell resolution: a review. Int J Extreme Manuf. 2023;5(4):042005. doi: 10.1088/2631-7990/ace863
- Hinton TJ, Jallerat Q, Palchesko RN, et al. Three-dimensional printing of complex biological structures by freeform reversible embedding of suspended hydrogels. Sci Adv. 2015;1(9):e1500758. doi: 10.1126/sciadv.1500758
- Bernal PN, Delrot P, Loterie D, et al. Volumetric bioprinting of complex living‐tissue constructs within seconds. Adv Mater. 2019;31(42):e1904209. doi: 10.1002/adma.201904209
- Ouyang L. Pushing the rheological and mechanical boundaries of extrusion-based 3D bioprinting. Trends Biotechnol. 2022;40(7):891-902. doi: 10.1016/j.tibtech.2022.01.001
- Wu Y, Yang X, Gupta D, et al. Dissecting the interplay mechanism among process parameters toward the biofabrication of high-quality shapes in embedded bioprinting. Adv Funct Mater. 2024;34(21):2313088. doi: 10.1002/adfm.202313088
- Highley CB, Song KH, Daly AC, Burdick JA. Jammed microgel inks for 3D printing applications. Adv Sci. 2019;6(1):1801076. doi: 10.1002/advs.201801076
- Yuan X, Wang Z, Che L, et al. Recent developments and challenges of 3D bioprinting technologies. Int J Bioprinting. 2024;10(2):1752. doi: doi.org/10.36922/ijb.1752
- Pitacco P, Sadowska JM, O’Brien FJ, Kelly DJ. 3D bioprinting of cartilaginous templates for large bone defect healing. Acta Biomater. 2023;156:61-74. doi: 10.1016/j.actbio.2022.07.037
- Xie M, Shi Y, Zhang C, et al. In situ 3D bioprinting with bioconcrete bioink. Nature communications. 2022;13(1):3597. doi: 10.1038/s41467-022-30997-y
- Sun Y, You Y, Jiang W, Wang B, Wu Q, Dai K. 3D bioprinting dual-factor releasing and gradient-structured constructs ready to implant for anisotropic cartilage regeneration. Sci Adv. 2020;6(37):eaay1422. doi: 10.1126/sciadv.aay1422
- Zhang X, Liu Y, Zuo Q, et al. 3D bioprinting of biomimetic bilayered scaffold consisting of decellularized extracellular matrix and silk fibroin for osteochondral repair. Int J Bioprint. 2021;7(4):401. doi: 10.18063/ijb.v7i4.401
- Albanna M, Binder KW, Murphy SV, et al. In situ bioprinting of autologous skin cells accelerates wound healing of extensive excisional full-thickness wounds. Sci Rep. 2019;9(1):1856. doi: 10.1038/s41598-018-38366-w
- Yang Y, Xu R, Wang C, Guo Y, Sun W, Ouyang L. Recombinant human collagen-based bioinks for the 3D bioprinting of full-thickness human skin equivalent. Int J Bioprint. 2022;8(4):611. doi: 10.18063/ijb.v8i4.611
- Noor N, Shapira A, Edri R, Gal I, Wertheim L, Dvir T. 3D printing of personalized thick and perfusable cardiac patches and hearts. Adv Sci. 2019;6(11):1900344. doi: 10.1002/advs.201900344
- Lee A, Hudson A, Shiwarski D, et al. 3D bioprinting of collagen to rebuild components of the human heart. Science. 2019;365(6452):482-487. doi: 10.1126/science.aav9051
- Lammers A, Hsu H-H, Sundaram S, et al. Rapid tissue perfusion using sacrificial percolation of anisotropic networks. Matter. 2024;7(6):2184-2204. doi: 10.1016/j.matt.2024.04.001
- Ouyang L, Armstrong JP, Chen Q, Lin Y, Stevens MM. Void‐free 3D bioprinting for in situ endothelialization and microfluidic perfusion. Adv Funct Mater. 2020;30(1):1908349. doi: 10.1002/adfm.201908349
- Lawlor KT, Vanslambrouck JM, Higgins JW, et al. Cellular extrusion bioprinting improves kidney organoid reproducibility and conformation. Nat Mater. 2021;20(2):260-271. doi: 10.1038/s41563-020-00853-9
- Brassard JA, Nikolaev M, Hübscher T, Hofer M, Lutolf MP. Recapitulating macro-scale tissue self-organization through organoid bioprinting. Nat Mater. 2021;20(1):22-29. doi: 10.1038/s41563-020-00803-5
- Ayan B, Heo DN, Zhang Z, et al. Aspiration-assisted bioprinting for precise positioning of biologics. Sci Adv. 2020;6(10):eaaw5111. doi: 10.1126/sciadv.aaw5111
- Daly AC, Davidson MD, Burdick JA. 3D bioprinting of high cell-density heterogeneous tissue models through spheroid fusion within self-healing hydrogels. Nat Commun. 2021;12(1):753. doi: 10.1038/s41467-021-21029-2
- Egawa N, Kitaoka S, Tsukita K, et al. Drug screening for ALS using patient-specific induced pluripotent stem cells. Sci Transl Med. 2012;4(145):145ra104. doi: 10.1126/scitranslmed.3004052
- Griffith LG, Swartz MA. Capturing complex 3D tissue physiology in vitro. Nat Rev Mol Cell Biol. 2006;7(3): 211-224. doi: 10.1038/nrm1858
- Nelson CM, Bissell MJ. Of extracellular matrix, scaffolds, and signaling: tissue architecture regulates development, homeostasis, and cancer. Annu Rev Cell Dev Biol. 2006;22:287-309. doi: 10.1146/annurev.cellbio.22.010305.104315
- Nie J, Gao Q, Fu J, He Y. Grafting of 3D bioprinting to in vitro drug screening: a review. Adv Healthc Mater. 2020;9(7):1901773. doi: 10.1002/adhm.201901773
