Effect of graphene and graphene oxide addition on crosslinking and mechanical properties of photocurable resins for stereolithography
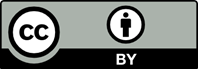
The mechanical properties of the resins used in stereolithography are often inadequate, prompting studies on their enhancement with nanofillers such as graphene-based nanomaterials (GBNs). GBNs hold promise for enhancing the mechanical performance of photocurable resins, yet their incorporation often leads to unexpected alterations that impact the final nanocomposite. The full spectrum of GBN effects on these resins remains incompletely understood, with many studies reporting suboptimal improvements. This study aims to elucidate the influence of graphene (G) and graphene oxide (GO) on the mechanical properties and polymer structure of an acrylic photocurable resin used in stereolithography. The novelty of this research lies in examining how GBNs affect the polymer structure during polymerization and the degree of crosslinking—parameters that have not been sufficiently explored—and correlating these effects with photopolymerization outcomes. Stereolithography is particularly valuable in biomedicine thanks to not only its exceptional precision in creating patient-specific models, functional parts, implant devices or scaffolds for tissue engineering, but also various other innovative uses across different industries. Through comprehensive tensile tests, dynamic mechanical thermal analysis, differential scanning calorimetry, Fourier-transformed infrared spectroscopy, and microscopy analyses, it was found that GO enhances tensile strength but reduces the crosslinking degree, thus hindering overall improvements. These findings highlight the critical roles of nanomaterial dispersion, matrix-polymer interaction, and reinforcement in affecting proper crosslinking. Future studies should investigate the impact of varying nanoparticle sizes on crosslinking to further validate these hypotheses.

- Pazhamannil RV, Govindan P. Current state and future scope of additive manufacturing technologies via vat photopolymerization. Mater Today Proc. 2021;43:130-136. doi: 10.1016/j.matpr.2020.11.225
- Alifui-Segbaya F. Biomedical photopolymers in 3D printing. RPJ. 2019;26:437-444. doi: 10.1108/RPJ-10-2018-0268
- Melchels FPW, Feijen J, Grijpma DW. A review on stereolithography and its applications in biomedical engineering. Biomaterials. 2010;31:6121-6130. doi: 10.1016/j.biomaterials.2010.04.050
- Al Rashid A, Ahmed W, Khalid MY, Koç M. Vat photopolymerization of polymers and polymer composites: processes and applications. Addit Manuf. 2021; 47:102279. doi: 10.1016/j.addma.2021.102279
- Shebeeb CM, Afif MB, Jacob L, Choi D, Butt H. Vat photopolymerisation 3D printing of graphene-based materials. Virt Phys Prototyping. 2013;18:e2276250. doi: 10.1080/17452759.2023.2276250
- Al Rashid A, Khan SA, Al-Ghamdi SG, Koç M. Additive manufacturing of polymer nanocomposites: needs and challenges in materials, processes, and applications. J Mater Res Technol. 2021;14:910-941. doi: 10.1016/j.jmrt.2021.07.016
- Alsaadi M, Hinchy EP, McCarthy CT, Moritz VF, Portela A, Devine DM. 3D printing of photocurable resin reinforced by functionalised graphene nanoplatelets. Mater Proc. 2023;14:20. doi: 10.3390/IOCN2023-14540
- Paz E, Forriol F, del Real JC, Dunne N. Graphene oxide versus graphene for optimisation of PMMA bone cement for orthopaedic applications. Mater Sci Eng C. 2017;77:1003-1011. doi: 10.1016/j.msec.2017.03.269
- He S, Petkovich ND, Liu K, Qian Y, Macosko CW, Stein A. Unsaturated polyester resin toughening with very low loadings of GO derivatives. Polymer. 2017;110:149-157. doi: 10.1016/j.polymer.2016.12.057
- Zhang G, Song D, Jiang J, et al. Electrically assisted continuous vat photopolymerization 3D printing for fabricating high-performance ordered graphene/polymer composites. Compos B Eng. 2023;250:110449. doi: 10.1016/j.compositesb.2022.110449