- Miller KL, Sit I, Xiang Y, et al. Evaluation of CuO nanoparticle toxicity on 3D bioprinted human iPSC-derived cardiac tissues. Bioprinting. 2023;32:e00284. doi: 10.1016/j.bprint.2023.e00284
- Zhao Y, Yao R, Ouyang L, et al. Three-dimensional printing of Hela cells for cervical tumor model in vitro. Biofabrication. 2014;6(3):035001. doi: 10.1088/1758-5082/6/3/035001
- Dai X, Ma C, Lan Q, Xu T. 3D bioprinted glioma stem cells for brain tumor model and applications of drug susceptibility. Biofabrication. 2016;8(4):045005. doi: 10.1088/1758-5090/8/4/045005
- Wang Y, Shi W, Kuss M, et al. 3D bioprinting of breast cancer models for drug resistance study. ACS Biomater Sci Eng. 2018;4(12):4401-4411. doi: 10.1021/acsbiomaterials.8b01277
- Wu M, Zhou H, Hu J, et al. Decellularized porcine kidney-incorporated hydrogels for cell-laden bioprinting of renal cell carcinoma model. Int J Bioprint. 2024; 10(2):1413. doi: 10.36922/ijb.1413
- Huang B, Wei X, Chen K, Wang L, Xu M. Bioprinting of hydrogel beads to engineer pancreatic tumor-stroma microtissues for drug screening. Int J Bioprint. 2023; 9(3):676. doi: 10.18063/ijb.676
- Gu Z, Xie M, Lv S, et al. Perfusable vessel-on-a-chip for antiangiogenic drug screening with coaxial bioprinting. Int J Bioprint. 2022;8(4):619. doi: 10.18063/ijb.v8i4.619
- Fang L, Liu Y, Qiu J, Wan W. Bioprinting and its use in tumor-on-a-chip technology for cancer drug screening: a review. Int J Bioprint. 2022;8(4):603. doi: 10.18063/ijb.v8i4.603
- Liu C, Chen Y, Chen H, Zhang P. Droplet-based bioprinting for fabrication of tumor spheroids. Int J Bioprint. 2024;10(1):1214. doi: 10.36922/ijb.1214
- Maizels L, Huber I, Arbel G, et al. Patient-specific drug screening using a human induced pluripotent stem cell model of catecholaminergic polymorphic ventricular tachycardia type 2. Circ Arrhythm Electrophysiol. 2017;10(6):e004725. doi: 10.1161/CIRCEP.116.004725
- Akbari M, Khademhosseini A. Tissue bioprinting for biology and medicine. Cell. 2022;185(15):2644-2648. doi: 10.1016/j.cell.2022.06.015
- Fonseca AC, Melchels FP, Ferreira MJ, et al. Emulating human tissues and organs: a bioprinting perspective toward personalized medicine. Chem Rev. 2020;120(19): 11093-11139. doi: 10.1021/acs.chemrev.0c00342
- Wang Z, Liang X, Wang G, Wang X, Chen Y. Emerging bioprinting for wound healing. Adv Mater. 2023:e2304738. doi: 10.1002/adma.202304738
- Liu H, He L, Kuzmanović M, et al. Advanced nanomaterials in medical 3D printing. Small Methods. 2024;8(3):e2301121. doi: 10.1002/smtd.202301121
- Eisenstein M. First 3D-printed pill. Nat Biotechnol. 2015;33:1014. doi: 10.1038/nbt1015-1014a
- Loh JM, Lim YJL, Tay JT, Cheng HM, Tey HL, Liang K. Design and fabrication of customizable microneedles enabled by 3D printing for biomedical applications. Bioactive Mater. 2024;32:222-241. doi: 10.1016/j.bioactmat.2023.09.022
- Li R, Liu X, Yuan X, et al. Fast customization of hollow microneedle patches for insulin delivery. Int J Bioprint. 2022;8(2):553. doi: 10.18063/ijb.v8i2.553
- Wang Z, Fu R, Han X, et al. Shrinking fabrication of a glucose‐responsive glucagon microneedle patch. Adv Sci. 2022;9(28):2203274. doi: 10.1002/advs.202203274
- Joo SH, Kim J, Hong J, Fakhraei Lahiji S, Kim YH. Dissolvable self‐locking microneedle patches integrated with immunomodulators for cancer immunotherapy. Adv Mater. 2023;35(10):2209966. doi: 10.1002/adma.202209966
- Li R, Zhang L, Jiang X, et al. 3D-printed microneedle arrays for drug delivery. J Control Release. 2022;350:933-948. doi: 10.1016/j.jconrel.2022.08.022
- Yadid M, Oved H, Silberman E, Dvir T. Bioengineering approaches to treat the failing heart: from cell biology to 3D printing. Nat Rev Cardiol. 2022;19(2):83-99. doi: 10.1038/s41569-021-00603-7
- Tao J, Liu H, Wu W, et al. 3D‐printed nerve conduits with live platelets for effective peripheral nerve repair. Adv Funct Mater. 2020;30(42):2004272. doi: 10.1002/adfm.202004272
- Choi S, Lee KY, Kim SL, et al. Fibre-infused gel scaffolds guide cardiomyocyte alignment in 3D-printed ventricles. Nat Mater. 2023;22(8):1039-1046. doi: 10.1038/s41563-023-01611-3
- Chen S, Luo J, Shen L, et al. 3D printing mini-capsule device for islet delivery to treat type 1 diabetes. ACS Appl Mater Interfaces. 2022;14(20):23139-23151. doi: 10.1021/acsami.2c02487
- Bertsch P, Diba M, Mooney DJ, Leeuwenburgh SC. Self-healing injectable hydrogels for tissue regeneration. Chem Rev. 2022;123(2):834-873. doi: 10.1021/acs.chemrev.2c00179
- Daly AC, Riley L, Segura T, Burdick JA. Hydrogel microparticles for biomedical applications. Nat Rev Mater. 2020;5(1):20-43. doi: 10.1038/s41578-019-0148-6.
- Liu X, Tao J, Liu J, et al. 3D printing enabled customization of functional microgels. ACS Appl Mater Interfaces. 2019;11(13):12209-12215. doi: 10.1021/acsami.8b18701.
- Muir VG, Qazi TH, Weintraub S, Torres Maldonado BO, Arratia PE, Burdick JA. Sticking together: injectable granular hydrogels with increased functionality via dynamic covalent inter‐particle crosslinking. Small. 2022;18(36):e2201115. doi: 10.1002/smll.202201115
- Tang M, Xie Q, Gimple RC, et al. Three-dimensional bioprinted glioblastoma microenvironments model cellular dependencies and immune interactions. Cell Res. 2020;30(10):833-853. doi: 10.1038/s41422-020-0338-1.
- Prendergast ME, Burdick JA. Recent advances in enabling technologies in 3D printing for precision medicine. Adv Mater. 2020;32(13):e1902516. doi: 10.1002/adma.201902516
- Wen X, Zhang B, Wang W, et al. 3D-printed silica with nanoscale resolution. Nat Mater. 2021;20(11):1506-1511. doi: 10.1038/s41563-021-01111-2
- You S, Xiang Y, Hwang HH, et al. High cell density and high-resolution 3D bioprinting for fabricating vascularized tissues. Sci Adv. 2023;9(8):eade7923. doi: 10.1126/sciadv.ade7923
- Guan J, You S, Xiang Y, et al. Compensating the cell-induced light scattering effect in light-based bioprinting using deep learning. Biofabrication. 2021;14(1):015011. doi: 10.1088/1758-5090/ac3b92.
- Bauer J, Crook C, Baldacchini T. A sinterless, low-temperature route to 3D print nanoscale optical-grade glass. Science. 2023;380(6648):960-966. doi: 10.1126/science.abq3037
- Zhang Y, Su Y, Zhao Y, Wang Z, Wang C. Two‐photon 3D printing in metal–organic framework single crystals. Small. 2022;18(18):e2200514. doi: 10.1002/smll.202200514
- Xu H-Q, Liu J-C, Zhang Z-Y, Xu C-X. A review on cell damage, viability, and functionality during 3D bioprinting. Mil Med Res. 2022;9(1):70. doi: 10.1186/s40779-022-00429-5
- Lipkowitz G, Samuelsen T, Hsiao K, et al. Injection continuous liquid interface production of 3D objects. Sci Adv. 2022;8(39):eabq3917. doi: 10.1126/sciadv.abq3917
- Regehly M, Garmshausen Y, Reuter M, et al. Xolography for linear volumetric 3D printing. Nature. 2020;588(7839): 620-624. doi: 10.1038/s41586-020-3029-7
- Stüwe L, Geiger M, Röllgen F, et al. Continuous volumetric 3D printing: xolography in flow. Adv Mater. 2024;36(4):e2306716. doi: 10.1002/adma.202306716
- Cheng J, Wang R, Sun Z, et al. Centrifugal multimaterial 3D printing of multifunctional heterogeneous objects. Nat Commun. 2022;13(1):7931. doi: 10.1038/s41467-022-35622-6
- Larson NM, Mueller J, Chortos A, Davidson ZS, Clarke DR, Lewis JA. Rotational multimaterial printing of filaments with subvoxel control. Nature. 2023;613(7945):682-688. doi: 10.1038/s41586-022-05490-7
- Buchner TJ, Rogler S, Weirich S, et al. Vision-controlled jetting for composite systems and robots. Nature. 2023;623(7987):522-530. doi: 10.1038/s41586-023-06684-3
- Wang Y, Cui H, Esworthy T, Mei D, Wang Y, Zhang LG. Emerging 4D printing strategies for next‐generation tissue regeneration and medical devices. Adv Mater. 2022;34(20):e2109198. doi: 10.1002/adma.202109198
- Bedell ML, Navara AM, Du Y, Zhang S, Mikos AG. Polymeric systems for bioprinting. Chem Rev. 2020;120(19): 10744-10792. doi: 10.1021/acs.chemrev.9b00834
- Hao XP, Xu Z, Li CY, Hong W, Zheng Q, Wu ZL. Kirigami‐design‐enabled hydrogel multimorphs with application as a multistate switch. Adv Mater. 2020;32(22):e2000781. doi: 10.1002/adma.202000781
- Cui H, Miao S, Esworthy T, et al. A novel near-infrared light responsive 4D printed nanoarchitecture with dynamically and remotely controllable transformation. Nano Res. 2019;12:1381-1388. doi: 10.1007/s12274-019-2340-9
- Miao S, Cui H, Esworthy T, et al. 4D self‐morphing culture substrate for modulating cell differentiation. Adv Sci. 2020;7(6):1902403. doi: 10.1002/advs.201902403
- Zhang F, Wang L, Zheng Z, Liu Y, Leng J. Magnetic Programming of 4D Printed Shape Memory Composite Structures. Elsevier. 2019;125:105571. doi: 10.1016/j.compositesa.2019.105571
- Bai Y, Zhang J, Wen D, et al. A reconfigurable, self-healing and near infrared light responsive thermoset shape memory polymer. Compos Sci Technol. 2020;187:107940. doi: 10.1016/j.compscitech.2019.107940
- Lee YB, Jeon O, Lee SJ, Ding A, Wells D, Alsberg E. Induction of four‐dimensional spatiotemporal geometric transformations in high cell density tissues via shape‐changing hydrogels. Adv Funct Mater. 2021;31(24):2010104. doi: 10.1002/adfm.202010104.