- Liang A, Jiang X, Hong X, Jiang Y, Shao Z, Zhu D. Recent developments concerning the dispersion methods and mechanisms of graphene. Coatings. 2018;8:33. doi: 10.3390/coatings8010033
- Markandan K, Lai CQ. Enhanced mechanical properties of 3D printed graphene-polymer composite lattices at very low graphene concentrations. Compos A: Appl Sci Manuf. 2020;129:105726. doi: 10.1016/j.compositesa.2019.105726
- de Armentia SL, Fernández-Villamarín S, Ballesteros Y, Del Real JC, Dunne N, Paz E. 3D printing of a graphene-modified photopolymer using stereolithography for biomedical applications: a study of the polymerization reaction. Int J Bioprint. 2022;8:503. doi: 10.18063/ijb.v8i1.503
- Holt BD, Wright ZM, Arnold AM, Sydlik SA. Graphene oxide as a scaffold for bone regeneration. WIREs Nanomed Nanobiotechnol. 2017;9:e1437. doi: 10.1002/wnan.1437
- Salgado H, Fialho J, Marques M, Vaz M, Figueiral MH, Mesquita P. Mechanical and surface properties of a 3D-printed dental resin reinforced with graphene. J Rpemd. 2023;64:12-19. doi: 10.24873/j.rpemd.2023.03.1050
- Hanon MM, Ghaly A, Zsidai L, Szakál Z, Szabó I, Kátai L. Investigations of the mechanical properties of DLP 3D printed graphene/resin composites. Acta Polytech Hung. 2021;18(8):143-161. doi: 10.12700/APH.18.8.2021.8.8
- Lopez De Armentia S, Abenojar J, Ballesteros Y, Del Real JC, Dunne N, Paz E. Polymerization kinetics of acrylic photopolymer loaded with graphene-based nanomaterials for additive manufacturing. Nanomaterials. 2022;12:4498. doi: 10.3390/nano12244498
- Mendes-Felipe C, Patrocinio D, Laza JM, Ruiz-Rubio L, Vilas-Vilela JL. Evaluation of postcuring process on the thermal and mechanical properties of the Clear02TM resin used in stereolithography. Polymer Test. 2018;72:115-121. doi: 10.1016/j.polymertesting.2018.10.018
- Cingesar IK, Marković M-P, Vrsaljko D. Effect of post-processing conditions on polyacrylate materials used in stereolithography. Addit Manuf. 2022;55:102813. doi: 10.1016/j.addma.2022.102813
- Markandan K, Seetoh IP, Lai CQ. Mechanical anisotropy of graphene nanocomposites induced by graphene alignment during stereolithography 3D printing. J Mater Res. 2021;36:4262-4274. doi: 10.1557/s43578-021-00400-5
- Lai CQ, Markandan K, Luo B, Lam YC, Chung WC, Chidambaram A. Viscoelastic and high strain rate response of anisotropic graphene-polymer nanocomposites fabricated with stereolithographic 3D printing. Addit Manuf. 2021;37:101721. doi: 10.1016/j.addma.2020.101721
- Jančovičová V, Mikula M, Havlínová B, Jakubíková Z. Influence of UV-curing conditions on polymerization kinetics and gloss of urethane acrylate coatings. Prog Organic Coat. 2013;76:432-438. doi: 10.1016/j.porgcoat.2012.10.010
- Arias-Ferreiro G, Ares-Pernas A, Lasagabáster-Latorre A, et al. Printability study of a conductive polyaniline/acrylic formulation for 3D printing. Polymers. 2021;13:2068. doi: 10.3390/polym13132068
- ISO 527-2:2012. Plastics – Determination of Tensile Properties – Part 2: Test Conditions for Moulding and Extrusion Plastics.
- Quantifying Polymer Crosslinking Density Using Rheology and DMA – TA Instruments. Available at: https://www. tainstruments.com/applications-notes/quantifying-polymer-crosslinking-density-using-rheology-and-dma/ (accessed March 26, 2024).
- Zheng J, Png ZM, Ng SH, et al. Vitrimers: Current research trends and their emerging applications. Mater Today. 2021;51:586-625. doi: 10.1016/j.mattod.2021.07.003
- Ryu SH, Sin JH, Shanmugharaj AM. Study on the effect of hexamethylene diamine functionalized graphene oxide on the curing kinetics of epoxy nanocomposites. Eur Polymer J. 2014;52:88-97. doi: 10.1016/j.eurpolymj.2013.12.014
- Berlanga Duarte ML, Reyna Medina LA, Torres Reyes P, Esparza González SC, Herrera González AM. Dental restorative composites containing methacrylic spiroorthocarbonate monomers as antishrinking matrixes. J Appl Polym Sci. 2019;136:47114. doi: 10.1002/app.47114
- Alifui-Segbaya F, Bowman J, White AR, George R, Fidan I. Characterization of the double bond conversion of acrylic resins for 3D printing of dental prostheses. Compend Contin Educ Dent. 2019;40:e7-e11.