- Samandari M, Mostafavi A, Quint J, Memić A, Tamayol A. In situ bioprinting: intraoperative implementation of regenerative medicine. Trends Biotechnol. 2022;40(10):1229-1247. doi: 10.1016/j.tibtech.2022.03.009
- Duchi S, Onofrillo C, O’Connell CD, et al. Handheld co-axial bioprinting: application to in situ surgical cartilage repair. Sci Rep. 2017;7(1):5837. doi: 10.1038/s41598-017-05699-x
- Li W, Wang M, Mille LS, et al. A smartphone‐enabled portable digital light processing 3D printer. Adv Mater. 2021;33(35):e2102153. doi: 10.1002/adma.202102153
- Thai MT, Phan PT, Tran HA, et al. Advanced soft robotic system for in situ 3D bioprinting and endoscopic surgery. Adv Sci. 2023;10(12):2205656. doi: 10.1002/advs.202205656
- Chen Y, Zhang J, Liu X, et al. Noninvasive in vivo 3D bioprinting. Sci Adv. 2020;6(23):eaba7406. doi: 10.1126/sciadv.aba7406
- Urciuolo A, Poli I, Brandolino L, et al. Intravital three-dimensional bioprinting. Nat Biomed Eng. 2020;4(9):901-915. doi: 10.1038/s41551-020-0568-z
- Zhou C, Yang Y, Wang J, et al. Ferromagnetic soft catheter robots for minimally invasive bioprinting. Nat Commun. 2021;12(1):5072. doi: 10.1038/s41467-021-25386-w
- Kuang X, Rong Q, Belal S, et al. Self-enhancing sono-inks enable deep-penetration acoustic volumetric printing. Science. 2023;382(6675):1148-1155. doi: 10.1126/science.adi1563
- Post MJ, Levenberg S, Kaplan DL, et al. Scientific, sustainability and regulatory challenges of cultured meat. Nat Food. 2020;1(7):403-415. doi: 10.1038/s43016-020-0112-z
- Su L, Jing L, Zeng X, et al. 3D‐printed prolamin scaffolds for cell‐based meat culture. Adv Mater. 2023;35(2):2207397. doi: 10.1002/adma.202207397
- Ianovici I, Zagury Y, Redenski I, Lavon N, Levenberg S. 3D-printable plant protein-enriched scaffolds for cultivated meat development. Biomaterials. 2022;284:121487. doi: 10.1016/j.biomaterials.2022.121487
- David S, Ianovici I, Guterman Ram G, Shaulov Dvir Y, Lavon N, Levenberg S. Pea protein‐rich scaffolds support 3D bovine skeletal muscle formation for cultivated meat application. Adv Sustain Syst. 2024;8(6):2300499. doi: 10.1002/adsu.202300499
- Kang D-H, Louis F, Liu H, et al. Engineered whole cut meat-like tissue by the assembly of cell fibers using tendon-gel integrated bioprinting. Nat Commun. 2021;12(1):5059. doi: 10.1038/s41467-021-25236-9
- Xu E, Niu R, Lao J, et al. Tissue-like cultured fish fillets through a synthetic food pipeline. NPJ Sci Food. 2023;7(1):17. doi: 10.1038/s41538-023-00194-2
- Jeong D, Seo JW, Lee HG, Jung WK, Park YH, Bae H. Efficient myogenic/adipogenic transdifferentiation of bovine fibroblasts in a 3D bioprinting system for steak‐type cultured meat production. Adv Sci. 2022;9(31):2202877. doi: 10.1002/advs.202202877
- Ng WL, Tan JS. Application of machine learning in 3D bioprinting of cultivated meat. Int J AI Mater Design. 2024;1(1):2279. doi: 10.36922/ijamd.2279
- Ng WL, Goh GL, Goh GD, Sheuan TJJ, Yeong WY. Progress and opportunities for machine learning in materials and processes of additive manufacturing. Adv Mater. 2024:202310006. doi: 10.1002/adma.202310006
- Nikkhah A, Rohani A, Zarei M, et al. Toward sustainable culture media: Using artificial intelligence to optimize reduced-serum formulations for cultivated meat. Sci Total Environ. 2023;894:164988. doi: 10.1016/j.scitotenv.2023.164988
- Cosenza Z, Block DE, Baar K. Optimization of muscle cell culture media using nonlinear design of experiments. Biotechnol J. 2021;16(11):2100228. doi: 10.1002/biot.202100228
- Ji H, Pu D, Yan W, Zhang Q, Zuo M, Yuyu Z. Recent advances and application of machine learning in food flavor prediction and regulation. Trends Food Sci Technol. 2023;138:738-751. doi: 10.1016/j.tifs.2023.07.012
- Soni A, Dixit Y, Reis MM, Brightwell G. Hyperspectral imaging and machine learning in food microbiology: developments and challenges in detection of bacterial, fungal, and viral contaminants. Compr Rev Food Sci Food Saf. 2022;21(4):3717-3745. doi: 10.1111/1541-4337.12983
- Huang X, Ng WL, Yeong WY. Predicting the number of printed cells during inkjet-based bioprinting process based on droplet velocity profile using machine learning approaches. J Intell Manuf. 2023;35(5). doi: 10.1007/s10845-023-02167-4
- Wang J, Ding S, Da C, et al. Morphology-based prediction of proliferation and differentiation potencies of porcine muscle stem cells for cultured meat production. J Agric Food Chem. 2023;71(47):18613-18621. doi: 10.1021/acs.jafc.3c06919
- Axpe E, Oyen ML. Applications of alginate-based bioinks in 3D bioprinting. Int J Mol Sci. 2016;17(12):1976. doi: 10.3390/ijms17121976
- Jia J, Richards DJ, Pollard S, et al. Engineering alginate as bioink for bioprinting. Acta Biomater. 2014;10(10):4323-4331. doi: 10.1016/j.actbio.2014.06.034
- Gao Q, Kim B-S, Gao G. Advanced strategies for 3D bioprinting of tissue and organ analogs using alginate hydrogel bioinks. Marine Drugs. 2021;19(12):708. doi: 10.3390/md19120708
- López-Marcial GR, Zeng AY, Osuna C, Dennis J, García JM, O’Connell GD. Agarose-based hydrogels as suitable bioprinting materials for tissue engineering. ACS Biomater Sci Eng. 2018;4(10):3610-3616. doi: 10.1021/acsbiomaterials.8b00903
- Wang X, Wang Q, Xu C. Nanocellulose-based inks for 3d bioprinting: key aspects in research development and challenging perspectives in applications—a mini review. Bioengineering. 2020;7(2):40. doi: 10.3390/bioengineering7020040
- Athukoralalage SS, Balu R, Dutta NK, Roy Choudhury N. 3D bioprinted nanocellulose-based hydrogels for tissue engineering applications: a brief review. Polymers. 2019;11(5):898. doi: 10.3390/polym11050898
- Saji S, Hebden A, Goswami P, Du C. A brief review on the development of alginate extraction process and its sustainability. Sustainability. 2022;14(9):5181. doi: 10.3390/su14095181
- Chew KW, Juan JC, Phang SM, Ling TC, Show PL. An overview on the development of conventional and alternative extractive methods for the purification of agarose from seaweed. Sep Sci Technol. 2018;53(3):467-480. doi: 10.1080/01496395.2017.1394881
- Youssouf L, Lallemand L, Giraud P, et al. Ultrasound-assisted extraction and structural characterization by NMR of alginates and carrageenans from seaweeds. Carbohydr Polymers. 2017;166:55-63. doi: 10.1016/j.carbpol.2017.01.041
- Yuan Y, Macquarrie DJ. Microwave assisted step-by-step process for the production of fucoidan, alginate sodium, sugars and biochar from Ascophyllum nodosum through a biorefinery concept. Bioresourc Technol. 2015;198:819-827. doi: 10.1016/j.biortech.2015.09.090
- Torabi P, Hamdami N, Keramat J. Microwave-assisted extraction of sodium alginate from brown macroalgae Nizimuddinia zanardini, optimization and physicochemical properties. Sep Sci Technol. 2022;57(6):872-885. doi: 10.1080/01496395.2021.1954020
- Sugiono S, Masruri M, Estiasih T, Widjanarko SB. Optimization of extrusion-assisted extraction parameters and characterization of alginate from brown algae (Sargassum cristaefolium). J Food Sci Technol. 2019;56(8):3687-3696. doi: 10.1007/s13197-019-03829-z
- Din SS, Chew KW, Chang Y-K, Show PL, Phang SM, Juan JC. Extraction of agar from Eucheuma cottonii and Gelidium amansii seaweeds with sonication pretreatment using autoclaving method. J Oceanol Limnol. 2019;37(3):871-880. doi: 10.1007/s00343-019-8145-6
- Sharma M, Chaudhary JP, Mondal D, Meena R, Prasad K. A green and sustainable approach to utilize bio-ionic liquids for the selective precipitation of high purity agarose from an agarophyte extract. Green Chem. 2015;17(5):2867-2873. doi: 10.1039/C4GC02498B
- Rieland JM, Love BJ. Ionic liquids: a milestone on the pathway to greener recycling of cellulose from biomass. Resources Conserv Recycl. 2020;155:104678. doi: 10.1016/j.resconrec.2019.104678
- Chen H, Xiao Q, Weng H, Zhang Y, Yang Q, Xiao A. Extraction of sulfated agar from Gracilaria lemaneiformis using hydrogen peroxide-assisted enzymatic method. Carbohydr Polym. 2020;232:115790. doi: 10.1016/j.carbpol.2019.115790
- de Aguiar J, Bondancia TJ, Claro PIC, Mattoso LHC, Farinas CS, Marconcini JM. Enzymatic deconstruction of sugarcane bagasse and straw to obtain cellulose nanomaterials. ACS Sustain Chem Eng. 2020;8(5):2287-2299. doi: 10.1021/acssuschemeng.9b06806
- Morimura S, Nagata H, Uemura Y, Fahmi A, Shigematsu T, Kida K. Development of an effective process for utilization of collagen from livestock and fish waste. Process Biochem. 2002;37(12):1403-1412. doi: 10.1016/S0032-9592(02)00024-9
- Ahmed M, Verma AK, Patel R. Collagen extraction and recent biological activities of collagen peptides derived from sea-food waste: a review. Sustain Chem Pharm. 2020;18:100315. doi: 10.1016/j.scp.2020.100315
- Noorzai S, Verbeek CJR, Lay MC, Swan J. Collagen extraction from various waste bovine hide sources. Waste Biomass Valoriz. 2020;11:5687-5698. doi: 10.1007/s12649-019-00843-2
- Lee JM, Suen SKQ, Ng WL, Ma WC, Yeong WY. Bioprinting of collagen: considerations, potentials, and applications. Macromolecular Biosci. 2020;21(1):2000280. doi: 10.1002/mabi.202000280
- Taghizadeh M, Taghizadeh A, Yazdi MK, et al. Chitosan-based inks for 3D printing and bioprinting. Green Chem. 2022;24(1):62-101. doi: 10.1039/d1gc01799c
- Chawla S, Midha S, Sharma A, Ghosh S. Silk‐based bioinks for 3D bioprinting. Adv Healthc Mater. 2018;7(8): 1701204. doi: 10.1002/adhm.201701204
- Yu K-F, Lu T-Y, Li Y-CE, et al. Design and synthesis of stem cell-laden keratin/glycol chitosan methacrylate bioinks for 3D bioprinting. Biomacromolecules. 2022;23(7): 2814-2826. doi: 10.1021/acs.biomac.2c00191
- Brodin E, Boehmer M, Prentice A, et al. Extrusion 3D printing of keratin protein hydrogels free of exogenous chemical agents. Biomed Mater. 2022;17(5):055006. doi: 10.1088/1748-605X/ac7f15
- Navarro J, Clohessy RM, Holder RC, et al. In vivo evaluation of three-dimensional printed, keratin-based hydrogels in a porcine thermal burn model. Tissue Eng Part A. 2020; 26(5-6):265-278. doi: 10.1089/ten.tea.2019.0181