- Ahmadi-Moghadam B, Sharafimasooleh M, Shadlou S, Taheri F. Effect of functionalization of graphene nanoplatelets on the mechanical response of graphene/epoxy composites. Mater Design (1980-2015). 2015;66:142-149. doi: 10.1016/j.matdes.2014.10.047
- Hawkins DA, Haque A. Fracture toughness of carbon-graphene/epoxy hybrid nanocomposites. Procedia Eng. 2014;90:176-181. doi: 10.1016/j.proeng.2014.11.833
- Guo W, Yang Y, Liu C, et al. 3D printed TPMS structural PLA/GO scaffold: process parameter optimization, porous structure, mechanical and biological properties. J Mech Behav Biomed Mater. 2023;142:105848. doi: 10.1016/j.jmbbm.2023.105848
- Sharma A, Pan X, Bjuggren JM, et al. Probing the relationship between molecular structures, thermal transitions, and morphology in polymer semiconductors using a woven glass-mesh-based DMTA technique. Chem Mater. 2019;31:6740-6749. doi: 10.1021/acs.chemmater.9b01213
- Jiménez-Suárez A, Moriche R, Prolongo SG, Sánchez M, Ureña A. GNPs reinforced epoxy nanocomposites used as thermal interface materials. JNanoR. 2016;38:18-25. doi: 10.4028/www.scientific.net/JNanoR.38.18
- Putz KW, Palmeri MJ, Cohn RB, Andrews R, Brinson LC. Effect of cross-link density on interphase creation in polymer nanocomposites. Macromolecules. 2008;41:6752-6756. doi: 10.1021/ma800830p
- Vilas JL, Laza JM, Garay MT, Rodríguez M, León LM. Unsaturated polyester resins cure: kinetic, rheologic, and mechanical dynamical analysis. II. The glass transition in the mechanical dynamical spectrum of polyester networks. J Polym Sci B: Polym Phys. 2001;39:146-152. doi: 10.1002/1099-0488(20010101)39:1<146::AID-POLB130>3.0.CO;2-A
- La LBT, Nguyen H, Tran LC, et al. Exfoliation and dispersion of graphene nanoplatelets for epoxy nanocomposites. Adv Nanocompos. 2024;1:39-51. doi: 10.1016/j.adna.2023.10.001
- Tang L-C, Wan Y-J, Yan D, et al. The effect of graphene dispersion on the mechanical properties of graphene/epoxy composites. Carbon. 2013;60:16-27. doi: 10.1016/j.carbon.2013.03.050
- Paz E, Ballesteros Y, Abenojar J, Dunne N, del Real JC. Advanced G-MPS-PMMA bone cements: influence of graphene silanisation on fatigue performance, thermal properties and biocompatibility. Nanomaterials. 2021;11:139. doi: 10.3390/nano11010139
- Hu K, Kulkarni DD, Choi I, Tsukruk VV. Graphene-polymer nanocomposites for structural and functional applications. Prog Polym Sci. 2014;39:1934-1972. doi: 10.1016/j.progpolymsci.2014.03.001
- Surnova A, Balkaev D, Musin D, Amirov R, Dimiev AM. Fully exfoliated graphene oxide accelerates epoxy resin curing, and results in dramatic improvement of the polymer mechanical properties. Compos B: Eng. 2019;162:685-691. doi: 10.1016/j.compositesb.2019.01.020
- Nielsen LE. Cross-linking–effect on physical properties of polymers. J Macromol Sci C. 1969;3:69-103. doi: 10.1080/15583726908545897
- Shokuhfar A, Arab B. The effect of cross linking density on the mechanical properties and structure of the epoxy polymers: molecular dynamics simulation. J Mol Model. 2013;19:3719-3731. doi: 10.1007/s00894-013-1906-9
- Gao C, Liu T, Shuai C, Peng S. Enhancement mechanisms of graphene in nano-58S bioactive glass scaffold: mechanical and biological performance. Sci Rep. 2014; 4:4712. doi: 10.1038/srep04712
- Sharif M, Pourabbas B, Sangermano M, et al. The effect of graphene oxide on UV curing kinetics and properties of SU8 nanocomposites. Polym Int. 2017;66: 405-417. doi: 10.1002/pi.5271
- Moriche R, Artigas J, Reigosa L, Sánchez M, Prolongo SG, Ureña A. Modifications induced in photocuring of Bis- GMA/TEGDMA by the addition of graphene nanoplatelets for 3D printable electrically conductive nanocomposites. Compos Sci Technol. 2019;184:107876. doi: 10.1016/j.compscitech.2019.107876
- Wan Y-J, Tang L-C, Yan D, et al. Improved dispersion and interface in the graphene/epoxy composites via a facile surfactant-assisted process. Compos Sci Technol. 2013;82:60-68. doi: 10.1016/j.compscitech.2013.04.009
- Borella PS, Alvares LAS, Ribeiro MTH, et al. Das neves, physical and mechanical properties of four 3D-printed resins at two different thick layers: an in vitro comparative study. Dental Mater. 2023;39:686-692. doi: 10.1016/j.dental.2023.06.002
- Uysal E, Çakir M, Ekici B. Graphene oxide/epoxy acrylate nanocomposite production via SLA and importance of graphene oxide surface modification for mechanical properties. Rapid Prototyping J. 2021;27:682-691. doi: 10.1108/RPJ-06-2020-0114
- Alexopoulos ND, Paragkamian Z, Poulin P, Kourkoulis SK. Fracture related mechanical properties of low and high graphene reinforcement of epoxy nanocomposites. Compos Sci Technol. 2017;150:194-204. doi: 10.1016/j.compscitech.2017.07.030