- Aramwit P, Siritientong T, Srichana T. Potential applications of silk sericin, a natural protein from textile industry by-products. Waste Manage Res. 2012;30(3):217-224. doi: 10.1177/0734242X11404733
- Reddy R, Jiang Q, Aramwit P, Reddy N. Litter to leaf: the unexplored potential of silk byproducts. Trends Biotechnol. 2021;39(7):706-718. doi: 10.1016/j.tibtech.2020.11.001
- Shavandi A, Silva TH, Bekhit AA, Bekhit AE-DA. Keratin: dissolution, extraction and biomedical application. Biomater Sci. 2017;5(9):1699-1735. doi: 10.1039/C7BM00411G
- Wang Z, Yang Y, Gao Y, Xu Z, Yang S, Jin M. Establishing a novel 3D printing bioinks system with recombinant human collagen. Int J Biol Macromol. 2022;211:400-409. doi: 10.1016/j.ijbiomac.2022.05.088
- Tytgat L, Dobos A, Markovic M, et al. High-resolution 3D bioprinting of photo-cross-linkable recombinant collagen to serve tissue engineering applications. Biomacromolecules. 2020;21(10):3997-4007. doi: 10.1021/acs.biomac.0c00386
- Sorkio A, Koch L, Koivusalo L, et al. Human stem cell based corneal tissue mimicking structures using laser-assisted 3D bioprinting and functional bioinks. Biomaterials. 2018;171:57-71. doi: 10.1016/j.biomaterials.2018.04.034
- Dai M, Belaïdi J-P, Fleury G, et al. Elastin-like polypeptide-based bioink: a promising alternative for 3D bioprinting. Biomacromolecules. 2021;22(12):4956-4966. doi: 10.1021/acs.biomac.1c00861
- Lechner A, Trossmann VT, Scheibel T. Impact of cell loading of recombinant spider silk based bioinks on gelation and printability. Macromol Biosci. 2022;22(3):2100390. doi: 10.1002/mabi.202100390
- Schacht K, Jüngst T, Schweinlin M, Ewald A, Groll J, Scheibel T. Biofabrication of cell‐loaded 3D spider silk constructs. Angew Chemie Int Ed. 2015;54(9):2816-2820. doi: 10.1002/anie.201409846
- Buechter DD, Paolella DN, Leslie BS, Brown MS, Mehos KA, Gruskin EA. Co-translational incorporation of trans-4- hydroxyproline into recombinant proteins in bacteria. J Biol Chem. 2003;278(1):645-650. doi: 10.1074/jbc.M209364200
- Toman PD, Chisholm G, McMullin H, et al. Production of recombinant human type I procollagen trimers using a four-gene expression system in the yeast Saccharomyces cerevisiae. J Biol Chem. 2000;275(30):23303-23309. doi: 10.1074/jbc.M002284200
- Vaughan PR, Galanis M, Richards KM, Tebb TA, Ramshaw JA, Werkmeister JA. Production of recombinant hydroxylated human type III collagen fragment in Saccharomyces cerevisiae. DNA Cell Biol. 1998;17(6): 511-518. doi: 10.1089/dna.1998.17.511
- Barnes LM, Bentley CM, Dickson AJ. Stability of protein production from recombinant mammalian cells. Biotechnol Bioeng. 2003;81(6):631-639. doi: 10.1002/bit.10517
- Demain AL, Vaishnav P. Production of recombinant proteins by microbes and higher organisms. Biotechnol Adv. 2009;27(3):297-306. doi: 10.1016/j.biotechadv.2009.01.008
- LeCun Y, Bengio Y, Hinton G. Deep learning. Nature. 2015;521(7553):436-444. doi: 10.1038/nature14539
- Ng WL, Chan A, Ong YS, Chua CK. Deep learning for fabrication and maturation of 3D bioprinted tissues and organs. Virtual Phys Prototyping. 2020;15(3):340-358. doi: 10.1080/17452759.2020.1771741
- Zhuang P, Ng WL, An J, Chua CK, Tan LP. Layer-by-layer ultraviolet assisted extrusion-based (UAE) bioprinting of hydrogel constructs with high aspect ratio for soft tissue engineering applications. PLoS One. 2019;14(6):e0216776. doi: 10.1371/journal.pone.0216776
- Ozbolat IT, Hospodiuk M. Current advances and future perspectives in extrusion-based bioprinting. Biomaterials. 2016;76:321-343. doi: 10.1016/j.biomaterials.2015.10.076
- Ng WL, Yeong WY, Naing MW. Potential of bioprinted films for skin tissue engineering. In: Proceedings of the 1st International Conference on Progress in Additive Manufacturing; 2014:441-446. doi: 10.3850/978-981-09-0446-3_065
- Ng WL, Yeong WY, Naing MW. Development of polyelectrolyte chitosan-gelatin hydrogels for skin bioprinting. Procedia CIRP. 2016;49:105-112. doi: 10.1016/j.procir.2015.09.002
- Suntornnond R, Ng WL, Huang X, Yeow ECH, Yeong WY. Improving printability of hydrogel-based bio-inks for thermal inkjet bioprinting applications via saponification and heat treatment process. J Mater Chem B. 2022;10(31): 5989-6000. doi: 10.1039/D2TB00442A
- Ng WL, Yeong WY, Naing MW. Microvalve bioprinting of cellular droplets with high resolution and consistency. Proc Int Conf Prog Additive Manuf. 2016; 2016:397-402. doi: 10.3850/2424-8967_V02-236
- Ng WL, Lee JM, Yeong WY, Win Naing M. Microvalve-based bioprinting – process, bio-inks and applications. Biomater Sci. 2017;5(4):632-647. doi: 10.1039/C6BM00861E
- Li W, Mille LS, Robledo JA, Uribe T, Huerta V, Zhang YS. Recent advances in formulating and processing biomaterial inks for vat polymerization‐based 3D printing. Adv Healthc Mater. 2020;9(15):2000156. doi: 10.1002/adhm.202000156
- Ng WL, Chua CK, Shen Y-F. Print me an organ! Why we are not there yet. Prog Polymer Sci. 2019;97:101145. doi: 10.1016/j.progpolymsci.2019.101145
- Levato R, Jungst T, Scheuring RG, Blunk T, Groll J, Malda J. From shape to function: the next step in bioprinting. Adv Mater. 2020;32(12):1906423. doi: 10.1002/adma.201906423
- Ng WL, Wang S, Yeong WY, Naing MW. Skin bioprinting: impending reality or fantasy? Trends Biotechnol. 2016;34 (9):689-699. doi: 10.1016/j.tibtech.2016.04.006
- Lee JM, Ng WL, Yeong WY. Resolution and shape in bioprinting: strategizing towards complex tissue and organ printing. Appl Phys Rev. 2019;6(1):011307. doi: 10.1063/1.5053909
- Ng WL, Lee JM, Zhou M, Yeong WY. Hydrogels for 3-D bioprinting-based tissue engineering. In: Narayan R, ed. Rapid Prototyping of Biomaterials. Elsevier; 2020: 183-204. doi: 10.1016/B978-0-08-102663-2.00008-3
- Ng WL, Tan ZQ, Yeong WY, Naing MW. Proof-of-concept: 3D bioprinting of pigmented human skin constructs. Biofabrication. 2018;10(2):025005. doi: 10.1088/1758-5090/aa9e1e
- Lu Y, Rai R, Nitin N. Image-based assessment and machine learning-enabled prediction of printability of polysaccharides-based food ink for 3D printing. Food Res Int. 2023;173:113384. doi: 10.1016/j.foodres.2023.113384
- Chen H, Liu Y, Balabani S, Hirayama R, Huang J. Machine learning in predicting printable biomaterial formulations for direct ink writing. Research. 2023;6:0197. doi: 10.34133/research.0197
- Han S, Kim CM, Jin S, Kim TY. Study of the process-induced cell damage in forced extrusion bioprinting. Biofabrication. 2021;13(3):035048. doi: 10.1088/1758-5090/ac0415
- Fischer L, Nosratlo M, Hast K, et al. Calcium supplementation of bioinks reduces shear stress-induced cell damage during bioprinting. Biofabrication. 2022;14(4):045005. doi: 10.1088/1758-5090/ac84af
- Ng WL, Shkolnikov V. Optimizing cell deposition for inkjet-based bioprinting. Int J Bioprint. 2024;10(2):2135. doi: 10.36922/ijb.2135
- Blaeser A, Duarte Campos DF, Puster U, Richtering W, Stevens MM, Fischer H. Controlling shear stress in 3D bioprinting is a key factor to balance printing resolution and stem cell integrity. Adv Healthc Mater. 2016; 5(3):326-333. doi: 10.1002/adhm.201500677
- Ng WL, Huang X, Shkolnikov V, Goh GL, Suntornnond R, Yeong WY. Controlling droplet impact velocity and droplet volume: key factors to achieving high cell viability in sub-nanoliter droplet-based bioprinting. Int J Bioprint. 2022;8(1):424. doi: 10.18063/ijb.v8i1.424
- Ng WL, Huang X, Shkolnikov V, Suntornnond R, Yeong WY. Polyvinylpyrrolidone-based bioink: influence of bioink properties on printing performance and cell proliferation during inkjet-based bioprinting. Bio-Design Manufacturing. 2023;6:676-690. doi: 10.1007/s42242-023-00245-3
- Ng WL, Yeong WY, Naing MW. Polyvinylpyrrolidone-based bio-ink improves cell viability and homogeneity during drop-on-demand printing. Materials. 2017;10(2):190. doi: 10.3390/ma10020190
- Ng WL, Lee JM, Zhou M, et al. Vat polymerization-based bioprinting–process, materials, applications and regulatory challenges. Biofabrication. 2020;12(2):022001. doi: 10.1088/1758-5090/ab6034
- Mohammadrezaei D, Podina L, De Silva J, Kohandel M. Cell viability prediction and optimization in extrusion-based bioprinting via neural network-based Bayesian optimization models. Biofabrication. 2024;16(2). doi: 10.1088/1758-5090/ad17cf
- Xu H, Liu Q, Casillas J, et al. Prediction of cell viability in dynamic optical projection stereolithography-based bioprinting using machine learning. J Intell Manuf. 2022;33(4):995-1005. doi: 10.1007/s10845-020-01708-5
- Ruberu K, Senadeera M, Rana S, et al. Coupling machine learning with 3D bioprinting to fast track optimisation of extrusion printing. Appl Mater Today. 2021;22:100914. doi: 10.1016/j.apmt.2020.100914
- Fu Z, Angeline V, Sun W. Evaluation of printing parameters on 3D extrusion printing of pluronic hydrogels and machine learning guided parameter recommendation. Int J Bioprint. 2021;7(4):434. doi: 10.18063/ijb.v7i4.434
- Oh D, Shirzad M, Kim MC, Chung E-J, Nam SY. Rheology-informed hierarchical machine learning model for the prediction of printing resolution in extrusion-based bioprinting. Int J Bioprinting. 2023;9(6):1280. doi: 10.36922/ijb.1280
- Hales S, Tokita E, Neupane R, et al. 3D printed nanomaterial-based electronic, biomedical, and bioelectronic devices. Nanotechnology. 2020;31(17):172001. doi: 10.1088/1361-6528/ab5f29
- Kong YL, Gupta MK, Johnson BN, McAlpine MC. 3D printed bionic nanodevices. Nano Today. 2016;11(3): 330-350. doi: 10.1016/j.nantod.2016.04.007
- Gao C, Li Y, Liu X, Huang J, Zhang Z. 3D bioprinted conductive spinal cord biomimetic scaffolds for promoting neuronal differentiation of neural stem cells and repairing of spinal cord injury. Chem Eng J. 2023;451:138788. doi: 10.1016/j.cej.2022.138788
- Mannoor MS, Jiang Z, James T, et al. 3D printed bionic ears. Nano Lett. 2013;13(6):2634-2639. doi: 10.1021/nl4007744
- Zips S, Grob L, Rinklin P, et al. Fully printed μ-needle electrode array from conductive polymer ink for bioelectronic applications. ACS Appl Mater Interfaces. 2019;11(36):32778-32786. doi: 10.1021/acsami.9b11774.
- Su C-K, Chen J-C. One-step three-dimensional printing of enzyme/substrate–incorporated devices for glucose testing. Anal Chim Acta. 2018;1036:133-140. doi: 10.1016/j.aca.2018.06.073
- Hussain A, Abbas N, Ali A. Inkjet printing: a viable technology for biosensor fabrication. Chemosensors. 2022;10(3):103. doi: 10.3390/chemosensors10030103
- Tomaskovic‐Crook E, Zhang P, Ahtiainen A, et al. Human neural tissues from neural stem cells using conductive biogel and printed polymer microelectrode arrays for 3D electrical stimulation. Adv Healthc Mater. 2019;8(15):1900425. doi: 10.1002/adhm.201900425
- Moya A, Ortega-Ribera M, Guimerà X, et al. Online oxygen monitoring using integrated inkjet-printed sensors in a liver-on-a-chip system. Lab Chip. 2018;18(14):2023-2035. doi: 10.1039/C8LC00456K
- Rothbauer M, Eilenberger C, Spitz S, et al. Recent advances in additive manufacturing and 3D bioprinting for organs-on-a-chip and microphysiological systems. Review. Front Bioeng Biotechnol. 2022;10. doi: 10.3389/fbioe.2022.837087
- Lind JU, Busbee TA, Valentine AD, et al. Instrumented cardiac microphysiological devices via multimaterial three-dimensional printing. Nat Mater. 2017;16(3):303-308. doi: 10.1038/nmat4782
- Liu Z, Zhang Y, Xu S, et al. A 3D printed smartphone optosensing platform for point-of-need food safety inspection. Anal Chim Acta. 2017;966:81-89. doi: 10.1016/j.aca.2017.02.022
- Dufil G, Bernacka-Wojcik I, Armada-Moreira A, Stavrinidou E. Plant bioelectronics and biohybrids: the growing contribution of organic electronic and carbon-based materials. Chem Rev. 2022;122(4):4847-4883. doi: 10.1021/acs.chemrev.1c00525
- Yamashita T, Yamashita Y, Takano M, Sato N, Nakano S, Yokoyama H. 3D printed lattice-structured metal electrodes for enhanced current production in bioelectrochemical systems. Environ Technol. 2023;44(21):3229-3235. doi: 10.1080/09593330.2022.2056083
- Baş F, Kaya MF. 3D printed anode electrodes for microbial electrolysis cells. Fuel. 2022;317(6):123560. doi: 10.1016/j.fuel.2022.123560
- Hoffmann M, Elwany A. In-space additive manufacturing: a review. J Manuf Sci Eng. 2023;145(2). doi: 10.1115/1.4055603
- Rezapour Sarabi M, Yetisen AK, Tasoglu S. Bioprinting in microgravity. ACS Biomater Sci Eng. 2023;9(6):3074-3083. doi: 10.1021/acsbiomaterials.3c00195
- Yang J-Q, Jiang N, Li Z-P, et al. The effects of microgravity on the digestive system and the new insights it brings to the life sciences. Life Sci Space Res. 2020;27:74-82. doi: 10.1016/j.lssr.2020.07.009
- Mao M, Meng Z, Huang X, et al. 3D printing in space: from mechanical structures to living tissues. Int J Extreme Manuf. 2024;6(2):023001. doi: 10.1088/2631-7990/ad23ef
- Parfenov VA, Khesuani YD, Petrov SV, et al. Magnetic levitational bioassembly of 3D tissue construct in space. Sci Adv. 2020;6(29):eaba4174. doi: 10.1126/sciadv.aba4174
- Tabury K, Rehnberg E, Baselet B, Baatout S, Moroni L. Bioprinting of cardiac tissue in space: where are we? Adv Healthc Mater. 2023;12(23): 2203338. doi: 10.1002/adhm.202203338
- Chang CC, Boland ED. 3D bioprinting aboard the international space station using the techshot biofabrication facility. In: Hessel V, Stoudemire J, Miyamoto H, Fisk I D, eds. In‐Space Manufacturing and Resources; 2022:303-317. doi: 10.1002/9783527830909.ch16
- Mo XW, Zhang YM, Wang ZX, et al. Satellite-based on-orbit printing of 3D tumor models. Adv Mater. 2023: e2309618. doi: 10.1002/adma.202309618
- Averesch NJH, Berliner AJ, Nangle SN, et al. Microbial biomanufacturing for space-exploration—what to take and when to make. Nat Commun. 2023;14(1):2311. doi: 10.1038/s41467-023-37910-1
- Duraj-Thatte AM, Manjula-Basavanna A, Rutledge J, et al. Programmable microbial ink for 3D printing of living materials produced from genetically engineered protein nanofibers. Nat Commun. 2021;12(1):6600. doi: 10.1038/s41467-021-26791-x
- Heveran CM, Williams SL, Qiu J, et al. Biomineralization and successive regeneration of engineered living building materials. Matter. 2020;2(2):481-494. doi: 10.1016/j.matt.2019.11.016
- Gantenbein S, Colucci E, Käch J, et al. Three-dimensional printing of mycelium hydrogels into living complex materials. Nat Mater. 2022;22(1):128-134. doi: 10.1038/s41563-022-01429-5